AED
Phenytoin
Carbamazepine
Oxcarbazepine
MHD
Phenobarbital
Valproate
Ethosuximide
Protein bindinga
High
Intermediate
Low
Low
High
Low
Half-lifeb
Intermediate (can become long in toxicity)
Intermediate
Short
Long
Intermediate
Long
Metabolism
Extensive,
Nonlinear
Extensive
Extensive
>70%
Extensive
Extensive
Enzyme Induction
+++
+++
autoinduction
+
(CYP 2C19)
+++
–
–
Enzyme Inhibition
–
–
+
(CYP 3A4)
–
+++
–
Phenobarbital exerts its action by binding to the GABA-A receptor and enhancing chloride currents by prolonging the opening of the chloride channel. It may also have other actions including blocking high-voltage-activated calcium channels and AMPA subtype glutamate receptors.
Phenobarbital is available as oral preparations as well as parenteral solution. Its oral availability is greater than 90%. Its protein binding is about 45%. Its volume of distribution is approximately 0.6 L/kg. Phenobarbital is mostly metabolized in the liver but 20–25% is eliminated unchanged in the urine. The half-life in adults is 80–100 h. The half-life is longer in newborns and shorter in young children. Phenobarbital is a potent inducer of P4 50 enzymes. It does accelerate the metabolism and reduces the levels of anti-epileptic drugs processed by this enzyme system. For example, phenobarbital reduces serum concentrations of valproate, ethosuximide, lamotrigine, and others. It reduces the serum concentration of carbamazepine but may increase the carbamazepine epoxide to carbamazepine ratio. It reduces the efficacy of warfarin, steroids, and the oral contraceptive.
Phenobarbital has a variable effect on phenytoin concentration; while it may induce phenytoin metabolism, it may also compete with phenytoin for metabolic enzymes CYP 2C9 and 2C19. Phenobarbital serum concentration may be increased by the inhibitors valproate and felbamate.
The main adverse effects of phenobarbital are sedation, mood changes (particularly depression), hyperactivity and irritability in children, and decreased memory and concentration. It also has long-term adverse effects. Long-term use of phenobarbital is associated with decreased bone density and some connective tissue disorders, particularly Dupuytren’s contractures, plantar fibromatosis, and frozen shoulder. Phenobarbital is associated with increased risk of cardiac malformations in the exposed fetus and reduced cognitive abilities in the exposed male offspring. It is assigned to pregnancy category D.
Phenobarbital is effective against partial onset (focal) seizures, generalized tonic–clonic seizures, and other generalized onset seizures except for absence. The recommended therapeutic concentration is 50 and 2 14 mg/L. Phenobarbital is not a drug of first choice in developed countries, because of its adverse effects, namely sedation, and because of its enzyme-inducing properties. However, it is inexpensive and widely available, and may be the only affordable antiepileptic drug in much of the developing world.
Primidone
Primidone is converted to phenobarbital and phenylethylmalonamide (PEMA), which is also an active metabolite. Unlike phenobarbital, primidone does not have a direct effect on GABA receptors. Primidone and phenobarbital may act synergistically to reduce sustained high-frequency repetitive firing, at clinically relevant concentrations. This is an effect on the sodium channel that neither drug has when used alone. As mentioned earlier, phenobarbital acts at the GABA-A receptor to prolong the opening of the chloride channel. The mechanism of action of PEMA is unknown, and its anti-seizure activity is modest. Primidone is available only as an oral preparation. It is poorly soluble, precluding an IV preparation.
Primidone oral bioavailability is fairly complete. Its volume of distribution is ~0.7 L/kg. Its protein binding is low, less than 10% for primidone and for PEMA.
Primidone is metabolized in the liver. PEMA is the first detected metabolite. When used in monotherapy, about 25% of oral primidone is converted to phenobarbital. After one dose, approximately 64% of primidone is excreted unchanged in the urine. In the presence of enzyme induction, only about 40% is excreted unchanged. In monotherapy, primidone half-life is 10–15 h; it is shorter (6.5–8.3 h) in the presence of enzyme inducers.
In the presence of inducers, particularly phenytoin, the ratio of primidone-to-phenobarbital is reduced due to the acceleration of primidone-to-phenobarbital conversion. Primidone and phenobarbital are potent enzyme inducers, decreasing the efficacy of drugs metabolized by the p450 enzymes system. Since phenobarbital will be present when primidone is used, all phenobarbital interactions are also present by necessity.
