Fig. 2.1
Waking up of the giant Kumbhakarna, brother of Ravana in the great Indian epic Ramayana (circa 1000 B.C.) by hitting him with weapons and clubs, and shouting in his ear. This is a historical example of pathologic sleepiness resembling contemporary Kleine-Levin syndrome
Throughout the literature, a close relationship between sleep and death has been perceived, but the rapid reversibility of sleep episodes differentiates sleep from coma and death. There are myriad references to sleep, death, and dream in poetic and religious writings, including the following quotations: “The deepest sleep resembles death” (The Bible, I Samuel 26:12); “sleep and death are similar … sleep is one-sixtieth [i.e., one piece] of death” (The Talmud, Berachoth 576); “There she [Aphrodite] met sleep, the brother of death” (Homer’s Iliad, circa 700 B.C.); “To sleep perchance to dream … For in that sleep of death what dreams may come?” (Shakespeare’s Hamlet); “How wonderful is death; Death and his brother sleep” (Shelly’s “Queen Mab”).
The 3 major behavioral states in human—wakefulness, non-rapid eye movement (NREM), and rapid eye movement (REM) sleep—are three basic biological processes that have independent functions and controls. The reader should consult Borbely’s monograph Secrets of Sleep [2] for an interesting historical introduction to sleep.
What is the origin of sleep? The words sleep and somnolence are derived from the Latin word somnus; the German words sleps, slaf, or schlaf; and the Greek word hypnos. Hippocrates, the father of medicine, postulated a humoral mechanism for sleep and asserted that sleep was caused by the retreat of blood and warmth into the inner regions of the body, whereas the Greek philosopher Aristotle thought sleep was related to food, which generates heat and causes sleepiness. Paracelsus, a sixteenth-century physician, wrote that “natural” sleep lasted 6 h, eliminating tiredness and refreshing the sleeper. He also suggested that people not sleep too much or too little, but awake when the sun rises and go to bed at sunset. This advice from Paracelsus is strikingly similar to modern thinking about sleep. Views about sleep in the seventeenth and eighteenth centuries were expressed by Alexander Stuart, the British physician and physiologist, and by the Swiss physician, Albrecht von Haller. According to Stuart, sleep was due to a deficit of the “animal spirits”; von Haller wrote that the flow of the “spirits” to the nerves was cut off by the thickened blood in the heart, resulting in sleep. Nineteenth-century scientists used principles of physiology and chemistry to explain sleep. Both Humboldt and Pfluger thought that sleep resulted from a reduction or lack of oxygen in the brain [2].
Ideas about sleep were not based on solid scientific experiments until the twentieth century. Ishimori [6] in 1909, and Legendre and Pieron [7] in 1913, observed sleep-promoting substances in the cerebrospinal fluid of animals during prolonged wakefulness. The discovery of the EEG waves in dogs by the English physician Caton [8] in 1875 and of the alpha waves from the surface of the human brain by the German physician Berger [9] in 1929 provided the framework for contemporary sleep research. It is interesting to note that Kohlschutter, a nineteenth-century German physiologist, thought sleep was deepest in the first few hours and became lighter as time went on [2]. Modern sleep laboratory studies have generally confirmed these observations.
The golden age of sleep research began in 1937 with the discovery by American physiologist Loomis et al. [10] of different stages of sleep reflected in EEG changes. Aserinsky and Kleitman’s [11] discovery of REM sleep in the 1950s at the University of Chicago electrified the scientific community and propelled sleep research to the forefront. Observations of muscle atonia in cats by Jouvet and Michel in 1959 [12] and in human laryngeal muscles by Berger in 1960 [13] completed the discovery of all major components of REM sleep. Following this, Rechtschaffen and Kales produced the standard sleep scoring technique monograph in 1968 (R–K scoring technique) [14] which remained the gold standard until now. Recently, the American Academy of Sleep Medicine (AASM) published the AASM manual for the scoring of sleep and associated events [15] which modified the R–K technique and extended the scoring rules. The other significant milestone in the history of sleep medicine was the discovery of the site of obstruction in the upper airway in obstructive sleep apnea syndrome (OSAS) independently by Gastaut et al. [16] in France as well as Jung and Kuhlo [17] in Germany in 1965, followed by the polygraphic observations in the same year by Lugaresi et al. [18] of obstructive central and mixed apnea in these patients associated with periodic fall of blood pressure (BP) during apnea and rise above the baseline on resumption of breathing. The next milestone was the demonstration of dramatic relief of symptoms in these patients following tracheostomy (which bypasses the upper airway obstruction) by Kuhlo et al. [19]. In 1969 in a brief polygraphic report, Chokroverty et al. [20] made two important observations in patients with obesity hypoventilation syndrome (Pickwickian syndrome): Oxygen inhalation produced more prolonged and frequent episodes of apneas–hypopneas indicating the importance of peripheral chemoreceptor-driven hypoxemia causing respiratory stimulation and arousal in the presence of chronic daytime hypercapnia (these findings were later confirmed by other investigators [21]); the other observation is that following weight loss of 100–150-pound patients’ symptoms improved, daytime arterial carbon dioxide normalized but apneas–hypopneas persisted, though these were less frequent than before weight loss. Subsequently, numerous papers were published by Guilleminault et al. [22] who coined the term sleep apnea syndrome. Then came the seminal paper by Sullivan and associates in 1981 [23] of continuous positive airway pressure (CPAP) titration to eliminate such obstruction as the standard treatment modality for moderate-to-severe OSAS. Finally, identification of 2 neuropeptides, hypocretin 1 and 2 (orexin A and B) in the lateral hypothalamus and perifornical regions [24, 25] followed by an animal model of a human narcolepsy phenotype in dogs by mutation of hypocretin 2 receptors (HCTR2) by Lin et al. [26], the creation of similar phenotype in pre-prohypocretin knockout mice [27] and transgenic mice, [28] documentation of decreased hypocretin 1 in the cerebrospinal fluid in humans, [29] and decreased hypocretin neurons in the hypothalamus at autopsy [30, 31] in human narcolepsy patients opened a new and exciting era of sleep research.
