html xmlns=”http://www.w3.org/1999/xhtml” xmlns:mml=”http://www.w3.org/1998/Math/MathML” xmlns:epub=”http://www.idpf.org/2007/ops”>
Introduction and history
In 1960 and 1967, Hornykiewicz and Cotzias opened a new era in the management of neurodegenerative diseases by the demonstration of decreased levels of dopamine in the striatum and the indisputable effects of oral levodopa (l-DOPA) on parkinsonian motor symptoms [1, 2]. Nevertheless, within a few years of the introduction of levodopa, clinicians recognized several problems associated with its continued use. If the chronic use of levodopa was not associated with the development of motor complications – either motor fluctuations or dyskinesias – levodopa would still stand as the drug to use in patients with Parkinson’s disease (PD). Other dopaminergic agents most likely would not have been developed, and the progressive impairment of dopamine-dependent PD motor deficits would be well controlled by increasing doses of levodopa. However, as has been well established, PD patients in monotherapy with levodopa develop motor complications after 5–10 years of treatment onset. In community-based studies of patients treated with levodopa, disabling motor complications develop in up to 80% of PD patients after 10 years of disease onset [3].
In fact, levodopa is still the most effective symptomatic treatment of PD. In the early stages of the disease, the response to levodopa is sustained during both the daytime and nighttime, despite its relatively short half-life. Even though more than 50% of neurons in the substantia nigra pars compacta are already lost when parkinsonian motor symptoms emerge, the remaining neurons preserve enough presynaptic nerve terminals so as to store and release for 24h the available endogenous and exogenous dopamine. As the disease progresses, the beneficial effect of each dose of levodopa progressively shortens, and postsynaptic striatal receptors need to change their conformation and sensitivity to dopamine to maintain the effects of dopamine on dopamine for as long as possible receptors [4, 5]. The combination of pre- and postsynaptic changes progressively leads to more unpredictable motor fluctuations and the development of dyskinesias [4, 6, 7].
To overcome the limitations in the use of levodopa, new drugs with longer pharmacokinetic properties have been explored. Monoamine oxidase B (MAO-B) inhibitors and dopamine agonists (DAs) had longer half-lives and improved motor function in animal models [8, 9]. In addition, since DAs act directly on dopamine receptors, their efficacy was not supposed to be modified by the degenerating dopaminergic cells. Both the MAO-B inhibitor selegiline, and the DA bromocriptine entered into the clinical field, but the higher efficacy of bromocriptine on motor symptoms placed DAs as the drug family to complement levodopa in the management of PD [10, 11].
Compared with levodopa, treatment of parkinsonism with bromocriptine – either alone or in combination – resulted in fewer fluctuations and peak-dose dyskinesias after 3–5 years of treatment [11, 12]. Monotherapy with bromocriptine appeared to be a less effective stimulant than levodopa, but in combination achieved similar therapeutic responses with fewer motor complications [13, 14].
It is worth noting that psychiatric complications have appeared as a limiting factor in DA use since the first clinical trials. Hallucinations, anxiety, psychomotor agitation and vivid dreams were related to the administration of high doses and bromocriptine and lisuride [12, 15]. These complications were related to dose effects and were controlled by adjusting effective therapeutic ranges, but they stress the specific effects that DAs have on limbic structures, and the deleterious complications this may entail.
After bromocriptine, newer ergot-derived DAs emerged. Pergolide was an extremely potent and effective DA, and cabergoline, due to its long half-life, stood as a very good drug to deliver continuous dopaminergic stimulation. However, restrictive valvular heart disease and serositis appeared as adverse events common to all ergot-derived DAs, so that changing to a nonergot DA was advised [16, 17]. The nonergot-derived pramipexole and ropinirole have since been the most commonly used oral DAs, having proven to be beneficial to patients at all stages of the disease. Piribedil is also used in some countries, and rotigotine, a DA delivered through a silicone-based transdermal patch that is administered once daily, provides a stable drug release throughout the 24h patch application [18].
During the last decade, the growing description of impulse control disorders (ICDs) in PD associated with the use of DAs [19, 20] – at both low and high doses – may alert physicians to remain cautious in their use, trying to select which patients may benefit.
