Oxygen Administration in the Sleep Center
Thomas Russell
LEARNING OBJECTIVES
On completion of this chapter, the reader should be able to:
1. List the various storage methods for oxygen.
2. Regulate and control the flow of oxygen.
3. Choose an appropriate oxygen administration device.
4. Describe the limitations and inaccuracies of oxygen delivery.
5. List variables affecting oxygen therapy with continuous positive airway pressure/bilevel positive airway pressure.
6. Recognize the potential for creating increased nasal resistance.
KEY TERMS
Hypoxia
Hypoxemia
FiO2
lpm
psi
Delivered FiO2
Actual FiO2
Entrainment
SpO2%
Ambient or atmospheric air comprises nearly 21% oxygen gas. Oxygen is tasteless, colorless, odorless, and transparent. By itself, oxygen is not flammable but vigorously accelerates combustion. Oxygen is an elemental gas required for life. Various respiratory and/or cardiac problems require augmentation of ambient oxygen concentrations.
This chapter discusses the techniques and concerns of oxygen therapy, specifically, how supplemental oxygen is delivered in the sleep center environment. Common delivery devices and their inherent limitations are discussed.
Effective oxygen administration demands a technologist familiar with the physiology of respiration, ventilation, and gas exchange. Oxygen is considered a drug and therefore administered in accordance with institution policy and procedure. Typically, oxygen therapy is prescribed by a physician and administered by appropriately credentialed personnel. The reader is encouraged to review his or her particular workplace procedures, pertinent policies, and licensure.
Oxygen is one of the most commonly used drugs in hospitals. Interestingly, given the incidence and severity of hypoxemia encountered in the sleep center, the use of oxygen is relatively uncommon. Because hypoxemia occurring during sleep is most often caused by transient upper airway obstruction and/or hypoventilation, treatment is directed toward maintaining upper airway patency using continuous positive airway pressure (CPAP) and/or augmenting ventilation using bilevel positive airway pressure (BPAP). Supplemental oxygen is usually not required and is rarely considered a “stand-alone” therapy (1).
OXYGEN STORAGE AND DISTRIBUTION
The use of medical grade oxygen requires a method of storage and means of delivery. Oxygen is commercially packaged as a compressed gas or in its liquid form. Alternatively, oxygen can be separated and concentrated from room air using an “oxygen concentrator.”
Manufactured oxygen is most often void of any humidity and is termed anhydrous, meaning without water. The gas is completely dry, which can irritate the upper airway mucous membranes. This is more of an issue with higher oxygen flows not often encountered in the sleep center.
Compressed Oxygen Gas
Medical gas cylinders (tanks) have been in use since 1888. Several medical/anesthetic gases, including pure (99%) oxygen, are packaged in cylinders, which are manufactured of seamless, high-quality, finely tempered steel. For safety, cylinders are color coded. Oxygen tanks are colored white (or green). White is the international color code for oxygen. Despite color coding, the contents of a cylinder should be verified by its tag/label. If there is no tag, do not use the cylinder.
Numerous federal and industrial regulatory bodies govern cylinder manufacturing specifications, labeling, transportation, storage, and handling of medical gases.
Oxygen cylinders are available in different sizes that contain various amounts of gas. Cylinder size is designated by single letters. For example, popular oxygen tanks are termed H (large) size and E (small) size. E-sized tanks are usually used for patient transport or in emergencies. H-sized tanks measure over 4½ ft (1.37 m) tall and are used more for stationary/long-term (bedside) applications. Oxygen cylinders are an expensive and bulky form of packaging for a relatively inexpensive substance.
Cylinders contain much more gas than their unpressurized internal volume. By pressurizing the gas into cylinders, H and E cylinders when full contain 244 ft3 (74.4 m3) and 22 ft3 (6.7 m3) of oxygen, respectively. The reader is reminded that 1 ft3 (0.30 m3) equates to 28.3 L. Regardless of the size of the oxygen cylinder, it is filled to a tremendous pressure (2,200 psi). As a comparison, most automobile tires contain less than 50 psi.
A means of controlling the release of oxygen from the cylinder is necessary to permit safe and adjustable delivery to the patient. Typically, this includes three components:
Cylinder valve
Pressure-reducing valve
Flowmeter
In most applications, the pressure-reducing valve and flowmeter are combined into a single assembly called a regulator.