Primidone has acute toxic reactions that are different from phenobarbital. It can produce transient drowsiness, dizziness, ataxia, nausea, and vomiting that can be debilitating. These reactions are present even before phenobarbital has appeared as a metabolite. Therefore, a slow titration of primidone is necessary. Tolerance to these acute adverse experiences develops rapidly within hours to days. Otherwise, primidone has similar adverse experiences to phenobarbital, including adverse experiences from long-term use.
Primidone is effective against the same seizure types as phenobarbital. The recommended primidone therapeutic plasma concentration is 5–12 mg/L. A phenobarbital level should also be monitored. Since about 25% of oral primidone is converted to phenobarbital, the dose of primidone required for a certain level is about 4–5 times the dose of phenobarbital required for that same level. Primidone was found to have equal efficacy but lower tolerability in comparison with phenobarbital, phenytoin, and carbamazepine.
Phenytoin
Phenytoin has been used since 1938 when Houston and Merritt discovered its efficacy in the maximum electroshock animal model. Phenytoin acts by binding to active state of the sodium channel and reducing high-frequency firing (as might occur during a seizure), while allowing normal action potentials to occur.
Phenytoin is available as oral preparations and parenteral solution. There is also a phenytoin prodrug for parenteral administration, fosphenytoin.
Phenytoin absorption is variable. The rate and extent of absorption may also differ among formulations and is affected by a variety of factors including age and food. While oral bioavailability can be greater than 90% in adults, it is decreased in neonates and is also decreased in the presence of nasogastric feedings, calcium, and antacids. There is limited absorption in the stomach. Absorption is primarily in the duodenum where the higher pH increases the phenytoin solubility. The time to maximal concentration is shorter with immediate release preparations and longer with extended-release formulations. The volume of distribution is approximately 0.75 L/Kg. Protein binding is approximately 90%.
The major pathway of elimination of phenytoin is hydroxylation, mediated mainly by the cytochrome p450 enzymes CYP 2C9 and to a lesser extent CYP 2C19. Phenytoin follows nonlinear kinetics. Small changes in CYP 2C9 activity may have clinically significant effects. Some CYP 2C9 alleles are associated with reduced clearance of phenytoin. The importance of CYP 2C19 increases with higher levels. Some inhibitors such as ticlopidine and isoniazid may lead to phenytoin accumulation. Similarly, some alleles are associated with decreased activity leading to accumulation.
The phenytoin half-life is dependent on the serum concentration. The initial half-life is approximately 22 h (with the range of 8–60 h). The half-life increases as the serum concentration increases within and above the recommended therapeutic range (10–20 mg/L).
The mechanism of the nonlinear elimination kinetics is that enzymes responsible for most of phenytoin elimination are partially saturated with concentrations within the recommended range (with individual variation as to the concentration at which this phenomenon first appears). These enzymes are not able to increase their activity in proportion to phenytoin concentration, as the concentration increases in the recommended therapeutic range. Therefore, steady-state phenytoin level increases disproportionately as the maintenance dose is increased within and above the recommended therapeutic range. Below are two examples of the consequences of phenytoin nonlinear kinetics.
Example 1: A daily dose of 300 mg per day results in a concentration of 9 mg/L, with some residual seizures. Increasing the dose to 400 mg per day, a 30% increase in dose would have been expected to increase the steady-state concentration by 30%, to 12 mg/L, if phenytoin were to follow linear elimination kinetics. However, with its nonlinear kinetics, the concentration increases disproportionately, by more than 300%, to 31 mg/L, with associated toxicity. Therefore, when increasing phenytoin dose within the therapeutic range, small increments should be used (e.g., 30–60 mg).
Example 2: A patient presents with phenytoin toxicity and a serum concentration of 40 mg/L. The half-life was previously estimated at 24 h when the serum concentration was 13 mg/L. However, after phenytoin was stopped, it took 3 days for the serum concentration to drop below 20 mg/L. The reason for this is that the half-life was markedly prolonged in the presence of toxicity.
Phenytoin is affected by drugs that decrease absorption (e.g., nasogastric tube feedings) drugs that compete for protein binding (such as valproate) and by enzyme inducers or inhibitors. Drugs that cause phenytoin accumulation include amiodarone, azoles, fluoxetine/fluvoxamine, and isoniazid. Phenytoin is a potent enzyme inducer that reduces the efficacy of other drugs metabolized by the p450 enzyme system, including a number of other antiepileptic drugs.
Phenytoin protein binding plays an important role in some phenytoin interactions. The protein-free phenytoin level is responsible for its therapeutic effect and for its toxicity. The free fraction increases in the presence of low-protein state, renal failure, hepatic failure, old age, or with co-administration of valproate. Therapeutic decisions are usually made based on the total phenytoin level, assuming that 10% is free. However, a free level should be obtained in clinical situations where an increase in the free fraction is expected.