In this chapter, we will review the evidence of the effectiveness and safety of pramipexole, ropinirole, piribedil and rotigotine in the management of PD.
Rationale for the use of dopamine agonists in Parkinson’s disease
The National Institute for Health and Clinical Excellence (NICE) guidelines stated in 2011 that “there is no single drug choice for the initial pharmacotherapy of early PD” [21]. In the evidence-based reviews of the American Academy of Neurology, DAs have enough evidence to consider their use in both the symptomatic treatment of patients with early PD [22] and for the prevention and management of motor complications [23]. In addition, due to the higher risk of motor complications and the greater expectation of life of younger patients, there is a strong argument for using DAs as first-line therapy in patients younger than 60 years.
In the elderly patient, the use of DAs is not contraindicated but a balance between efficacy and adverse events must be taken into account. As indicated before, the description of ICDs in younger patients must be now carefully considered, and the relevant information given to patients when DAs are indicated. Nevertheless, the fact that DAs are currently one of the most relevant drug families in the management of PD is because they have given clear evidence in favor of delaying and even decreasing the prevalence of motor complications, as reported when levodopa was used in monotherapy [3].
The longer half-lives of available DAs seems almost certainly the reason why motor fluctuations and dyskinesias are less prevalent. Beyond age and genetic factors, the more continuous dopaminergic stimulation a drug may achieve seems a major variable for preventing motor fluctuations [24]. Levodopa-related motor complications in PD are associated with nonphysiological, discontinuous or pulsatile stimulation of striatal dopamine receptors, and can be prevented or reversed by long-acting dopaminergic drugs, such as DAs, that provide more continuous stimulation of striatal dopamine receptors.
That the long life of available DAs is crucial for the prevention of motor complications was reinforced by studies using experimental DAs with short half-lives. The use in animal models of intermittent injections of the short-acting DAs, such as apomorphine or U-91356A, rapidly induced dyskinesias, whereas continuous infusion of the same drug did not. Thus, the same drug may or may not induce motor complications depending only on its pharmacokinetic properties [25, 26].
Laboratory findings could be transferred to clinical studies. In patients with early PD, prospective double-blinded, controlled trials have shown that patients randomized to initiate therapy with long-acting DAs had a lower risk of developing motor complications in comparison with patients initiating treatment with levodopa. Initial pramipexole treatment resulted in significantly less development of wearing off, dyskinesias, or “on–off” motor fluctuations (28%) compared with levodopa (51%) after a follow-up of 2 years [27]. Using ropinirole, Rascol et al. [28] found that the cumulative incidence of dyskinesias after 5 years of treatment onset was significantly lower in the ropinirole group (20%) compared with levodopa (45%), and Whone et al. [29] obtained similar results after a follow-up of only 2 years, with significantly fewer patients in the ropinirole group (3.4%) developing dyskinesias compared with the levodopa group (26.75%).
Compelling data have recently come from prospective studies of patients who initiated treatment with bromocriptine 14 years ago. Whereas the majority of the patients were on combined treatment with bromocriptine and levodopa after 3–5 years of follow-up, the protective effects of bromocriptine for preventing the development of motor complications as a result of having initiated treatment with a DA were maintained for up to 10 years [30]. Even more interestingly, the prevalence of both disabling motor fluctuations and dyskinesias after 14 years of follow-up was much lower than that registered in previous historical community-based studies [3]. In the study by Schrag et al. [3] – in which nearly all patients had initiated treatment with levodopa – motor fluctuations and dyskinesias were present in 63 and 53% of PD patients with more than 10 years of disease evolution. In comparison, only 35% developed either motor fluctuations or dyskinesias in the 14-year study [30]. One possible reason for this important and clinically relevant difference is that in clinical series of patients following treatment with both levodopa and DAs, the total daily requirements of levodopa after 10–15 years of disease are significantly lower, which would protect patients from the uncontrolled oscillations in striatal and synaptic dopamine concentrations provoked by the pulsatile stimulation of the short-acting exogenous levodopa.