Definition of Sleep
Sleep is “…great nature’s second course, chief nourisher in life’s feast” (Wm. Shakespeare).
The definition of sleep and a description of its functions have always baffled scientists. Moruzzi [32] while describing the historical development of the deafferentation hypothesis of sleep quoted the concept Lucretius articulated 2000 years ago—that sleep is the absence of wakefulness. A variation of the same concept was expressed by Hartley [33] in 1749, and again in 1830 by Macnish [34] who defined sleep as suspension of sensorial power, in which the voluntary functions are in abeyance, but the involuntary powers, such as circulation or respiration, remain intact. It is easy to comprehend what sleep is if one asks oneself that question as one is trying to get to sleep. Human sleep can be defined as an altered state in which there is impaired conscious awareness of the external world with different controls, rhythms, emotions, and dreams. It is a transient natural, periodic, physiologic phenomenon which is reversible, thus differentiating it from irreversible coma and death. Consciousness requires two components: awareness (function of cerebral cortex) and arousal (function of ascending reticular activating system). Sleep differs from unconscious state or coma (a pathological state) in the following manner: Sleep besides being a reversible physiological state also shows differences from coma in terms of brain metabolism and circulation which show marked depression and impairment in coma but slight alterations in sleep. Persistent vegetative state, minimally conscious state, and coma are distinctly different from sleep state although superficially may resemble those other states (see Chap. 43). Modern sleep researchers define sleep on the basis of both behavior of the person while asleep and the related physiologic changes that occur to the waking brain’s electrical rhythm in sleep [35–38]. The behavioral criteria (Table 2.1) include lack of mobility or slight mobility, closed eyes, a characteristic species-specific sleeping posture, reduced response to external stimulation, quiescence, increased reaction time, elevated arousal threshold, impaired cognitive function, and a reversible unconscious state. Sleep is an active anabolic state (e.g., promoting growth, stimulating immune system) and is observed in all mammals, birds, reptiles, amphibians, and fish. The physiologic criteria (see Sleep Architecture and Sleep Profile) based on the findings from electroencephalography (EEG), electrooculography (EOG), and electromyography (EMG) as well as other physiological changes in ventilation and circulation. While trying to define the process of falling asleep, we must differentiate sleepiness from fatigue or tiredness. Fatigue (see also Chap. 41) can be defined as a state of sustained lack of energy coupled with a lack of motivation and drive but does not require the behavioral criteria of sleepiness such as heaviness and drooping of the eyelids, sagging or nodding of the head, yawning, and an ability to nap given the opportunity to fall asleep. On the other hand, fatigue is often a secondary consequence of sleepiness.
Table 2.1
Behavioral criteria of wakefulness and sleep
Criteria | Awake | Non-rapid eye movement sleep | Rapid eye movement sleep |
---|---|---|---|
Posture | Erect, sitting, or recumbent | Recumbent | Recumbent |
Mobility | Normal | Slightly reduced or immobile; postural shifts | Moderately reduced or immobile; myoclonic jerks |
Response to stimulation | Normal | Mildly to moderately reduced | Moderately reduced to no response |
Level of alertness | Alert | Unconscious but reversible | Unconscious but reversible |
Eyelids | Open | Closed | Closed |
Eye movements | Waking eye movements | Slow rolling eye movements | Rapid eye movements |
The Moment of Sleep Onset and Offset
There is no exact moment of sleep onset—there are gradual changes in many behavioral and physiological characteristics including EEG rhythms, cognition, and mental processing including reaction time. Sleepiness begins at sleep onset even before reaching stage 1 NREM sleep (as defined later) with heaviness and drooping of the eyelids; clouding of the sensorium; and inability to see, hear, or perceive things in a rational or logical manner. At this point, an individual trying to get to sleep is now entering into another world in which the person has no control and the brain cannot respond logically and adequately. This is the stage coined by McDonald Critchley as the “Pre-Dormitum” [39] who also mentioned that Gowers used “sleepening” for this stage, and this is opposite of “awakening.” Slow rolling eye movements (SEMs) begin at sleep onset and continue through stage 1 NREM sleep. At sleep onset, there is a progressive decline in thinking process, and sometimes there may be hypnagogic imagery and hypnic myoclonus [40].
Similar to sleep onset, the moment of awakening or sleep offset (post-dormitum) is also a gradual process from the fully established sleep stages. This period is sometimes described as manifesting sleep inertia or sleep drunkenness. There is a gradual return to a state of alertness or wakefulness.
Sleep Architecture and Sleep Profile
Based on three physiologic measurements (EEG, EOG, and EMG), sleep is divided into two states [41] with independent functions and controls: NREM sleep and REM sleep. Table 2.2 lists the physiologic criteria of wakefulness and sleep and Table 2.3 summaries NREM and REM sleep states. In an ideal situation (which may not be seen in all normal individuals), NREM and REM alternate in a cyclic manner, each cycle lasting on an average from 90 to 110 mins. During a normal sleep period in adults, 4–6 such cycles are noted. The first two cycles are dominated by slow-wave sleep (SWS) (R–K stages 3 and 4 NREM and AASM stage N3 sleep); subsequent cycles contain less SWS, and sometimes SWS does not occur at all. In contrast, the REM sleep cycle increases from the first to the last cycle, and the longest REM sleep episode toward the end of the night may last for an hour. Thus, in human adult sleep, the first third is dominated by the SWS and the last third is dominated by REM sleep. It is important to be aware of these facts because certain abnormal motor activities are characteristically associated with SWS and REM sleep.
