div class=”ChapterContextInformation”>
15. Neurophysiology of Cluster Headache and Other Trigeminal Autonomic Cephalalgias
Keywords
Cluster headacheTrigeminal autonomic cephalalgiasEEGEvoked potentialsReflexPolysomnographySleepTMSPain mechanismsAbbreviations
- BR
Blink reflex
- CH
Cluster headache
- CPT
Cold pressor test
- DBS
Deep brain stimulation
- EEG
Electroencephalography
- ERPs
Event-related potentials
- HCs
Healthy controls
- IDAP
Intensity dependence of cortical auditory evoked potentials
- LEPs
Laser evoked potentials
- LFPs
Local field potentials
- nBR
Nociceptive blink reflex
- non-REM
Non-rapid eye movement
- NWR
Nociceptive withdrawal reflex
- REM
Rapid eye movement
- SSEPs
Somatosensory evoked potentials
- SUNA
Short-lasting unilateral neuralgiform headache attacks with cranial autonomic symptoms
- SUNCT
Short-lasting unilateral neuralgiform headache attacks with conjunctival injection and tearing
- TACs
Trigeminal autonomic cephalalgias
- TMS
Transcranial magnetic stimulation
- TST
Temporal summation threshold
- VEP
Visual evoked potential
15.1 Introduction
The conditions collectively termed trigeminal autonomic cephalalgias (TACs) include cluster headache (CH), paroxysmal hemicrania, short-lasting unilateral neuralgiform headache attacks with conjunctival injection and tearing (SUNCT), short-lasting unilateral neuralgiform headache attacks with cranial autonomic symptoms (SUNA), and hemicrania continua. These syndromes differ in attack duration and frequency and present different responses to therapy [1].
Neurophysiological methods, despite having no role in the routine activity of headache clinics [2], are commonly employed as atraumatic and noninvasive means of assessing neural functional integrity of the various subcortical-cortical structures claimed to be involved in the pathophysiology as well as in the peculiar phenotypical presentation of TACs.
Cluster headache is the most extensively studied TAC. Researchers, using cortical evoked potentials, brainstem reflexes, and spinal withdrawal reflexes, have described several interesting brain response abnormalities in this disorder. The results suggest that neurophysiological patterns may fluctuate between active and remission periods and differ according to certain clinical features of the headache.
This chapter aims to provide a complete and systematic outline of the results provided by different neurophysiological techniques that have been used to study the pathophysiology of TACs.
15.2 Electroencephalography and Microelectrode Recording During Deep Brain Stimulation in Cluster Headache
Electroencephalography, being of no use in the diagnosis of primary headaches [2], , is not recommended in the routine clinical evaluation of patients affected by TACs.
In the research setting, Silvestri and colleagues [3] performed a polysomnographic study in a group of 13 untreated episodic CH patients, 3 of whom experienced attacks during the recording. However, these three patients did not show abnormalities on electroencephalography (EEG), which, on the contrary, revealed abnormalities in two female patients with a family or personal history of epilepsy or head injury, and in one patient, also female, without personal or family antecedents. In the latter patient, non-rapid eye movement (non-REM) sleep EEG examination showed diffuse generalized rapid (4–5 c/s) polyspike and wave complexes bilaterally, mainly over the anterior derivations [3]. Another study failed to detect specific EEG findings in children with CH [4].
A few studies have evaluated patients implanted with devices for deep brain stimulation (DBS) of the ipsilateral hypothalamus (this is a treatment option for chronic CH patients refractory to other treatments) [5]. Recording of local field potentials (LFPs) from microelectrodes revealed tonic firing patterns with discharge rates ranging from 13 to 35 Hz; LFPs were unresponsive to sensory, motor, autonomic and emotional stimuli [6, 7], except in one case, reported to be sensitive to tactile stimulation of the ophthalmic branch of the trigeminal nerve, contralateral to the recording side [8]. Other authors observed, in a single patient during a CH attack, a prominent peak of hypothalamic LFP spectra recorded from the DBS electrode, with the LFPs showing a power increase of around 20 Hz. This activity lasted only a few minutes and was not detected at cortical level. These results were felt to argue for a role of hypothalamic activation in the generation of CH [9, 10].