Pharmacology
Pharmacodynamics: dopamine receptors
Pharmacodynamics is the study of the effects of drugs in the body at any level. Understanding the effects of DAs requires a basic knowledge of dopamine receptors (Table 5.1). There are two classes of dopamine receptors: D1 (constituted by D1 and D5 subtypes) and D2 (constituted by D2, D3 and D4 subtypes). D1 class receptors are coupled to a Gs protein, which activates adenylyl cyclase mediating an excitatory response in the postsynaptic neuron. D2 class receptors are coupled to a Gi protein, which inhibits adenylyl cyclase leading to inhibition in the postsynaptic neuron. The D1 and D2 subtypes are located in the striatum forming the nigrostriatal pathway. D1 mainly excites the direct pathway, while D2 inhibits the indirect pathway. This implies that activating any of these receptors can relieve bradykinesia caused by dopaminergic depletion [31]. D2 also has a major role in the tuberoinfundibular pathway inhibiting prolactin release and in the area postrema leading to nausea and vomiting. This effect can be blocked without affecting other central D2 effects, with antidopaminergics unable to cross the blood–brain barrier as the area postrema is located outside of it [32].
Affinities of dopamine agonists to various receptors
D1 | D2 | D3 | D4 | D5 | α2 | Other | |
---|---|---|---|---|---|---|---|
Piribedil | 0 | ++ | +++ | − | ? | − | 0 |
Pramipexole | 0 | +++ | +++ | ++ | 0 | +/− | 0 |
Ropinirole | 0 | +++ | +++ | 0 | 0 | +/− | +/−5HT2 |
Rotigotine | + | ++ | +++ | ++ | ++ | − | 5HT1a* |
+, Agonist; –, antagonist; +/–, very low affinity; 0, no affinity;?, unknown, *, partial agonist.
In the peripheral nervous system, the D1 main effects are vasodilatation and natriuresis leading to hypotension, while D2 decreases noradrenaline release, indirectly lowering blood pressure. This peripheral side effect could also be related to peripheral edema, and a clinical trial was designed to avoid this with domperidone, but the study was terminated due the lack of recruitment [33].
D3, D4 and D5 act mainly in the mesolimbic and mesocortical pathways. D3 is the best studied and has been implied in addiction, psychosis and depression. D4 is mostly known for its implication in attention-deficit hyperactivity disorder and novelty-seeking traits [34]. D5 is distributed mainly in the prefrontal cortex. Its effects are less well known because no selective agonists are known; therefore, studies of dopamine effects on the prefrontal cortex usually do not differentiate between the D1 and D5 subtypes.
Aside from these five subtypes, heteromeric D1/D2 receptors are known in rodents. Interestingly, their main effect differs from both subtypes, as they are coupled to Gq proteins, which increase intracellular Ca2+ concentration [35]. D2 receptors are expressed in two forms: high affinity and low affinity. An increase in high-affinity D2 receptors has been shown in animal models of several disorders with dopamine hypersensitivity. Nevertheless, there have been no clinical studies yet [36]. Probably there are more forms of dopamine receptors (either heteromeric or produced by alternative splicing) and these discoveries may have potential implications for new DAs or even for choosing better among the current ones.
Each DA has a different affinity for each dopamine receptor, but it should be noted that ligands for D1 also have a high affinity for D5 and, in general, ligands for D2 also join D3 and D4. Nowadays, all DAs indicated for PD have D2 agonist activity varying in the degree to which they activate D1 and D3. No D1 selective agonist is in use for PD because of low bioavailability and a strong hypotensive effect, although they have proven antiparkinsonian effects in nonhuman primate models [37].
Pharmacokinetics
Pharmacokinetics is the study of the processes undergone by a drug from its administration to its complete elimination from the body. Pramipexole, ropinirole and piribedil are rapidly absorbed after oral administration reaching peak plasma concentration in 1–2h (Table 5.2). Food delays cause peak plasma concentrations but do not affect the extent of absorption. Rotigotine is administered transdermally.