Table 2.2
Physiologic criteria of wakefulness and sleep
Criteria | Awake | Non-rapid eye movement sleep | Rapid eye movement sleep |
---|---|---|---|
Electroencephalography | Alpha waves; desynchronized | Synchronized | Theta or saw tooth waves; desynchronized |
Electromyography (muscle tone) | Normal | Mildly reduced | Moderately to severely reduced or absent |
Electrooculography | Waking eye movements | Slow rolling eye movements | Rapid eye movements |
Table 2.3
Summary of non-rapid eye movement and rapid eye movement sleep states
Sleep state | Sleep time (%) |
NREM sleep | 75–80 |
N1 | 3–8 |
N2 | 45–55 |
N3 | 15–23 |
REM sleep | 20–25 |
Tonic stage | – |
Phasic stage | – |
Non-rapid Eye Movement (NREM) Sleep—NREM sleep accounts for 75–80 % of sleep time in an adult human. According to R–K scoring manual, [14] NREM sleep is further divided into four stages (stages 1–4), and according to the recent AASM scoring manual, [15] this is subdivided into three stages (N1, N2, and N3), primarily on the basis of EEG criteria. Stage 1 NREM (N1) sleep occupies 3–8 % of sleep time; stage 2 (N2) comprises 45–55 % of sleep time; and stage N3 or SWS makes up 15–23 % of total sleep time. The dominant rhythm during adult human wakefulness consists of the alpha rhythm (8–13 Hz) noted predominantly in the posterior region intermixed with small amount of beta rhythm (>13 Hz) seen mainly in the anterior head regions (Fig. 24.1). This state called stage W may be accompanied by conjugate waking eye movements (WEMs) which may comprise vertical, horizontal, oblique, slow, or fast eye movements. In stage 1 NREM sleep (stage N1), alpha rhythm diminishes to less than 50 % in an epoch (i.e., a 30-second segment of the polysomnographic tracing with the monitor screen speed of 10 mm/s) intermixed with slower theta rhythms (4–7 Hz) and beta waves (Fig. 24.2). Electromyographic activity decreases slightly, and slow eye movements (SEMs) appear. Toward the end of this stage, vertex sharp waves are noted. Stage 2 NREM (stage N2) begins after approximately 10–12 min of stage 1. Sleep spindles (11–16 Hz, mostly 12–14 Hz) and K-complexes intermixed with vertex sharp waves herald the onset of stage N2 sleep (Fig. 24.3). Sleep spindles could be divided into two types: fast spindles (13–15 Hz) seen predominantly in the centroparietal region and slow spindles (11 ≤ 13 Hz) seen mostly in the frontal region. EEG at this stage also shows theta waves and slow waves (0.5–2 Hz) that occupy less than 20 % of the epoch. After about 30–60 mins of stage 2 NREM sleep (stage N2), stage 3 sleep begins and slow waves comprise 20–100 % of the epoch (Fig. 24.4). As stated above, R–K stages 3 and 4 NREM are grouped together as SWS and are replaced by stage N3 in the new AASM scoring manual. Body movements often are recorded as artifacts in PSG recordings toward the end of SWS as sleep is lightening. Stage N3 is briefly interrupted by stage 2 NREM (stage N2), which is followed by the first REM sleep approximately 60–90 mins after sleep onset.
Rapid Eye Movement (REM) Sleep—REM sleep accounts for 20–25 % of total sleep time. Based on EEG, EMG, and EOG characteristics, REM can be subdivided into two stages (tonic and phasic). This subdivision is not recognized in the recent AASM scoring manual. A desynchronized EEG, hypotonia or atonia of major muscle groups with the exception of the diaphragm and the oculomotor muscles, and depression of monosynaptic and polysynaptic reflexes are characteristics of tonic REM sleep. This tonic stage persists throughout the REM sleep, whereas the phasic stage is discontinuous and superimposed on the tonic stage. Phasic REM sleep is characterized by bursts of rapid eye movements (REMs) in all directions, in singlets or clusters. Phasic swings in blood pressure and heart rate, irregular respiration, spontaneous middle ear muscle activity (MEMA), periorbital integrated potentials (PIPs) [42], and myoclonic twitching of the facial and limb muscle and tongue movements [43] are all characteristics of phasic REM sleep (Fig. 2.2). These and other phasic phenomena of REM sleep are listed in Box 2.1. A few periods of apnea or hypopnea may occur during REM sleep. Electroencephalographic tracing during REM sleep consists of low-amplitude fast pattern in the beta frequency range mixed with a small amount of theta rhythms, some of which may have a “saw tooth” appearance (Fig. 2.2). Saw tooth waves are trains of sharply contoured, often serrated, 2–6 Hz waves usually with rapid ascent and slow descent seen maximally over the central regions and are thought to be the gateway to REM sleep, often preceding a burst of REMs. PIPs are seen during REMs (Fig. 2.2) but not all REMs are accompanied by PIPs. During REM sleep, there may be some intermittent intrusions of alpha rhythms in the EEG lasting for a few seconds. The first REM sleep lasts only a few minutes. Sleep then progresses to stage 2 followed by stage N3 before the second REM sleep begins.