15.3 Polysomnography and Sleep Dysfunction in Cluster Headache
Cluster headache and sleep are interrelated. Up to 80% of CH patients are woken during the night because of an attack [11], especially within the first 2 h of sleep onset, mostly between 1 and 3 a.m. [11–13].
Few polysomnographic studies have been carried out in CH.
Using a controlled study design in a population of 40 CH patients (21 episodic studied during an active cluster period, and 19 chronic), Barloese [11] investigated the macrostructure of sleep as well as arousals, and the relationship between spontaneous CH attacks and sleep (considering both sleep in general and specific sleep phenomena). They found a longer time to onset of rapid eye movement (REM) sleep (REM latency) and a lower percentage of REM sleep in patients versus controls; comparison of patients who did not suffer attacks during recording with those who did report attacks (during both sleep and wakefulness) revealed that both groups had reduced REM sleep. Moreover, the authors reported decreased cortical activation, as reflected in a decreased number of EEG arousals per hour of sleep, in the patients versus the controls [11]. In one case, these alterations remitted after the cluster phase [14]. Vetrugno et al. [15] found reduced sleep efficiency, fragmented sleep, and increased periodic limb movements during sleep in three male chronic CH patients. All these sleep abnormalities improved 4 months after implantation of DBS of the posterior hypothalamus [15].
Although some authors have reported an association between nocturnal CH attacks and REM sleep periods [16–18], others have not confirmed this [11]. This aspect is thus still debated. In a study involving a large sample of patients, no difference in the macrostructural composition of sleep was found between patients and controls, apart from a lower REM density in the former [11]. A relationship between REM-related attacks and sleep apneic events has been observed in both episodic and chronic CH by some authors [19–22], but not others [23, 24].
15.4 Evoked Potentials
Innocuous cortical evoked potential studies performed in cluster headache
Authors | Sensory modality | Stimulation parameters | Diagnosis | Results |
---|---|---|---|---|
Polich et al. [25] | VEPs | Full-field checkerboard, 1.9 Hz reversal rate, 0.25 and 1 degrees of visual angle | 10 male CH, 10 HCs | No differences between groups |
Boiardi et al. [26] | VEPs | Full-field checkerboard, 1.6 Hz reversal rate, 55 min of arc | 20 (18 males) CH, 20 HCs | P100 amplitudes were significantly lower than in controls, on the pain side only |
Boiardi et al. [27] | VEPs and BAEPs | VEP: full-field checkerboard, 1.6 Hz reversal rate, 55 min of arc; BAEPs: 11 Hz, 70 dB | 14 male CH, 14 HCs | VEPs: P100 amplitudes were significantly lower than in controls, on the pain side only; BAEPs: I–V latencies were increased on the pain side |
Bussone et al. [29] | BAEPs | Repetition rate of 11 Hz, 70 dB | 16 (12 episodic and 4 chronic) CH, 16 HCs | I–V latencies were increased in CH patients both during and outside an attack, and normalized during lithium therapy |
Afra et al. [30] | IDAP | Repetition rate of 0.55 Hz at four intensities (40, 50, 60 and 70 dB) | 15 (11 males) CH [during the bout, outside an attack], 13 HCs | ASF slopes of auditory evoked potentials were steep in CH patients compared with HCs, both during and outside a bout |
Firenze et al. [31] | SSEPs | Median nerve stimulation contralateral to the headache side, 0.2 stimulus duration, twitching threshold, repetition rate of 2 Hz | 10 male CH [during the bout, outside an attack, and again during an attack induced by intravenous administration of histamine], 20 HCs | During a bout, outside an attack, SSEP amplitudes were found to be like those of HCs, while they were reduced during an attacks induced by intravenous administration of histamine |