Cylinder Valve
Cylinders come with an onboard needle valve, which allows the tank to be opened and closed but does not allow pressure reduction or flow measurement. This valve opens when turned counterclockwise and closes when turned clockwise, much like household water taps. The outlet of the cylinder valve is safety indexed for each type of gas; only a specific oxygen regulator will attach to an oxygen cylinder’s valve. H cylinders use a threaded outlet to which a regulator is attached using a hex nut. E cylinders utilize a flat surface that receives a nipple from the oxygen regulator. This special type of regulator is not threaded but is pressed into position using a yoke-styled connection. Remember, only oxygen regulators will fit oxygen tanks. Generally, an appropriate hand tool (wrench) is required to connect and disconnect regulators from the cylinder valve. A special wrench is required to operate E cylinder valves.
Pressure-Reducing Valve
The cylinder valve is only a gross controller of flow, basically on or off. A pressure-reducing valve is used to reduce the extreme pressure within the cylinder to a working pressure, usually 50 psi. Fifty psi is still dangerously high for direct patient use but is a traditional working pressure for most ancillary oxygen therapy equipment (flowmeters, ventilators, nebulizers, etc.).
There are differing types of pressure regulators (preset, adjustable, and multistage), and most incorporate a round (Bourdon) gauge that measures the gas pressure within the tank. A full tank registers 2,200 psi but gradually drops as the cylinder empties. The registered pressure is accurate only when the cylinder valve is open.
It is possible to calculate how long the contents of an oxygen cylinder will last using a simple formula with the specific conversion factor (CF) for each cylinder size. The CF for E- and H-sized tanks is 0.28 and 3.14 L per psi, respectively.

Flowmeter
Flowmeters control and indicate the flow rate of oxygen. Given a fixed working pressure of 50 psi, the outlet flow increases in proportion to the opening of a needle valve control. The most common flowmeter uses a Thorpe tube. A small indicator (often a black ball) floats on the flow of gas as it passes through the tapered Thorpe tube. The tube is graduated in increments of liters per minute (lpm). The greater the flow, the higher the float rises within the tube.
Flowmeters are specific to a particular gas. It is wise to ensure oxygen flowmeters are used to measure oxygen flow. Pediatric oxygen flowmeters are available for finer control of gas flow. These flowmeters display flow in increments of 0.5 or even 0.25 lpm. The flow rate is read with the flowmeter in an upright position with the graduation that bisects the ball float indicating the liter flow.
Flowmeters can be used to turn off gas flow; however, if the cylinder is not being used for a prolonged time, it should be turned off using the cylinder valve and the flowmeter left open.
Having two controls (cylinder valve and flowmeter) can cause confusion. For example, the regulator may register near full pressure but then quickly drops to zero when the flowmeter is turned on. This occurs when the regulator is pressurized yet the cylinder valve is turned off. The pressure drops quickly as the relatively small volume of oxygen contained within the regulator bleeds down. This happens when the flowmeter is turned off before the cylinder valve is turned off. For this reason, it is important to watch the flowmeter for a few moments after the flow of oxygen begins to ensure that cylinder pressure is maintained.
Liquid Oxygen
Oxygen can be stored as a liquid. This requires very low temperatures (-183° C) maintained within a thermoslike cryogenic vessel. These systems operate under a
pressure of approximately 20 psi. As pressure is reduced, the liquid oxygen is converted into a gas. It is not possible to estimate the contents of a vessel containing liquid oxygen, because the vapor pressure remains constant until the tank is empty. This is analogous to estimating the amount of propane within a tank used with a domestic barbeque. The quantity of liquid gas contained within the vessel is measured in terms of weight, rather than pressure. The storage advantage is obvious when considering that a single cubic foot of liquid oxygen is equivalent to about 860 ft3 (262.1 m3) of oxygen gas. Liquid oxygen vessels can be transfilled, that is, one liquid system can be used to fill another. Patients requiring long-term oxygen treatment often use smaller, portable liquid oxygen vessels. Patients may arrive at the sleep center with portable liquid oxygen systems. Although the patient can easily be switched to the sleep center’s oxygen source, the technologist should be aware that the liquid oxygen will slowly vaporize and the vessel will empty even while not being used. This is similar to having a thermos of hot coffee that will slowly cool despite never being opened, the difference being that as liquid oxygen warms, it vaporizes to gas and escapes from the storage vessel.
pressure of approximately 20 psi. As pressure is reduced, the liquid oxygen is converted into a gas. It is not possible to estimate the contents of a vessel containing liquid oxygen, because the vapor pressure remains constant until the tank is empty. This is analogous to estimating the amount of propane within a tank used with a domestic barbeque. The quantity of liquid gas contained within the vessel is measured in terms of weight, rather than pressure. The storage advantage is obvious when considering that a single cubic foot of liquid oxygen is equivalent to about 860 ft3 (262.1 m3) of oxygen gas. Liquid oxygen vessels can be transfilled, that is, one liquid system can be used to fill another. Patients requiring long-term oxygen treatment often use smaller, portable liquid oxygen vessels. Patients may arrive at the sleep center with portable liquid oxygen systems. Although the patient can easily be switched to the sleep center’s oxygen source, the technologist should be aware that the liquid oxygen will slowly vaporize and the vessel will empty even while not being used. This is similar to having a thermos of hot coffee that will slowly cool despite never being opened, the difference being that as liquid oxygen warms, it vaporizes to gas and escapes from the storage vessel.