Fig. 2.2
Polysomnographic recording shows rapid eye movement (REM) sleep in an adult. EEG (top 8 channels) shows mixed-frequency theta, low-amplitude beta, and a small amount of alpha activity. Note the characteristic sawtooth waves (seen prominently in channels, 1, 2, 5, and 6 from the top) of REM sleep preceding bursts of REMs in the electrooculograms (E1-M1; E2-M2). Chin EMG shows marked hypotonia, whereas TIB and GAST EMG channels show very low-amplitude phasic myoclonic bursts. Reprinted from Chap. 2, 3rd edition
Box 2.1. Lists of Phasic Events of REM Sleep
Rapid eye movements (REMs)
Phasic muscle bursts (myoclonic or transient muscle bursts)
Phasic tongue movements
Periorbital integrated potentials (PIPS)
Middle ear muscle activity (MEMA)
Ponto-geniculo-occipital waves (PGO or P waves) [rats and cats; also reported in human during corticography while performing epilepsy surgery]
Phasic alterations of breathing (brady-tachypnea)
Phasic swings of BP (up and down)
Phasic swings of heart rate (brady-tachyarrhythmia)
Hippocampal theta waves (rhythms) [animal study]
Saw tooth waves in the EEG
Alpha bursts in the EEG during REM sleep
Phasic increase in brain intracellular firing rates during REM sleep
Penile erections in men and clitoral tumescence in women in REM sleep
Phasic increase in myocardial oxygen demand in REM sleep
Phasic vivid dreaming in REM sleep
Phasic suppression of REM muscle atonia
Phasic pupillary dilation and constriction
Phasic fractionations of diaphragmatic activity (pauses of 40–80 ms occurring in clusters) correlating with PGO waves which are phasic events of REM sleep
In summary, during normal sleep in adults, there is an orderly progression from wakefulness to sleep onset to NREM and then to REM sleep. Relaxed wakefulness is characterized by behavioral state of quietness and physiological state of alpha and beta frequency in the EEG, WEMs, and increased muscle tone. NREM sleep is characterized by progressively decreased responsiveness to external stimulation accompanied by SEMs, followed by electroencephalographic slow-wave activity associated with spindle and K-complexes, and decreased muscle tone. REMs markedly reduced or absent muscle tone and low voltage fast electroencephalographic activity mixed with distinctive saw tooth waves and PIPs characterize REM sleep.
The R–K system addresses normal adult sleep and macrostructure of sleep. In patients with sleep disorders such as sleep apnea, parasomnias, or sleep-related seizures, it may be difficult to score sleep according to R–K criteria. Furthermore, the R–K staging system does not address the microstructure of sleep. The recent AASM sleep scoring criteria with a brief reference to R and K system have been outlined in Chap. 24. The macrostructure of sleep is summarized in Box 2.2. There are several endogenous and exogenous factors, which will modify sleep macrostructure (Box 2.3).
Box 2.2: Sleep Macrostructure
Sleep states and stages |
Sleep cycles |
Sleep latency |
Sleep efficiency (the ratio of total sleep time to total time in bed expressed as a percentage) |
Wake after sleep onset |
Box 2.3: Factors Modifying Sleep
Macrostructure
Exogenous
Noise
Exercise
Ambient temperature
Drugs and alcohol
Endogenous
Age
Prior sleep–wakefulness
Circadian phase
Sleep pathologies
Sleep Microstructure—Sleep microstructure includes momentary dynamic phenomena such as arousals, which have been operationally defined by the Task Force of the American Sleep Disorders Association (now called American Academy of Sleep Medicine) [44] which has remained essentially unchanged in the recent AASM scoring manual, [15] and the cyclic alternating pattern (CAP), which has been defined and described in various publications by Terzano and co-investigators [45–47]. Other components of microstructure include K-complexes and sleep spindles (Box 2.4).
Box 2.4: Sleep Microstructure
Arousals |
Cyclic alternating pattern |
Sleep spindles |
K-complexes |
Arousals are transient phenomena resulting in fragmented sleep without behavioral awakening. An arousal is scored during sleep stages N1, N2, N3 (or REM sleep) if there is an abrupt shift in EEG frequency lasting from 3 to 14 s (Fig. 2.3) and including alpha, beta, or theta activities but not spindles or delta waves. Before an arousal can be scored, the subject must be asleep for 10 consecutive seconds. In REM sleep, arousals are scored only when accompanied by concurrent increase in segmental EMG amplitude. K-complexes, delta waves, artifacts, and only increased segmental EMG activities are not counted as arousals unless these are accompanied by EEG frequency shifts. Arousals can be expressed as number per hour of sleep (an arousal index), and up to 10 arousal index can be considered normal.


Fig. 2.3
Polysomnographic recording shows two brief periods of arousals out of stage N2 sleep in the left- and right-hand segments of the recording, lasting for 5.58 and 6.40 s and separated by more than 10 s of sleep. Note delta waves followed by approximately 10-Hz alpha activities during brief arousals. Reprinted from Chap. 2, 3rd edition
The Cyclic Alternating Pattern—The CAP (Fig. 2.4) indicates sleep instability, whereas frequent arousals with or without stage shifts signify sleep fragmentation [47]. A fragmentation index (number of arousals and stage shifts per hour) can also be calculated to indicate sleep instability. Sleep microstructure is best understood by the CAP, wherein an EEG pattern that repeats in a cyclical manner is noted mainly during NREM sleep. This is a promising technique in evaluating both normal and abnormal sleep, as well as in understanding the neurophysiologic and neurochemical basis of sleep. A CAP cycle [48] consists of an unstable phase (Phase A) and relatively stable phase (Phase B) each lasting between 2 and 60 s. Phase A of CAP is marked by an increase in EEG potentials with contributions from both synchronous high amplitude and slow, and desynchronized fast rhythms in the EEG recording standing out from a relatively low-amplitude slow background. The A phase is associated with an increase in heart rate, respiration, blood pressure, and muscle tone. CAP rate (total CAP time during NREM sleep) and arousals both increase in older individuals and in a variety of sleep disorders including both diurnal and nocturnal movement disorders. Non-CAP (sleep period without CAP) is thought to indicate a state of sustained stability.