Cosentino et al. [32] | TMS | Paired-pulse focal TMS over the hand motor cortex (120% of RMT) of both hemispheres. Measurements: ICF and SICI, CSP, input-output curves (I-O curves) | 25 episodic CH patients (21 males) [13 outside bout, 12 during a bout], 13 HCs | RMT and CSP values did not differ between groups. Both inside and outside bouts, ICF was more pronounced in the hemisphere ipsilateral to the headache side with respect to the contralateral one, and also with respect to values recorded from both hemispheres in HCs. Only in patients evaluated during a bout were significantly higher SICI values were found in the motor cortex ipsilateral to the headache side compared to the contralateral one. Compared with what was found in HCs, a steeper slope was recorded from both hemispheres of CH patients evaluated outside a bout, and from the motor cortex contralateral to the headache side in patients during a bout |
15.4.1 Visual Evoked Potentials
An early study by Polich et al. [25] failed to find any significant differences in visual evoked potential (VEP) latencies and amplitudes between a group of ten male CH patients and matched controls. However, when VEP responses were assessed over both occipital regions (O1 and O2, 10–20 EEG system), P100 amplitudes were found to be significantly lower in patients than in controls, on the pain side only, during a pain-free period, not due to pupillary asymmetry [26, 27]. This finding was interpreted as reflecting cerebral neurotransmitter dysfunction in CH. Further investigations are needed to elucidate whether it is related to a possible abnormal asymmetrical activation of the posterior hypothalamus to thalamus pathway [28].
15.4.2 Auditory Evoked Potentials
Measurement of brainstem auditory evoked potentials is a useful electrophysiological method for studying brainstem functional integrity.
A larger asymmetry of the I–V interpeak latency, increased on the symptomatic side [27], was detected in both episodic and chronic CH with respect to healthy controls (HCs), irrespective of whether patients were recorded during or outside an attack [29]. Interestingly, this neurophysiological pattern normalized during prophylactic intervention with lithium [29].
Afra and co-workers [30] recorded intensity dependence of cortical auditory evoked potentials (IDAP) in 15 episodic CH patients during both the active and the remission phase. They found steeper amplitude-stimulus intensity function slopes in CH patients than in controls, in both phases, and this difference was not related to patients’ clinical features. Since IDAP is a surrogate marker of central nervous system serotonin transmission, the authors argued that their results reflect diminished serotonergic activity in raphe-hypothalamic serotonergic pathways in CH patients.
15.4.3 Somatosensory Evoked Potentials
Only one study involving the use of somatosensory evoked potentials (SSEPs) has been performed in CH (a group of ten patients). SSEPs were recorded, after median nerve stimulation at the wrist, during an active period but outside an attack, and then during an attack induced by intravenous administration of histamine (40 μg) [31]. In the first condition (during a bout but outside an attack), the patients showed normal SSEP patterns; however, during a severe histamine-induced attack, the amplitudes of the N20-P25 complex were significantly decreased in comparison with outside the attack, and in comparison with values recorded in HCs. This histamine-related effect on SSEPs seems to be specific to CH, since it was not found in other primary headache disorders, such as migraine and tension-type headache [31].
15.4.4 Motor Evoked Potentials
Transcranial magnetic stimulation (TMS) is a noninvasive method used to study the excitability of the underlying cortical area.
To date, only one TMS study has been performed in CH [32]: in this study, episodic CH patients, recorded in active and remission periods, showed normal thresholds for the elicitation of motor evoked potentials and normal duration of the cortical silent period. Using paired-pulse TMS, intracortical facilitation, recorded both in active and remission phases, was found to be increased in the hemisphere ipsilateral to the headache side versus the contralateral one, and compared with values recorded from both hemispheres in healthy volunteers. Only patients evaluated during the active phase showed significantly lower short intracortical inhibition in the motor cortex ipsilateral to the headache side compared with the contralateral one.