Pharmacokinetics of dopamine agonists
Drug | Bioavailability | Protein binding | Half-life | Metabolism | Excretion |
---|---|---|---|---|---|
Piribedil | Low | Low | 1.7–6.9 h | Hepatic | Renal 68%, fecal 25% |
Pramipexole | >90% | 15% | 8–12 h | None | Urine 90%, fecal 2% |
Ropinirole | 50% | 40% | 5–6 h | Hepatic (CYP1A2) | Renal |
Rotigotine | 37% transdermal | 92% | 5–7 h | Hepatic (several CYP) | Urine 71%, fecal 23% |
Rotigotine and ropinirole have a half-life of 5–7h. Piribedil has a half-life of 1.7 to 6.7h and that of pramipexole is about 8–12h. Cabergoline and pergolide have much longer half-lives, which allow a single daily dose, but all nonergoline DAs need to be taken several times per day. Modified-release formulations are available for all of the nonergoline agonist, also allowing a single dose, except in the case of piribedil, which still needs to be taken three to five times per day.
It should be noted that, in advanced fluctuating PD, motor control is sometimes improved by splitting the dose of an extended-release agonist taken four times daily into two separated extended-release doses. This has been studied for ropinirole [38] but may apply to other extended-release agonists (author’s observation).
Ropinirole, piribedil and rotigotine are metabolized in the liver, while pramipexole is excreted intact.
Rotigotine plasma protein binding is high, potentially leading to protein displacement interactions. Ropinirole binding is intermediate, while pramipexole and piribedil binding are lower. Dopamine agonists are usually titrated for several weeks, although their half-life is much shorter. Therefore, the time needed to reach a steady state depends mainly on the length of the titration period.
Dopamine agonists most commonly used in daily practice
Details of the levodopa-equivalent doses and therapeutic ranges for the DAs most commonly used in daily practice are given in Table 5.3.
Levodopa-equivalent doses and therapeutic range
Drug | Dose for 100 mg levodopa | Conversion factor | Therapeutic range |
---|---|---|---|
Levodopa | 100 | ×1 | 150–1200mg |
Bromocriptine | 10 | ×10 | 20–60mg |
Pergolide | 1 | ×100 | 3–8mg |
Piribedil | 60–100 | ×1–1.67 | 150–250mg |
Pramipexole | 0.7 | ×140 | 0.7–4.5mg |
Ropinirole | 6 | ×17 | 8–24mg |
Rotigotine | 3.3–4.5 | ×22–30 | 6–16mg |
Pramipexole
Mechanism of action
The nonergot DA pramipexole was approved for the treatment of PD in 1997 in the US and in 1998 in most European countries. Pramipexole acts as a potent agonist at D2 (Ki = 3.9nM), D3 (Ki = 0.5nM) and D4 (Ki = 5.1nM) dopamine receptors, but has negligible affinity (>10,000nM) for D1 and D5 receptors. Unlike the ergot DA, pramipexole has little or no interaction with adrenergic or serotonergic receptors [39]. The preferential affinity of pramipexole for the D3 receptor subtype has been (i) claimed to contribute to the efficacy of the drug for the treatment of affective disorders in PD; (ii) the base for exploring the efficacy of pramipexole in the treatment of primary major depression; and (iii) also advocated as one of the main sites responsible for the development of ICDs in patients with PD [20] or restless legs syndrome [40].
Pramipexole shows linear pharmacokinetics. It is rapidly and completely absorbed, with peak concentrations appearing in the bloodstream within 2h of oral administration. It can be administered without regard to meals, and excretion is primarily renal. Of particular interest, pramipexole is not appreciably metabolized by the cytochrome P450 system, which minimizes drug–drug interactions in elderly populations [43].
Efficacy on motor symptoms
Clinical trials of pramipexole have included over 1200 patients, with 700 patients receiving pramipexole for up to 4 years, with doses ranging from 0.375 to 4.5mg/day. As sustained by evidence, pramipexole can be used to improve motor impairments and disability in early PD patients, to reduce dyskinesias and total daily levodopa dose in advanced patients, and to reduce “off” time in patients with wearing off. These conclusions are based on short- and medium-term trials (up to 24 weeks) [44].
In early and fluctuating PD patients, treatment onset with pramipexole treatment has been shown to improve both the Unified Parkinson’s Disease Rating Scale (UPDRS) II (daily disability) and UPDRS-III (motor severity) sum scores by 30%, and to decrease “off” times by approximately 2.5h/day. Differences between groups are achieved, since daily doses of 0.75mg/day of pramipexole, but increasing doses up to 4mg/day are accompanied by further amelioration of symptoms [45]. In advanced patients, pramipexole not only improved fluctuations but has also proven to reduce the severity of motor dysfunction (UPDRS-III) during “off” periods [46].
