Fig. 1
Schematic, showing proposed mechanisms involving this preliminary investigation, directives of further study
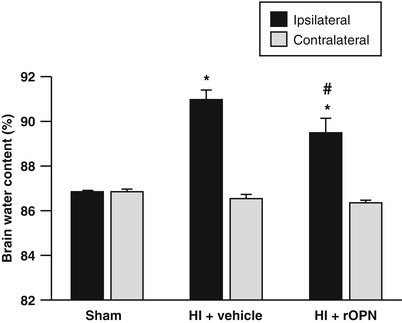
Fig. 2
OPN reduced brain edema. To determine the effects of OPN on BBB permeability, brain water content, which is a measure of brain edema, was evaluated. Brain edema was significantly increased in the ipsilateral hemisphere following HI induction. OPN significantly reduced brain edema compared with vehicle group. The contralateral hemisphere showed no increase in edema (*p < 0.05 vs. sham; #p < 0.05 vs. vehicle; N = 4/group)
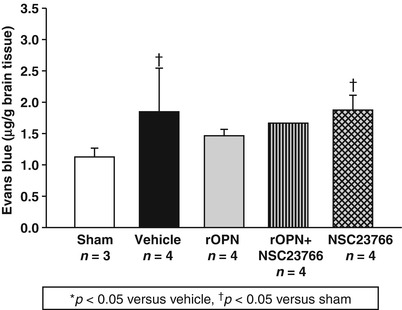
Fig. 3
OPN reduced BBB permeability. To determine the effect of OPN on BBB permeability, Evans blue dye extravasation as a measure of BBB permeability was evaluated. OPN (5 μg) was administered following HI induction, and HI significantly increased Evans blue dye extravasation, as seen in the ipsilateral hemisphere, which was significantly attenuated by OPN treatment
Conclusion
Translational stroke studies, including animal modeling, are greatly needed to safely integrate basic preclinical scientific principles ahead of clinical application [22–26]. Numerous attempts to reduce HI-induced consequences have failed in the clinical setting. Therefore, it is imperative to create translatable studies that incorporate unique characteristics of the immature brain, such as its anatomical structure, physiological function, cellular composition, and its response to injury [14, 25, 27–36].
The specific objective of this study was to determine a mechanism by which rOPN affected BBB permeability and brain edema in a well-established animal model of neonatal HI. Our data demonstrate that administration of rOPN improves recovery after neonatal HI by stabilization of the BBB, possibly via integrin receptor signaling pathway. The long-term goal of this study is to provide a further basis for the clinical translation of rOPN as an effective therapeutic option with long-term benefits.
In summary, this study showed that OPN protected the BBB following nHI, and this was reversed by Rac1 inhibitor (NSC23766). The mechanisms mediating this neuroprotection warrant further study.
Acknowledgment
This study was partially supported by the National Institutes of Health grant RO1 NS078755 (Dr. Zhang) and American Heart Association CRP 17380009 (Dr. Lekic).
Disclosures
None
References
1.
2.
Murphy BP, Inder TE, Rooks V, Taylor GA, Anderson NJ, Mogridge N, Horwood LJ, Volpe JJ (2002) Posthaemorrhagic ventricular dilatation in the premature infant: natural history and predictors of outcome. Arch Dis Child Fetal Neonatal Ed 87:F37–F41PubMedCentralCrossRefPubMed
3.
4.
Suzuki H, Ayer R, Sugawara T, Chen W, Sozen T, Hasegawa Y, Kanamaru K, Zhang JH (2010) Protective effects of recombinant osteopontin on early brain injury after subarachnoid hemorrhage in rats. Crit Care Med 38:612–618PubMedCentralCrossRefPubMed
5.
Chen W, Ma Q, Suzuki H, Hartman R, Tang J, Zhang JH (2011) Osteopontin reduced hypoxia-ischemia neonatal brain injury by suppression of apoptosis in a rat pup model. Stroke 42:764–769PubMedCentralCrossRefPubMed
6.
7.
Suzuki H, Hasegawa Y, Chen W, Kanamaru K, Zhang JH (2010) Recombinant osteopontin in cerebral vasospasm after subarachnoid hemorrhage. Ann Neurol 68:650–660PubMedCentralCrossRefPubMed
8.
Suzuki H, Hasegawa Y, Kanamaru K, Zhang JH (2010) Mechanisms of osteopontin-induced stabilization of blood-brain barrier disruption after subarachnoid hemorrhage in rats. Stroke 41:1783–1790PubMedCentralCrossRefPubMed
9.