Fig. 2.4
Polysomnographic recording showing consecutive stretches of non-cyclic alternating pattern (non-CAP) (top), cyclic alternating pattern (CAP) (middle), and non-CAP (bottom). The CAP sequence, confined between the two black arrows, shows three phase As and two phase Bs, which illustrate the minimal requirements for the definition of a CAP sequence (at least three phase As in succession). Electroencephalographic derivation (top 5 channels in top panel): FP2-F4, F4-C4, C4-P4, P4-02, and C4-A1. Similar electroencephalographic derivation is used for the middle and lower panels. From Terzano et al. [48]. Reprinted from Chap. 2, 3rd edition
In summary, sleep macrostructure is based on cyclic patterns of NREM and REM states, whereas sleep microstructure mainly consists of arousals, periods of CAP, and periods without CAP. An understanding of sleep macrostructure and microstructure is important because emergence of abnormal motor activity during sleep may be related to disturbed macrostructure and microstructure of sleep.
The Ontogeny of Sleep
Evolution of EEG and sleep states (see also Chap. 52) from the fetus, preterm, and term infant, early childhood, adolescence to adulthood follows in an orderly manner depending upon the maturation of the central nervous system (CNS) [49–52]. Neurological, environmental, and genetic factors as well as comorbid medical or neurological conditions will have significant effects on such ontogenetic changes. Sleep requirements change dramatically from infancy to old age. Newborns have a polyphasic sleep pattern, with 16 h of sleep per day. This sleep requirement decreases to approximately 10 h per day by 3–5 years of age. In preschool children, sleep assumes a biphasic pattern. Adults exhibit a monophasic sleep pattern, with an average duration from 7.5 to 8 h per night. This returns to a biphasic pattern in old age.
Upon falling asleep, a newborn baby goes immediately into REM sleep, or active sleep, which is accompanied by restless movements of the arms, legs, and facial muscles. In premature babies, it is often difficult to differentiate REM sleep from wakefulness. Sleep spindles appear from 6 to 8 weeks and are well formed by 3 months (may be asynchronous during the first year and by age 2 are synchronous). K-complexes are seen at 6 months but begin to appear at over 4 months. Hypnagogic hypersynchrony characterized by transient bursts of high amplitude waves in the slower frequencies appears at 5–6 months and is prominent at one year. By 3 months of age, the NREM/REM cyclic pattern of adult sleep is established. However, the REM/NREM cycle duration is shorter in infants, lasting for approximately 45–50 mins and increasing to 60–70 mins by 5–10 years and the normal adult cyclic pattern of 90–100 mins by age of 10 years. A weak circadian rhythm is probably present at birth, but by 6–8 weeks, it is established. Gradually, the nighttime sleep increases and daytime sleep decreases and the number of naps decreases. By 8 months, the majority take two naps (late morning and early afternoon). The first 3 months is a critical period of CNS reorganization, and striking changes occur in many physiological responses. In newborns, total sleep time is about 16 h. The total sleep time decreases to 14 h at 4 months and to 13 h at 6–8 months. By 3–6 months, major concentration of sleep occurs at night.
Sleep onset in the newborn occurs through REM sleep. During the first three months, sleep onset REM begins to change. In the newborn, active sleep (REM) occurs 50 % of the total sleep time. This decreases during the first 6 months of age. By 9–12 months, REM sleep occupies 30–35 % of sleep and by 5–6 years, REM sleep decreases to adult levels of 20–25 %. The napping frequency continues to decline, and by age 4–6 years, most children stop daytime naps. Nighttime sleep patterns become regular gradually, and by age 6, nighttime sleep is consolidated with few awakenings. At 9–10 years of age, most children sleep for 10 h at night. Pre-adolescents are highly alert during the day with multiple sleep latency test (MSLT) showing a mean sleep latency of 17–18 min. In summary, the multiphasic sleep pattern of newborns and infants gradually changes to biphasic sleep in preschool children, and finally to monophasic sleep in adults [49, 50]. Sleep reverts to biphasic pattern in the elderly.
There are two other important changes that occur in the sleep pattern in old age: repeated awakenings throughout the night, including early morning awakenings that prematurely terminate the night sleep, and a marked reduction of the amplitude of the slow waves resulting in a decreased percentage of slow-wave sleep (SWS) in this age group. The percentage of REM sleep in normal elderly individuals remains relatively constant, and the total duration of sleep time within 24 h is also no different from that of young adults; however, elderly individuals often nap during the daytime compensating for lost sleep during the night. Figure 2.5 shows schematically the evolution of sleep step distribution in newborns, infants, children, adults, and elderly adults. Night sleep histograms of children, young adults, and of elderly adults are shown in Fig. 2.6.