The CALM-PD (Comparison of the Agonist Pramipexole versus Levodopa on Motor complications of Parkinson’s Disease) trial explored the potential benefits of pramipexole for preventing the development of motor complications after a follow-up of 4 years. Patients could start treatment with either pramipexole 0.5mg three times daily or levodopa 100mg three times daily, and during the follow-up, levodopa supplementation was permitted, as required. Time to the first occurrence of dopaminergic complications (wearing off, dyskinesias, “on–off” or freezing) was determined. After 2 years, the prevalence of motor complications was significantly lower in patients with initial pramipexole treatment compared with the levodopa group (28 vs 51%; hazards ratio = 0.45; P < 0.001), and these benefits were maintained at 4 years (52 vs 74%; hazards ratio = 0.48; P < 0.001). The results at 6 years were also published, with PD patients who started treatment with pramipexole still showing both less wearing off (44.4 vs 58.8%; P = 0.01) and fewer dyskinesias (20.4 vs 36.8%; P = 0.004) [47]. Interestingly, the UPDRS total and motor scores, and scores on disability (Schwab and England scores) and Parkinson’s Disease Quality of Life (PDQUALIF) scales did not differ significantly.
In patients with advanced PD and motor fluctuations, pramipexole has a proven efficacy for (i) improving 30–40% UPDRS total and motor scores when compared with placebo; (ii) decreasing levodopa daily doses in up to 30% of patients; and (iii) reducing daily “off” times in 30% of patients [45, 46]. In particular, when compared with entacapone, pramipexole was found to be significantly more efficacious for both improving UPDRS scores and for diminishing daily “off” times [48].
In terms of neuroprotection, study designs based on dopamine transporter imaging with single-photon-emission computed tomography (DAT-SPECT) reuptake changes have not been conclusive (CALM-PD trial) [49]. Following the methodology of a randomized, double-blind, placebo-controlled, delayed-start trial – as had been used previously to disclose the potential neuroprotective benefits of rasagiline – the PROUD (Pramipexole On Underlying Disease) study did not find pramipexole to delay the progression of motor symptoms in early PD patients treated for 6–9 months [50, 51]. As a result, it remains an open question as to whether pramipexole may confer additional neuroprotective effects.
Efficacy on nonmotor symptoms
The affinity of pramipexole on D3 dopamine receptors was used first in psychiatry to explore whether this drug might have antidepressant properties in monotherapy or as an add-on treatment in patients with primary major depression [52].
In PD patients, observational and open-label studies in which pramipexole has been used as an add-on therapy to levodopa have consistently shown significant improvements in depressive scales [53, 54]. In addition, in a double-blind, placebo-controlled study, pramipexole was shown to improve depressive symptoms through a direct antidepressant effect, independent from motor symptoms alleviation [55]. In a comparative study of pramipexole versus sertraline, both drugs achieved significant improvements in depressive symptoms, but in the pramipexole group, the proportion of patients with remission from depression was significantly higher [56]. Pramipexole has also achieved improvements in studies using specific scales for anhedonia [57]. In contrast, due to the lack of use of specific scales, the effects of pramipexole on apathy are still inconclusive. However, as assessed by the motivational items in the UPDRS-I and the Non-Motor Symptoms Scale (NMSS), pramipexole clearly improved apathy [53]. It must be noted that none of the studies that analyzed the antidepressant effect of pramipexole used scales to measure depression and apathy separately [55], making it difficult to determine whether pramipexole improved sadness and negativity, or whether changes in depression scales were more related to the alleviation of loss of interest and blunted affect [58, 59].
Regarding cognition, no studies have assessed the issue properly, but no changes were observed in the long follow-up of the CALM-PD trial.
Ropinirole
Mechanism of action
Ropinirole is a D2 and D3 agonist with no effect on D1, D4 and D5 subtypes. It has no affinity for other receptor families. Ropinirole immediate release is absorbed quickly, reaching peak plasma concentrations in 1–2h. It has a half-life of 5.5h, requiring three immediate-release doses per day. Modified-release presentations are commercialized, allowing one only dose per day. Nevertheless, a study has shown improvement in wearing-off control by splitting the dose into two extended-release pills, at least according to patients’ impression [38]. Bioavailability is 50% in both cases, and transition from immediate release to extended release is performed easily overnight (just add up the daily dose of immediate release and substitute with the extended-release formulation with the closest dose).