15.4.5 Event-Related Potentials and P300
Few studies have investigated cognitive event-related potentials (ERPs) in CH. Evers et al. [33, 34], using a visual oddball paradigm, evaluated the P300 component in CH patients and found, in active (but not remission) periods, a delayed latency as compared with HCs, but normal amplitude, reaction time, and cognitive habituation. Furthermore, P300 latency, amplitude, and reaction time were found to be significantly increased in chronic CH during the cluster period as compared with remission and with all other conditions [34]. The same authors also studied a group of chronic paroxysmal hemicrania patients during indomethacin treatment and detected normal ERP parameters [33, 34]. Prophylactic drugs normalized the ERP latencies in episodic, but not in chronic, CH. No significant correlations were found between ERP data and disease duration, attack duration and daily frequency of attacks in the different groups of patients [33, 34]. In one visual ERP study, P300 amplitude was decreased in CH patients in comparison with HCs, irrespective of the headache side [35].
Cognitive event-related potentials and noxious-related pain-related cortical evoked potential studies performed in cluster headache
Authors | Sensory modality | Stimulation parameters | Diagnosis | Results |
---|---|---|---|---|
Evers et al. [33] | ERPs (P300 component) | Visual oddball paradigm | 37 (32 males) CH [11 during remission, 26 during active phase], 8 CPH, 30 HCs | Delayed P300 latency with normal habituation in CH patients during active phase. CPH patients showed normal ERP parameters |
Evers et al. [34] | ERPs (P300 component) | Visual oddball paradigm | 61 (32 males) CH [50 episodic during active phase, 11 chronic], 12 CPH, 20 HCs | Delayed P300 latency with normal habituation more marked in chronic CH patients than in episodic CH patients during active phase. CPH patients showed normal ERP parameters |
Casale et al. [36] | ERPs (P300 component) | Auditory paradigm | 27 (21 males) CH, 25 HCs | Significantly smaller P200 amplitude in CH patients than in controls. No significant differences in P300 or N100 amplitudes were detected, or increased latencies for any of the ERP components considered |