Fig. 2.5
Graphic representation of percentages of REM and NREM sleep at different ages. Note the dramatic changes in REM sleep in the early years. Adapted from Roffwarg et al. [49]. Reprinted from Chap. 2, 3rd edition

Fig. 2.6
Night sleep histogram from a child, a young adult, and an elderly person. Note significant reduction of stage 4 NREM sleep as one grows older. From Kales and Kales [238]. Reprinted from Chap. 2, 3rd edition
The significant evolutionary changes in the respiratory and cardiovascular functions [51, 53]. Respiratory controllers are immature and not fully developed at birth. Respiratory mechanics and upper airway anatomy are different in newborns than in adults contributing to breathing problems, particularly during sleep in newborn infants. Brief periods of respiratory pauses or apneas lasting for 3 s or longer, periodic breathing and irregular breathing may be noted in newborns, especially during active (REM) sleep. According to the National Institutes of Health, Consensus Development Conference on Infantile Apnea, [54] the term periodic breathing refers to respiratory pauses of at least 3 s with less than 20 s of normal breathing in between the pauses. Cheyne–Stokes breathing is periodic waxing and waning of respiration accompanied by central apneas and may be noted in preterm infants. Periodic breathing and occasional central apneas of up to 15 s duration in newborns may be noted without any clinical relevance unless accompanied by bradycardia or cyanosis. These breathing events gradually disappear during the first few weeks of life. The respiratory rate also gradually slows during the first few years of life. Another important finding in the newborn, particularly during active sleep is paradoxical inward motion of the rib cage. This occurs because of high compliance of the rib cage in newborns, circular rather than elliptical thorax and decreased tone of the intercostal and accessory muscles of respiration. This paradoxical breathing causes hypoxia and reduced diaphragmatic efficiency. Similar breathing in adults occurs during diaphragmatic weakness. At term, posterior cricoarytenoyd muscles which assist in maintaining upper airway patency are not adequately coordinated with diaphragmatic activity causing a few periods of obstructive apneas especially during active sleep. Ventilatory responses to hypoxia are also different in newborns than in adults. In quiet sleep, hypoxia stimulates breathing as in adults, but in active sleep after the initial period of stimulation, there is ventilatory depression. Laryngeal stimulation in adults causes arousal, but in infants, this may cause an apnea. Breathing becomes regular, and the respiratory control is adequately developed by the end of the first year. Changes in cardiovascular function indicate changes in the autonomic nervous system (ANS) during infancy and early childhood. There is greater parasympathetic control for children than infants (as assessed by heart rate low-frequency [LF] and high-frequency [HF] analysis (see also Chap. 11): 0.15–0.5 Hz [HF] indicates parasympathetic and 0.04–0.15 Hz [LF] indicates sympathetic activity). The better parasympathetic control for children than infants indicates ANS maturity. Respiratory heart rate modulation is variable in newborns as assessed by LF and HF heart rate spectral analysis. In active sleep, most of the power is in LF. In older infants and children, there is significant respiratory heart rate modulation termed normal sinus arrhythmia. Respiratory rate during quiet sleep decreases, and the respiratory variability decreases with age.
Sleep Habits
Sleep specialists sometimes divide people into two groups: “evening types” (owls) and “morning types” (larks). The morning types wake up early feeling rested and refreshed and work efficiently in the morning. These people get tired and go to bed early in the evening. In contrast, evening types have difficulty getting up early and they feel tired in the morning; they feel fresh and energetic toward the end of the day. These people perform best in the evening. They go to sleep late at night and wake up late in the morning. The body temperature rhythm takes on different curves in these two types of people. The body temperature reaches the evening peak an hour earlier in morning types than in evening types. What determines a morning or evening type is not known, but heredity may play a role. Katzenberg et al. [55] using the 19-item Horne–Ostberg questionnaire to determine morningness/eveningness in human circadian rhythms discovered a clock gene polymorphism associated with human diurnal preference. One of two H clock alleles (3111C) is associated with eveningness. These findings have been contradicted by later studies [56]. There is a third type (intermediate type) not conforming to either morning or evening type [57].
Sleep Need and Requirement
Sleep requirement or sleep need is defined as the optimum amount of sleep required to remain alert and fully awake and to function adequately throughout the day. Sleep debt is defined as the difference between the ideal sleep requirement and the actual duration of sleep obtained. There are two divergent views: Harrison and Horner [58] concluded that society is not sleep deprived, whereas Bonnet and Arand [59] stated that modern society is chronically sleep deprived. Between 1910 and 1963, there was a mean reduction of 1.5 h of sleep in adolescents aged 8–17 years [60]. However, there may be a significant sampling error in this survey. A study by Bliwise and associates [61] in healthy adults aged 50–65 years showed a reduction of about one hour of sleep between 1959 and 1980 surveys.
It has been traditionally stated that women need more sleep than men, but this has been questioned in a recent field study [62]. There is also a general perception based on questionnaire, actigraphy, and PSG studies that sleep duration decreases with increasing age [63, 64]. This relationship, however, remains controversial. Older adults take naps, and these naps may compensate for nighttime sleep duration curtailment. Sleep is regulated by homeostasis (increasing sleep drive during continued wakefulness) and circadian factors (the sleep drive varying with time of the day). The influence of these factors is reduced in older adults but is still present. Older adults are also phase advanced (e.g., internal clock set earlier yielding early bedtime and early morning awakenings).
Sleep requirement for an average adult is approximately 7½–8 h regardless of environmental or cultural differences [65]. Most probably whether a person is a long or a short sleeper and sleep need are determined by heredity rather than by different personality traits or other psychological factors. Sleep behavior is regulated by genetics. Sleep duration is influenced by the gene DEC2, mutation of which in some people may reduce sleep duration. Social (e.g., occupational) or biological (e.g., illness) factors may also play a role. Sleep need is genetically determined, but its physiologic mechanism is unknown. Slow-wave activity (SWA) in sleep EEG depends on sleep need and homeostatic drive. Adenosine, a peptide, seems to have a direct role in homeostasis. Prolonged wakefulness causes increased accumulation of adenosine which decreases during sleep. SWA increases after sleep loss. Long sleepers spend more time asleep but have less SWS [66] and more stage 2 NREM sleep than do short sleepers [67]. An early important epidemiological study [68] found that the chances of death from coronary arterial disease, cancer, or stroke are greater for adults who sleep less than 4 h or more than 9 h when compared to those who sleep an average of 7½–8 h. There have been some more recent studies by Kripke et al. [69] and others [70–72] confirming these observations. In later studies, other factors such as sleeping medications may have confounded these issues. There is no clear-cut conclusion yet.
There is controversy whether a person can extend sleep beyond the average requirement. Early studies by Taub and Berger [73, 74] showed that sleep extension beyond the average hours may cause exhaustion and irritability with detriment of sleep efficiency. The authors refer to this as the “Rip Van Winkle” effect [74]. Sleep extension studies in the past reported conflicting results regarding MSLT scores, vigilance, and mood ratings [75]. When subjects are challenged to maximum sleep extension, there is substantial improvement in daytime alertness, reaction time, and mood [75]. Most individuals carry a large sleep debt and as extra sleep reduces carryover sleep debt, it is then no longer possible to obtain extra sleep [76].