Liquid oxygen is more expensive than compressed oxygen, and its primary advantage is storage. Most hospitals and other high-volume users store oxygen on-site in its liquid form.
Oxygen Concentrators
These self-contained, somewhat portable devices use electricity to power a compressor and filtration system. Filtering of room air through a molecular sieve bed or permeable plastic membrane produces concentrated oxygen. Oxygen concentrators are not capable of delivering high flows or high concentrations of oxygen and are therefore not suited to emergency situations.
Modern oxygen concentrators are convenient, quiet, and safe and provide a reliable source of relatively inexpensive low-flow oxygen. Because of these benefits, concentrators are a good oxygen source for sleep centers located outside of hospitals. The reader is encouraged to become familiar with their respective oxygen concentrator in terms of flow rate capability and oxygen concentration specifications. Oxygen concentration should be checked as a part of the laboratory equipment quality assurance process.
OXYGEN DELIVERY IN THE SLEEP CENTER
Precautions
Pure oxygen itself presents potential physical dangers. First, oxygen cylinders contain very high pressures. It must be ensured that tanks are secured from falling or tipping to avoid tank or regulator breakage. Oxygen cylinders must be stored away from heat sources. Liquid oxygen is of extremely low temperature, and the vaporizing liquid can cause low-temperature burns to the skin. Oxygen itself is not explosive but vigorously supports combustion. Oxygen and materials that are flammable make for a very dangerous mix. For example, collodion, which is both volatile and flammable, should not be used in the vicinity of oxygen.
Oxygen also poses physiologic consequences. Prolonged high alveolar oxygen levels (>60%) are associated with the production of free radicals and lung damage (oxygen toxicity). Alveolar oxygen concentrations of more than 80% (with special circumstances) can be associated with regional microlung collapse (absorption atelectasis).
Hypoxic Drive
The predominate stimulus to breathe is carbon dioxide level. There is also a secondary drive to breathe motivated by hypoxemia, and it is termed “hypoxic drive,” which is a normal mechanism and can be accentuated at high altitude.
Some chronic lung diseases cause chronic elevation of PaCO2 (hypercarbia). Chronic hypercarbia, it is argued, causes normal sensitivity to carbon dioxide to become blunted, and hypoxia becomes a predominant stimulus to breathe. These patients are said to be dependent on their hypoxic drive; their drive to breathe is diminished when oxygen is administered, and they may become hypopneic or apneic.
Although patients with chronic carbon dioxide retention are predisposed to being more reliant on their hypoxic drive, the dependence is inconsistent. Patients should be closely monitored, and the technologist should be mindful of potential ventilatory drive changes.
Hypoxic drive is a term that is overused, poorly understood, and often misdiagnosed. Hypoxic drive is certainly not a valid argument for withholding oxygen administration when treating hypoxemia.
Aims and Rationale of Intervention
The objective of treatment is to use the least amount of oxygen to bring about the desired therapeutic effect, which is often defined as a predetermined level of oxygen saturation. Various devices are available to deliver oxygen to patients, each with inherent limitations. Choosing the optimum delivery method is dependent on therapeutic goals, clinical status, and delivery device limitations.
It is advisable to establish both criteria and method for oxygen usage before performing polysomnography. Such guidelines are set by the physician and are usually based on patient clinical circumstance. The sleeping patient is particularly challenging, because changes in blood
oxygen saturation are normal (during rapid eye movement), often transient, and perhaps expected with patients with preexisting respiratory pathology (2). Setting limits of desaturation during polysomnography is a medical determination and must be clear to the technologist.
oxygen saturation are normal (during rapid eye movement), often transient, and perhaps expected with patients with preexisting respiratory pathology (2). Setting limits of desaturation during polysomnography is a medical determination and must be clear to the technologist.
Patients with sleep-disordered breathing (SDB) who require oxygen therapy when awake will often require oxygen when sleeping despite optimal maintenance of airway patency. In these instances, oxygen may be a useful adjunct to CPAP/BPAP treatment (3).