Hepatic CYP1A2 is the major P450 enzyme metabolizing ropinirole. Therefore, the drug interacts with CYP1A2 inhibitors (including but not limited to fluoroquinolones, fluvoxamine, verapamil, caffeine and grapefruit) and inducers (including but not limited to omeprazole, tobacco, broccoli and modafinil) [60]. It is usually titrated up to 8mg/day (or 9mg in three divided doses) over 8 weeks. The maximum dosage is 24mg/day.
Efficacy on motor symptoms
Ropinirole appeared superior to placebo in a double-blinded trial on early PD. Patients in the active group had better motor scores and fewer required levodopa during the 6-month treatment. It has also been proven to delay motor complications in a 5-year follow-up [28] and to prevent changes in postsynaptic availability of D2 receptors on positron emission tomography (PET) studies [29].
Ropinirole was also studied in PD with motor fluctuations for the reduction of levodopa dose and duration of “off” periods. Both were lowered in active and control groups, but the reductions were greater in patients treated with ropinirole [61].
Efficacy on nonmotor symptoms
In an open-label study, ropinirole was shown to relieve depression and anxiety in PD patients suffering motor complications, but no improvement was observed in patients without motor complications [62].
Ropinirole side effects are similar to other nonergoline DAs. Meta-analysis of clinical trials [63] has shown fewer cognitive adverse effects than other agonists, while another meta-analysis showed a higher risk for nausea, dizziness and somnolence than pramipexole although fewer hallucinations and less confusion (although the results were not significant) [64]. An indirect comparison in early PD against pramipexole showed more gastrointestinal and fewer cognitive adverse effects [63]. Nevertheless, the frequency of ICDs is similar to other DAs [20].
Rotigotine
Mechanism of action
A rotigotine transdermal patch was approved by the regulatory authorities for use in all stages of PD in 2007, at doses of 2–8mg/day in early PD or 4–16mg/day in advanced patients. After some problems with crystal formation in the patches, they were reformulated and reintroduced in 2012.
Rotigotine is analogous to 7-OH-DPAT and UH-232, all three of which are aminotetralin derivatives. These compounds are similar in structure to dopamine, likely underlying their pharmacology. Rotigotine has agonistic activity on all dopamine-receptor subtypes (D1–D5), but demonstrates its highest affinity for the D3 receptor (Ki = 0.71nM), and acts as a potent agonist at D4 (Ki = 3.9nM), D5 (Ki = 5.4nM) and D2 (Ki = 13.5nM); affinity for D1 receptors is lower (Ki = 83nM). Rotigotine also possesses antagonistic activities at the α2-adrenergic receptor, and is a partial agonist at the serotonin 5-HT1A receptor [65].
In 70 patients with early PD who were treated with rotigotine at up to 18mg/day, mean plasma concentrations decreased slightly after application of a new patch but concentrations remained similar independent of the application site, and stable drug blood levels were stable over a 24-h period. Rotigotine’s pharmacokinetics are dose proportional and are not affected by renal impairment, and because of the transdermal administration route, food and/or gastrointestinal conditions do not influence the pharmacokinetics of the drug. The majority of the rotigotine dose is excreted in the urine (71%), with approximately 23% excreted in the feces [66].
Efficacy on motor symptoms
Published clinical studies with rotigotine include three large-scale phase III studies in early PD, two large-scale phase III studies in advanced PD, and five smaller phase 2 trials in early and advanced PD [66].
In the first open-label uncontrolled dose-escalation study of rotigotine monotherapy in early PD, patients received rotigotine to a maximum dose of 18mg/day, which resulted in significant improvement of motor function (−4.62 ± 5 points on UPDRS-III; P < 0.0001) and functional disability (−2.76 ± 3 points on UPDRS-II; P = 0.0001) [67].