Sleep and Dreams
Freud [77] called dreams the “Royal Road to the Unconscious” in his seminal book, The Interpretation of Dreams, published in 1900. The Freudian theory postulated that repressed feelings are psychologically suppressed or hidden in the unconscious mind and often manifested in dreams. Sometimes those feelings are expressed as mental disorders or other psychologically determined physical ailments, according to this psychoanalytic theory. In Freud’s view, most of the repressed feelings are determined by repressed sexual desires and appear in dreams or symbols representing sexual organs. In recent times, Freudian theory has fallen in disrepute. The modern sleep scientists try to interpret dreams in anatomic and physiologic terms. Nevertheless, we still cannot precisely define what is “dream” and why we dream. The field of dream research took a new direction since the existence of REM sleep was first observed by Aserinsky and Kleitman [11] in 1953. It is postulated that approximately 80 % of dreams occur during REM sleep and 20 % occur during NREM sleep [78]. It is easier to recall REM dreams than NREM dreams. It is also easier to recall dreams if awakened immediately after the onset of REM dreams rather than trying to remember them the next morning upon getting out of bed. REM dreams are often vivid, highly emotionally charged, unrealistic, complex, and bizarre. In contrast, dream recall which sometimes may partially occur upon awakening from the NREM dream state is more realistic. People are generally oriented when awakening from REM sleep but are somewhat disoriented and confused when awakened from NREM sleep. Most of all, dreams take place in natural color, rather than black and white. In our dreams, we employ all five senses. In general, we use mostly the visual sensations, followed by auditory sensation. Tactile, smell, and taste sensation are represented least. Dreams can be pleasant, unpleasant, frightening, or sad. They generally reflect one’s day-to-day activities. Fear, anxiety, and apprehension are incorporated into our dreams. In addition, stressful events of past or present may occupy our dreams. The dream scenes or events are rarely rational but often occur in an irrational manner with rapid change of scene, place, or people or a bizarre mixture of these elements. Sometimes, lucid dreams may arise in which the dreamer seems to realize vividly that he or she is actually dreaming [79].
The neurobiologic significance of dreams remains unknown. Sleep scientists try to explain dreams in the terms of anatomical and physiological interpretation of REM sleep. During this state, the synapses, nerve cells, and nerve fibers connecting various groups of nerve cells in the brain become activated. This activation begins in the brainstem, and the cerebral hemisphere then synthesizes these signals and creates colorful or black and white images giving rise to dreams. Similarly, signals sometimes become converted into auditory, tactile, or other sensations to cause dream imagery. Why the nerve circuits are stimulated to cause dreaming is not clearly understood. Some suggestions to explain significance of dreams include activation of the neural networks in the brain, [80] restructuring and reinterpretation of data stored in memory [81]. This resembles Jouvet’s hypothesis of a relationship between REM sleep and recently acquired information [82]. According to molecular biologist and Nobel laureate Crick and Mitchison [83], the function of dreaming is to unlearn, that is, to remove unnecessary and useless information from the brain. Some have also suggested that memory consolidation takes place during the dream stage of sleep (see Chap. 13). In addition, stories abound regarding artists, writers, and scientists who develop innovative ideas about their art, literature, and scientific projects during dreams. Dream-enacting behavior associated with abnormal movement during sleep (REM behavior disorder) and frightening dreams called nightmares or dream anxiety attacks constitute two important REM parasomnias.
Phylogeny of Sleep
Studies have been conducted to find out whether, like humans, other mammals have sleep stages [1, 84–87]. The EEG recordings of mammals show similarities to those of humans. Both REM and NREM sleep stages can be differentiated by EEG, EMG, and EOG in animals. Dolphins and whales are the only groups of mammals showing no REM sleep on recordings [1, 88, 89]. Although initially thought to have no REM sleep, [90] some recent evidence suggests that Australian spiny anteaters (the monotremes, or egg-laying mammals, echidna) do have REM sleep [91–93]. Siegel and colleagues [94] suggest that the echidna combines REM and NREM aspects of sleep in a single sleep state. These authors further suggest that REM and NREM sleep evolved from a single, phylogenetically older sleep state.
Like humans, mammals can be short or long sleepers. There are considerable similarities between sleep duration and length of sleep cycles in small and large animals. Small animals with a high metabolic rate have a shorter life span and sleep longer than larger animals with lower metabolic rates [95]. Smaller animals also have a shorter REM–NREM cycle than larger animals. The larger the animal, the less it sleeps; e.g., elephants sleep 4–5 h and giraffes sleep even less than that.
A striking finding in dolphins is that during sleep, half the brain shows the characteristic EEG features of sleep while the other half shows the EEG features of waking [96]. Each sleep episode lasts approximately 30–60 mins; then, the roles of the two halves of the brain reverse. Similar unihemispheric sleep episodes with eye closure contralateral to the sleeping hemisphere are known to occur in the pilot whale and porpoise [35]. It is of interest to note that there are indications from computerized coherence analysis of such interhemispheric SWS asymmetry even in human with severe OSA during apneic arousals [97, 98].
Both vertebrates and invertebrates display sleep and wakefulness [99]. Most animals show the basic rest–activity rhythms during a 24-h period. There is behavioral and EEG evidence of sleep in birds but the avian REM–NREM cycles are very short [99, 100]. Although birds are thought to have evolved from reptiles, the question of the existence of REM sleep in reptiles remains somewhat controversial [99]. The absence of REM sleep in reptiles and the presence of NREM and REM sleep in both birds and mammals would be in favor of REM sleep being a more recent development in the phylogenetic history of land-dwelling organisms [99]. Sleep has also been noted in invertebrates, such as insects, scorpions, and worms, based on behavioral criteria [100].