Wang et al. 2014 [35] | ERPs (P300 component) | Visual oddball paradigm | 17 (15 males) CH, 15 HCs | P300 amplitude was decreased in CH patients compared with HCs, without side-to-side differences. No differences in P300 latency or in reaction times were detected |
van Vliet et al. [37] | TSEPs | Electrical stimuli were delivered to both sides on the corner of the mouth in all subjects at three times the sensory threshold | 28 male episodic CH patients during active phase [repeated in 22 also during remission], 22 HCs | TSEP N2 component latency was more delayed during the active phase on the affected side versus the non-affected side, compared with HCs and recordings outside the active phase. N1 latency was significantly delayed on the affected (during the active phase) and unaffected side (in remission phase only) compared with HCs |
Ellrich et al. [39] | LEPs | YAG laser stimulation of the cutaneous innervation territory of the supraorbital nerve | 25 (21 males) CH patients (16 episodic [7 during and 10 outside the active phase], 9 chronic), 10 HCs | In chronic CH patients on the headache side, the LEP N1c component occurred later, while the P2 amplitude was smaller than on the non-headache side. In episodic CH patients during the active phase, P2 latency was shorter on the headache side. In episodic CH outside the active phase the N2-P2 ratio was lower on the headache side |
Holle et al. [38] | PREPs | The PREP was recorded using two planar concentric surface electrodes placed above the entry zone of the supraorbital nerve | 66 (49 males) CH patients (46 episodic [7 during and 28 outside the active phase], 20 chronic), 30 HCs | In chronic CH patients, the authors found a decreased N2 latency ratio ([latency headache side/latency non-headache side] × 100) compared with HCs, which was not detected in episodic CH either during or outside the active phase |
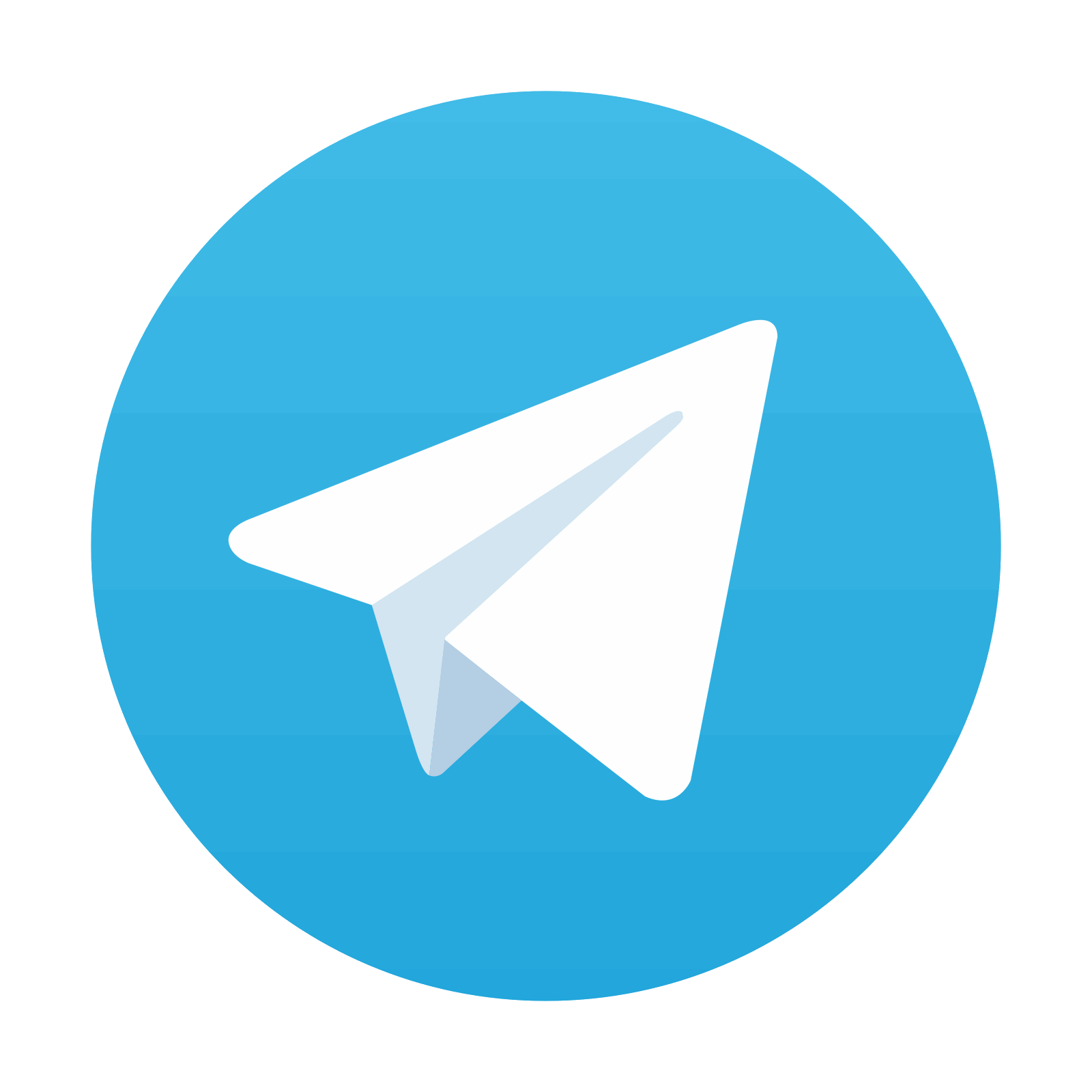
Stay updated, free articles. Join our Telegram channel

Full access? Get Clinical Tree
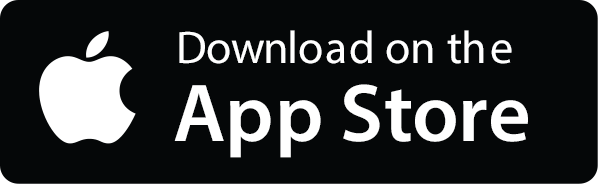
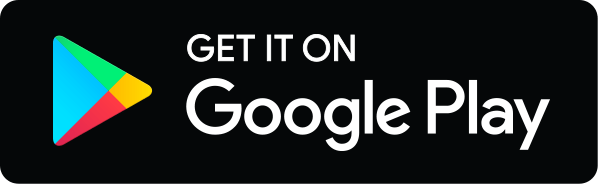