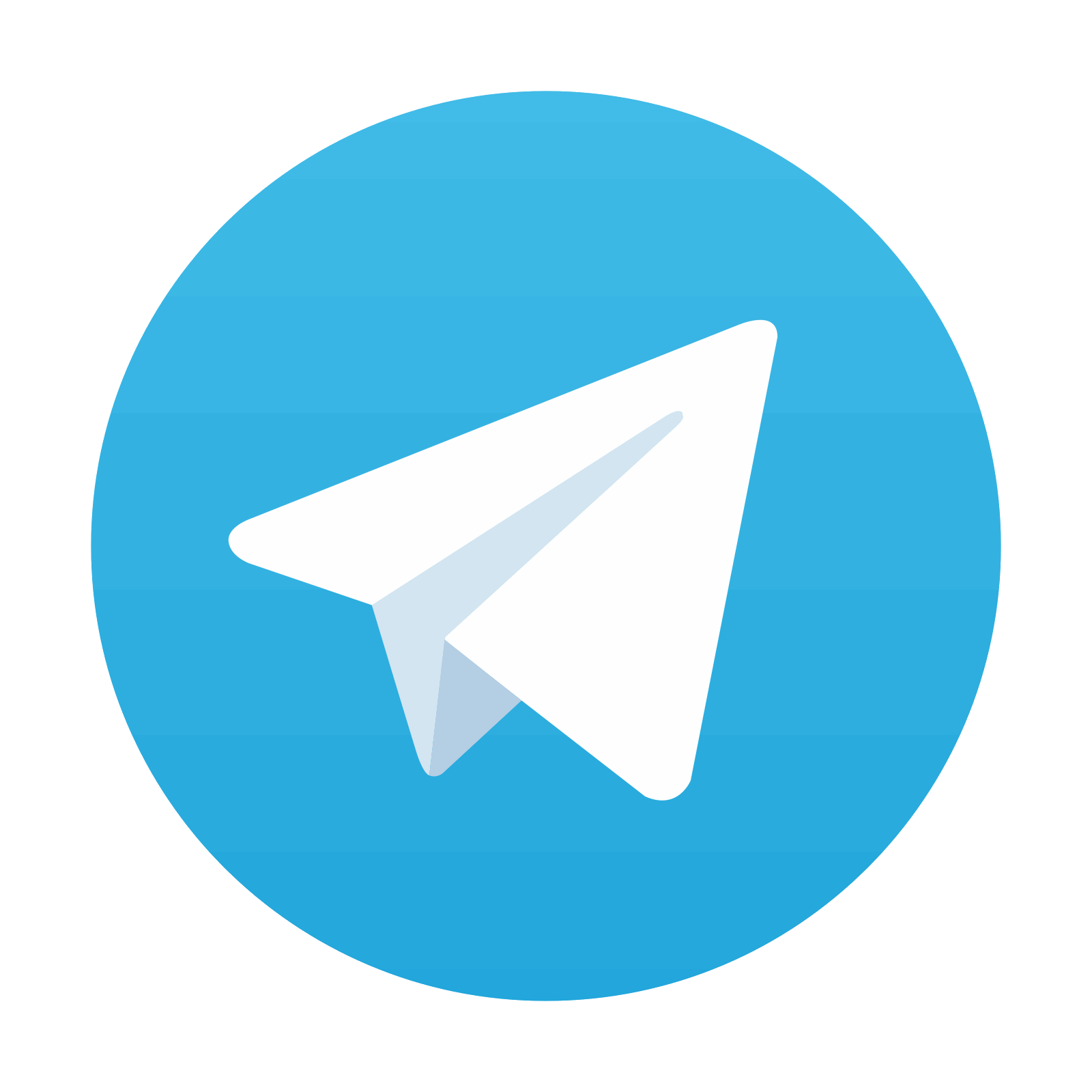
Stay updated, free articles. Join our Telegram channel

Full access? Get Clinical Tree
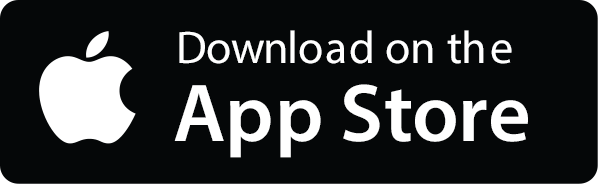
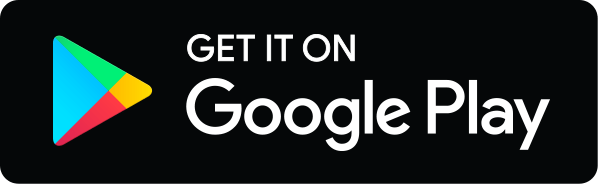