In conclusion, the purpose of studying the phylogeny of sleep is to understand the neurophysiologic and neuroanatomic correlates of sleep as one ascends the ladder of phylogeny from inframammalian to mammalian species. Tobler [35] concluded that sleep is homeostatically regulated, in a strikingly similar manner, in a broad range of mammalian species. These similarities in sleep and its regulation among mammals suggest common underlying mechanisms that have been preserved in the evolutionary process.
Circadian Sleep–Wake Rhythm
The existence of circadian rhythms has been recognized since the eighteenth century, when the French astronomer de Mairan [101] noted a 24-hr rhythm in heliotrope plants. The plants closed their leaves at sunset and opened them at sunrise, even when they were kept in darkness, shielded from direct sunlight. The discovery of 24-h rhythm in the movements of plant leaves suggested to de Mairan an “internal clock” in the plant. Experiments by chronobiologists Pittendrigh [102] and Aschoff [103] in 1960 clearly proved the existence of 24-h rhythms in animals.
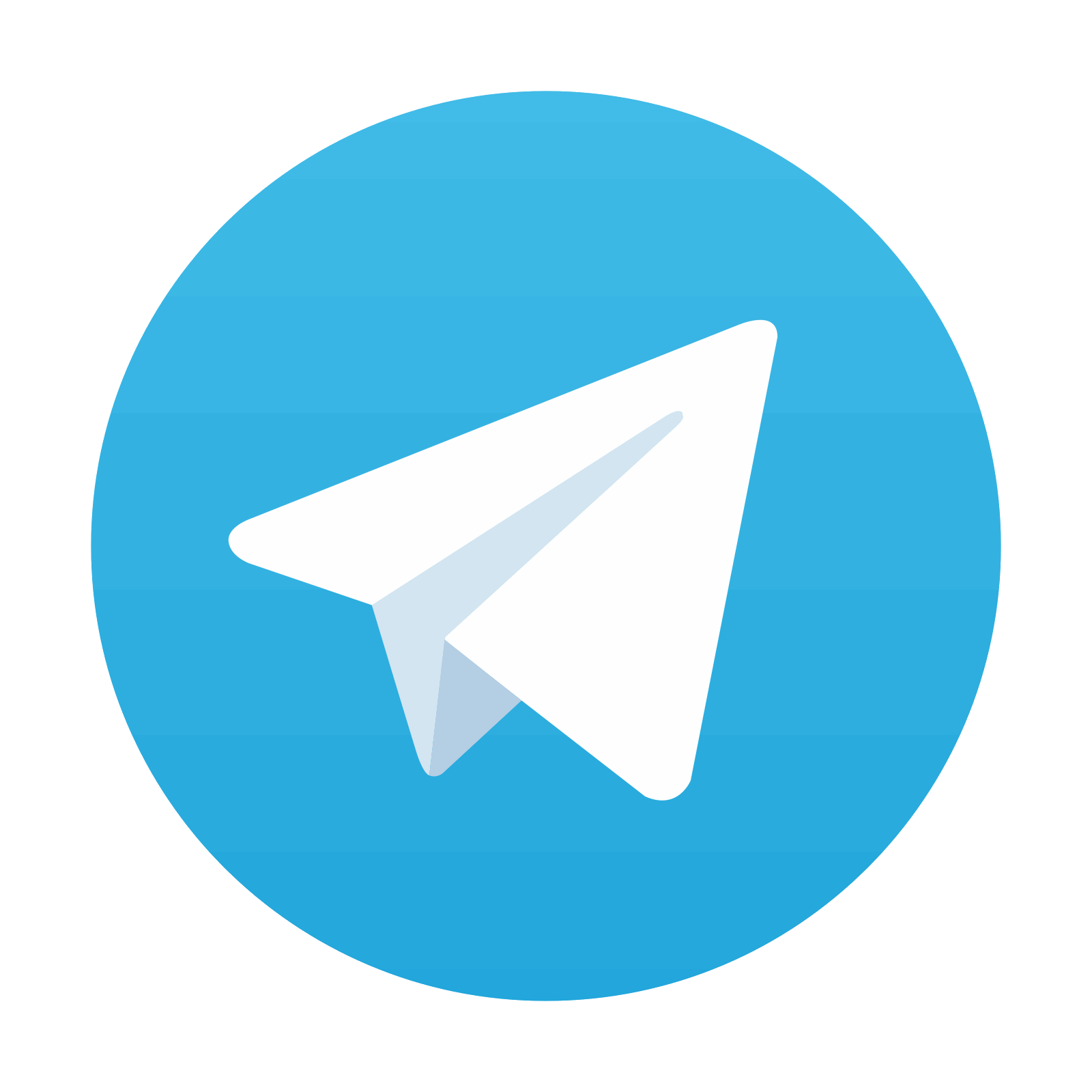
Stay updated, free articles. Join our Telegram channel

Full access? Get Clinical Tree
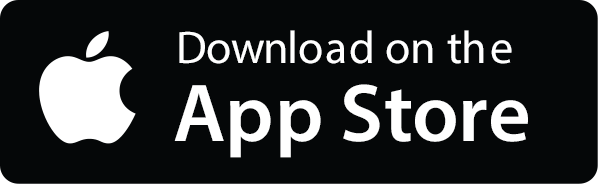
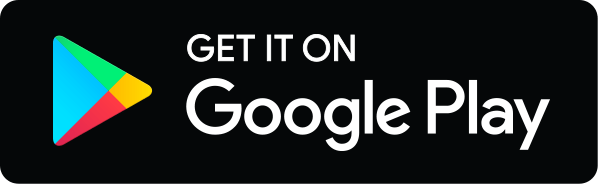