Three double-blind, placebo-controlled studies were then carried out. In early PD, significant improvements were observed from doses of 2mg/day, reaching a response plateau at 8mg in 24h. One of these studies compared the efficacy of rotigotine with ropinirole over a 37-week period, showing a similar rate of responders for rotigotine ≤8mg/day (52%) and ropinirole ≤24mg/day (68%), and noninferiority to ropinirole, with UPDRS-II + -III mean decrease of −7.2 versus −11.0 points. It must be noted that the mean dose of ropinirole used in this trial was unusually high compared with other published clinical trials, and when post-hoc analysis compared rotigotine 8mg with ropinirole 12mg, the improvement in UPDRS-III was more similar (−7.2 versus −7.6) [68].
In large double-blinded controlled studies in advanced PD patients, rotigotine 8–12mg/day significantly improved the UPDRS-II score by 2.7–3.5 points and UPDRS-III by 5.3–5.9 points, as well as the quality of life as assessed by the 39-Item Parkinson’s Disease Questionnaire (PDQ-39) [69, 70]. In PD patients with motor complications, the CLEOPATRA-PD (Clinical Efficacy of Pramipexole and Transdermal Rotigotine in Advanced PD) study showed noninferiority of rotigotine (13mg/day) against pramipexole (3.1mg/day), with similar improvements in motor and disability scales, and significant reductions in daily “off” time, achieving responder rates (≥30% reduction in “off” time) of 60% for rotigotine and 67% for the pramipexole group [70].
In the double-blind, placebo-controlled RECOVER (Randomized Evaluation of the 24-Hour Coverage: Efficacy of Rotigotine) study, early-morning motor function was assessed by the UPDRS-III before a new dose of rotigotine was administered. At the end of the maintenance period, rotigotine significantly improved the UPDRS-III score by 7 points, with parallel improvements in quality-of-life scales [71].
Efficacy of nonmotor symptoms
The RECOVER study also assessed important nonmotor aspects of PD. The PD Sleep Scale (PDSS-2), NMSS and the Beck Depression Inventory (BDI) were administered to explore potential significant benefits compared with placebo. The NMSS represents a set of 30 questions about the severity and frequency of nonmotor symptoms in several domains. A greater and significant improvement was seen in NMSS total scores in the rotigotine group (P = 0.015). These results were evaluated in more detail in a post-hoc analysis, which concluded that total score NMSS changes were explained mainly by the significant amelioration of specific items assessing the degree of sleep, fatigue, sadness, anhedonia, and loss of interest in surroundings or in doing things [72]. The significant improvement in global sleep quality measured by the PDSS-2 was observed to cover different sleep disturbances, with significant and more specific changes in restless legs-like symptoms, motor nocturnal disability (uncomfortable and immobile postures, muscle cramps, pain in arms and legs, breathing problems) and early-morning parkinsonism (waking tremor and painful posturing). Mood changes were reinforced by the parallel improvement observed in BDI total scores (P = 0.01) [71, 73]. Rotigotine’s effects on cognitive impairment have not been assessed yet in this or other studies.
Piribedil
Mechanism of action
Piribedil is the oldest nonergoline dopamine agonist still in use. It was introduced in the 1970s and, in addition to PD, it was approved in some countries for moderate cognitive impairment not constituting dementia, peripheral vasculopathies and retinal. Currently, it is still in use for PD, both in monotherapy and as adjunctive therapy. It activates D2 and D3 receptors and also inhibits α2 receptors.
Piribedil is absorbed rapidly after oral administration. It has the shortest half-life among the oral DAs requiring several doses per day, even for the extended-release formulation. In monotherapy, its titration is performed by increasing a 50mg extended-release pill weekly. The common therapeutic range is 150–250mg/day. When supplementing levodopa, 20mg of piribedil is given per 100mg of levodopa and the common range is 80–140mg/day. Piribedil is metabolized in the liver, although the exact pathway is unknown. It is excreted in both bile and urine [74, 75].