As an example of the potential consequences of altered protein binding of phenytoin, a patient with epilepsy and renal impairment may be having uncontrolled seizures with a total phenytoin concentration of 15 mg/L. The decision has to be made whether the dose should be increased to improve seizure control or should be decreased because of toxicity causing a paradoxical increase in seizure frequency. The protein-free concentration turns out to be 4.5 mg/L, equivalent to a total phenytoin concentration of 45 mg/L under the condition of normal protein binding. In this case, holding phenytoin was the correct approach. While renal and hepatic failures are frequently associated with the decreased albumin concentration, the reduction in protein binding may also occur as a result of small molecules that compete for protein binding.
Valproate competes with phenytoin for protein binding. In monotherapy, each of phenytoin and valproate are approximately 90% protein-bound, and the free phenytoin level is about 10% of the total level. With concomitant use, each 1 mg/L of valproate increases the free fraction of phenytoin by approximately 0.1–0.2%. At a valproate level of 100 mg/L, the free phenytoin fraction may increase to 20–30%. A phenytoin level of 15 mg/L may actually be toxic with a free level of 3–4.5 mg/L, equivalent to a total level of 30–45 mg/L in the presence of normal protein binding.
When a low-protein state is present as the only factor affecting protein binding, the total phenytoin level can be corrected using the following formula: Cn = Co/[(0.02 × albumin) + 0.1], where Cn is the normal total level and Co is the observed total level.
Phenytoin concentration-dependent adverse effects include nystagmus, ataxia, incoordination, diplopia, dysarthria, and drowsiness. In addition, exacerbation of seizures may occur with concentrations above 30 mg/L. Some individuals may experience prominent adverse effects within the recommended therapeutic range, including cognitive adverse effects.
Idiosyncratic reactions may be related to the formation of an arene oxide, the active metabolite that forms due to inadequate epoxide hydrolase activity. Allergic rash occurs in up to 8.5% of patients. Serious severe rash such as Stevens–Johnson syndrome or toxic epidermal necrolysis are much less common. A hypersensitivity syndrome may occur rarely, with rash, fever, lymphadenopathy, eosinophilia, elevated liver enzymes, and renal failure.
Phenytoin also has long-term adverse effects including gingival hyperplasia, hirsutism, acne, cerebellar atrophy (which may also occur after acute intoxication), reduced bone density, reduced folate levels, anemia, and macrocytosis. Phenytoin also has potential teratogenicity and is classified with pregnancy category D.
The IV preparation is associated with local reactions such as pain and burning at the infusion site, phlebitis, cellulitis, or necrosis from extravasation, and the purple glove syndrome with discoloration then petechial rash. Cardiovascular adverse experiences include hypotension, conduction abnormality, and arrhythmia. They are related in part to the vehicle, propylene glycol. They can be avoided with the slowing of the infusion rate, which should not exceed 50 mg/m.
Phenytoin is effective against partial onset (focal seizures) and generalized tonic–clonic seizures. Efficacy against tonic and atonic seizures is less well established. Phenytoin is not effective against generalized myoclonic or generalized absence seizures and may even exacerbate these seizures.
Phenytoin can be loaded orally (18 mg/kg divided into 3 doses given 2–3 h apart). IV loading dose for status epilepticus is 18–20 mg/kg. Phenytoin should be evaluated in normal saline, not dextrose 5% in water. It should be administered into a large vein with a maximum rate not exceeding 50 mg/min.
Intramuscular injection is not recommended due to slow and erratic absorption as well as crystallization at the injection site causing pain and a sterile abscess.
Fosphenytoin
Fosphenytoin is a water-soluble phenytoin prodrug. It can be given intravenously or intramuscularly. It is rapidly and completely converted to phenytoin by the cleavage of the phosphate group by nonspecific phosphatases. Its conversion half-life is 8–18 min, and the conversion is completed in a little more than 1 h. It is highly bound to serum albumin (95–99%). It displaces phenytoin from protein-binding sites after IV administration, increasing unbound phenytoin concentrations as a function of fosphenytoin concentration. It is indicated for a replacement of oral fosphenytoin or for intravenous or intramuscular loading. It is marketed in phenytoin equivalents, so the loading dose is the same as phenytoin. The maximum rate of intravenous infusion is much higher, at 150 mg/min, in view of the absence of propylene glycol. A therapeutic phenytoin level is usually reached within 10 min after IV loading and within 30 min after intramuscular administration.