Topkoru BC, Altay O, Duris K, Krafft PR, Yan J, Zhang JH (2013) Nasal administration of recombinant osteopontin attenuates early brain injury after subarachnoid hemorrhage. Stroke 44:3189–3194PubMedCentralCrossRefPubMed
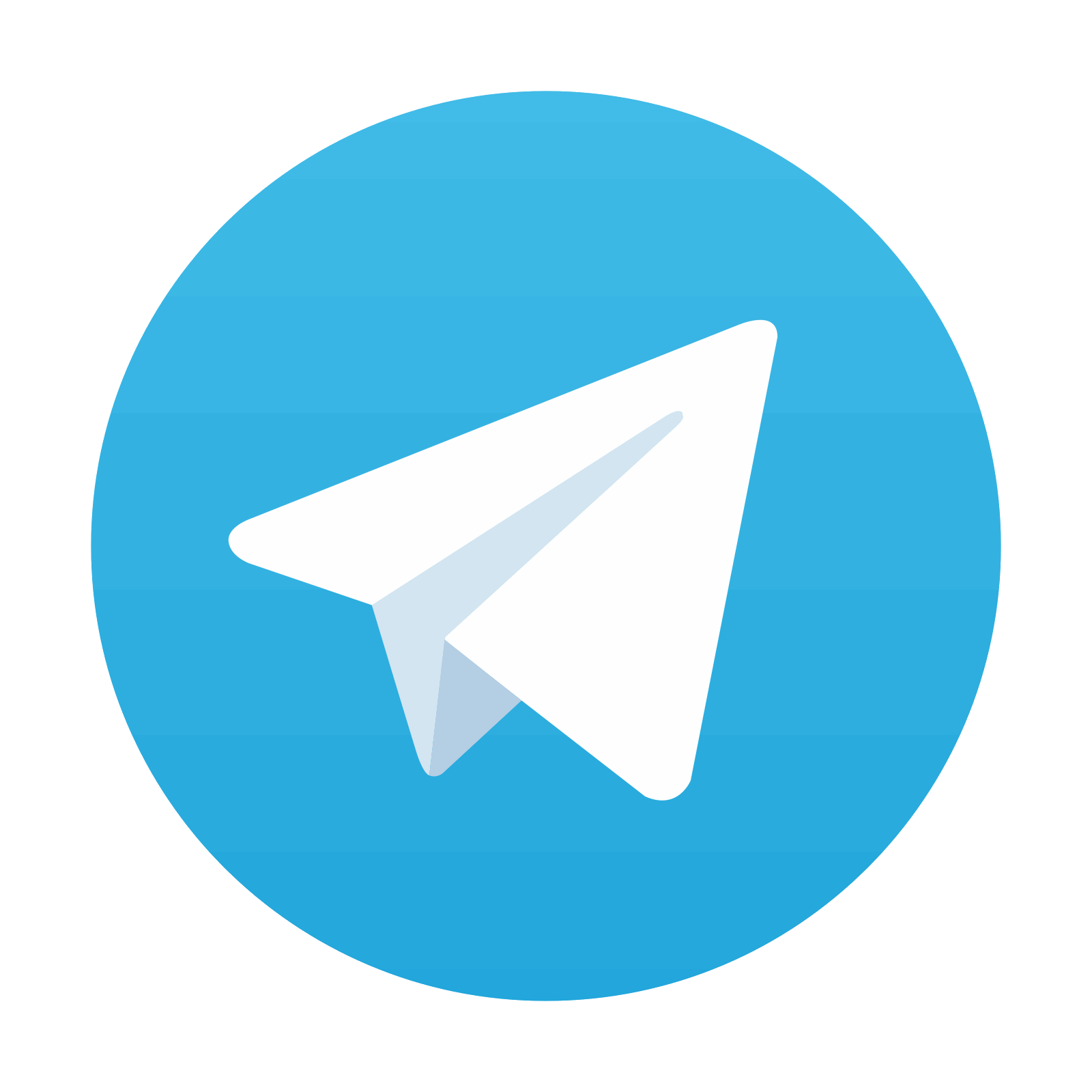
Stay updated, free articles. Join our Telegram channel

Full access? Get Clinical Tree
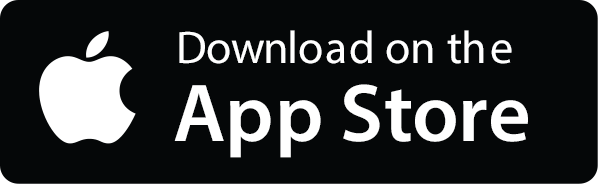
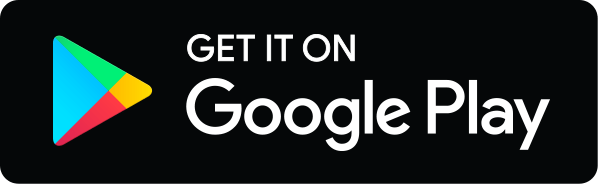