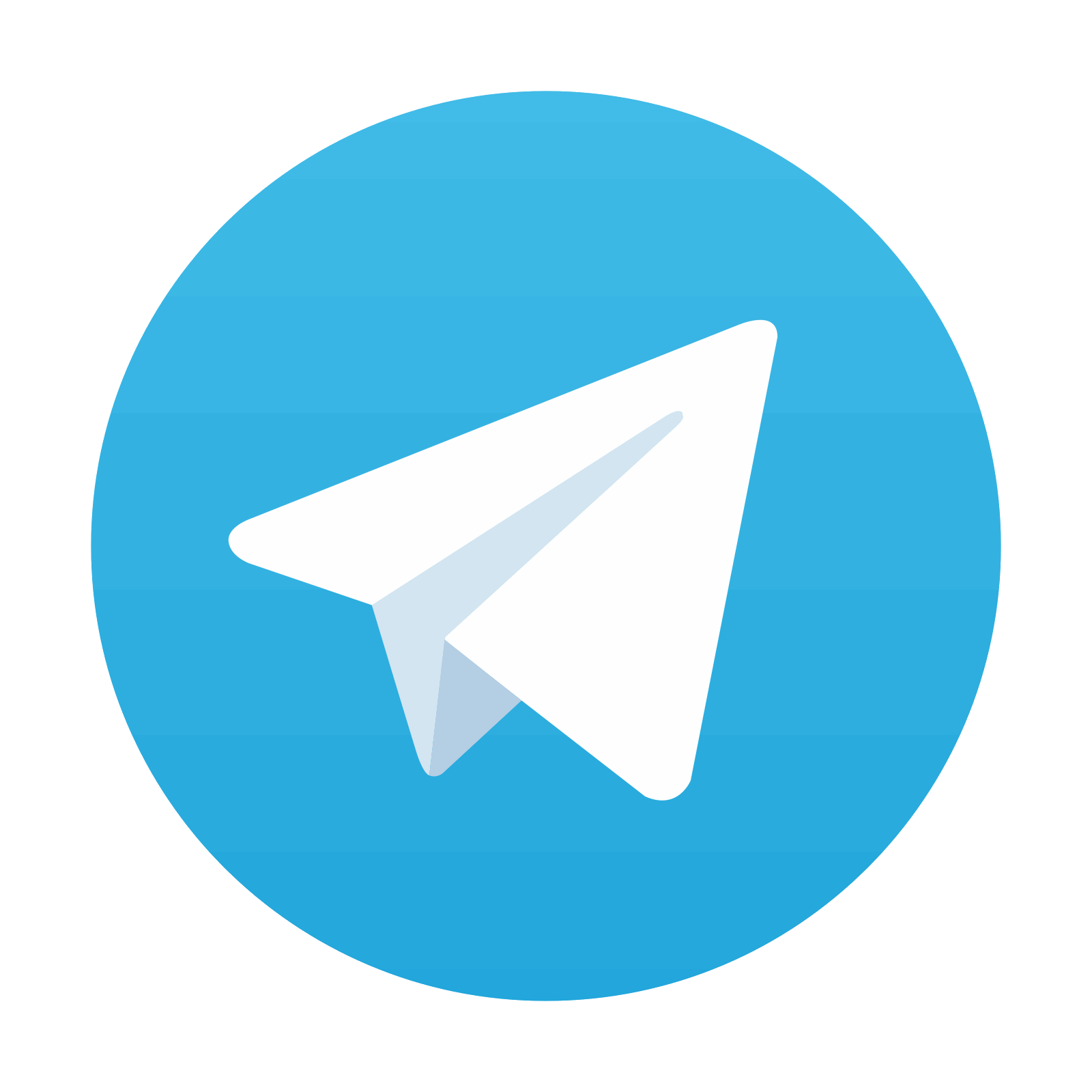
Stay updated, free articles. Join our Telegram channel

Full access? Get Clinical Tree
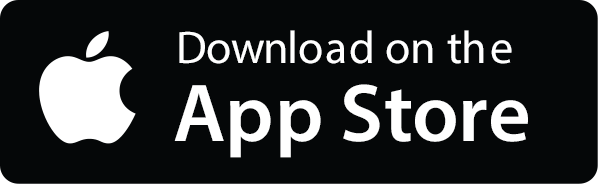
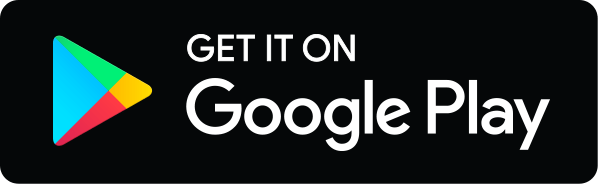