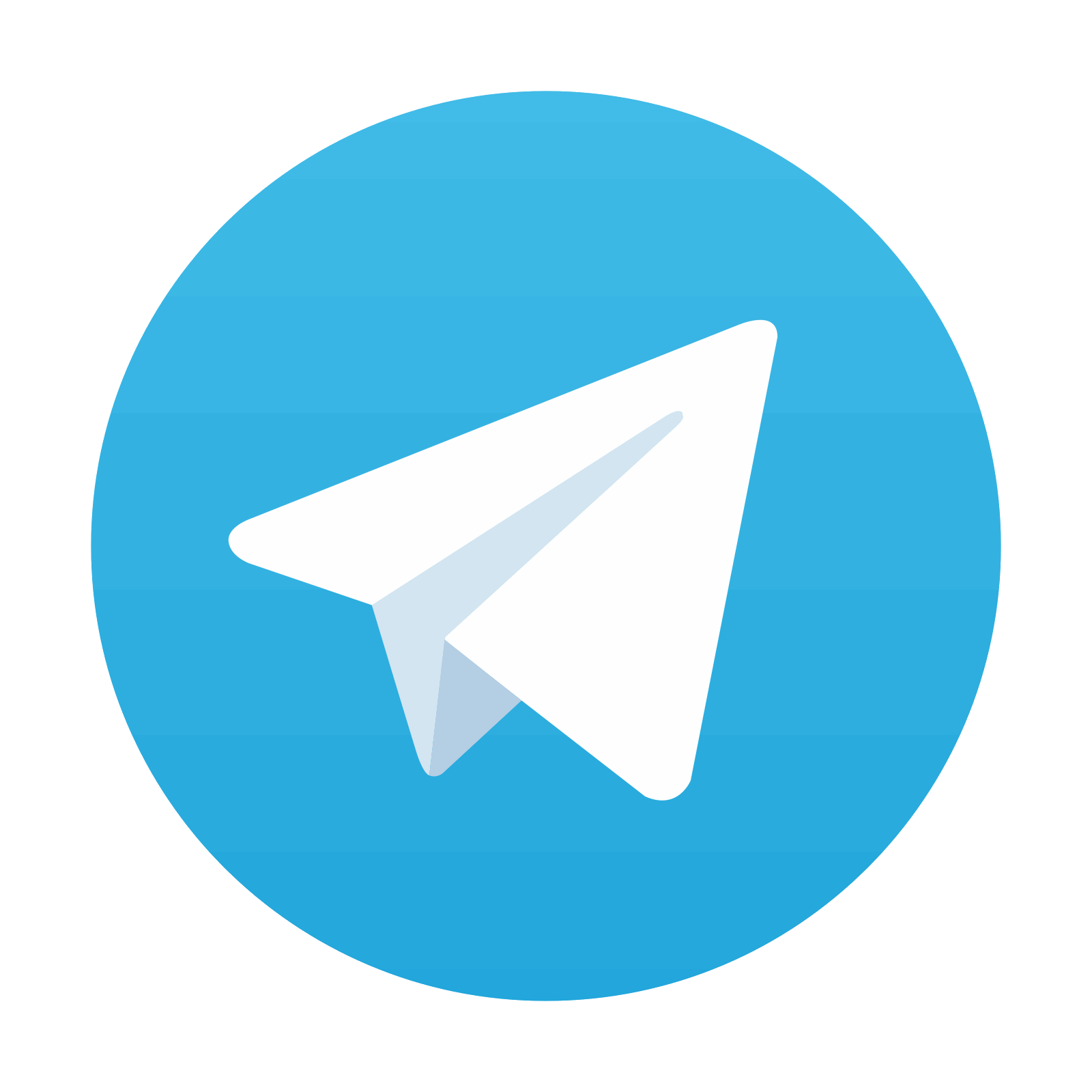
Stay updated, free articles. Join our Telegram channel

Full access? Get Clinical Tree
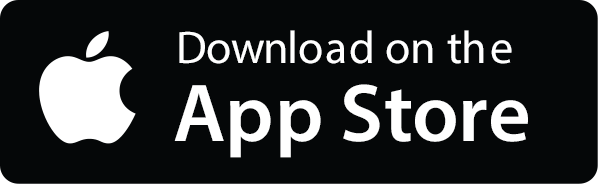
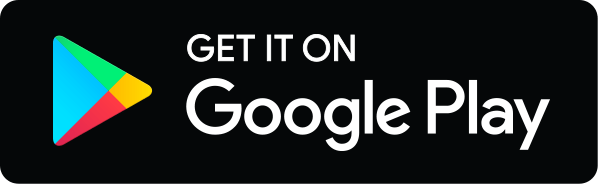