Oxygen and Gas Exchange in the Body
Stephen Tarnoczy
LEARNING OBJECTIVES
On completion of this chapter, the reader should be able to:
1. Describe the basic exchange of gases within the body as well as the outside environment.
2. Discuss the methods for oxygen delivery to the tissues throughout the body.
3. Define the relationship between oxygen and hemoglobin and factors that alter their relationship.
4. Describe how disease may impact oxygen diffusion, transport, and/or delivery.
5. Define the different types of hypoxia, hypoxemia, and ischemia.
6. Describe how supplemental oxygen affects the healthy and compromised patient.
KEY TERMS
Ventilation
External respiration
Internal respiration
Room air
Alveoli
Hypoxia
Anemic hypoxia
Circulatory hypoxia
Hypoxemia
Ischemia
Anemia
Hemoglobin
The human organism is a magnificent yet complex machine that operates with the precision of a fine Swiss watch. Like any mechanical device, the human organism is the sum of all its parts. Rather than analyzing gears, springs, and pendulums as the primary makeup of our machine, we will be referring to organs, tissues, and cells to describe the processes of the body and the wonderment of respiration.
RESPIRATION VERSUS VENTILATION
A very important concept surrounding this topic of discussion is the distinction between respiration and ventilation, which are two very different processes. Ventilation is defined as “the process of moving gas (usually air) in and out of the lungs” (1). This is completely independent of respiration, where the exchange of gas actually occurs either in the lungs (external respiration) or at the level of the tissues inside the body (internal respiration). These two very important areas will be addressed, but only respiration will be covered in this chapter. The mechanism of ventilation (moving air in and out of the lungs) will be covered in another forum. It is important to realize that ventilating a patient does not ensure that respiration is actually taking place and vice versa. Rather than dwelling on these two distinctions, let us examine the forces and laws that govern the miracle of respiration.
External Respiration
The exchange of gas between the body and the outside environment is referred to as “external respiration.” The process of external respiration takes place between the lungs and the atmospheric air we breathe. This takes place in the tiny spherical sacs of the lungs called “alveoli” and the blood supply carried in by very tiny blood vessels in the lungs called the “pulmonary capillaries.” Collectively, we refer to the conjoining of these two systems as the “alveolar-capillary membrane” (ACM). The process of gas exchange at this level is one of physics and pure diffusion, the movement of gas from an area of high concentration to an area of low concentration, until the two areas are equilibrated or equal. As we take air in or inhale air (inhalation), we are bringing in a gas (air) that comprises primarily nitrogen (N2) and 20.9% oxygen (O2). The amount of oxygen in the inspired air, or the fraction of inspired oxygen (FiO2), is greater inside the alveoli than the amount of oxygen contained in the pulmonary capillaries. Utilizing the gradient of diffusion and movement of gas from a high to a low concentration, oxygen leaves the alveoli, crosses the ACM, and enters the red blood cell (RBC). As oxygen moves in, carbon dioxide (CO2) is simultaneously moving out. Again, under the laws of diffusion, the amount of CO2
inside the RBC is significantly higher than the amount of CO2 inside the alveoli, and CO2 diffuses from the RBC into the alveoli to be excreted or expired during exhalation. Under normal conditions, this takes place very quickly at the level of the ACM, usually in about 0.25 seconds. The amount of contact time that the average RBC has with the ACM is about 0.75 seconds.
inside the RBC is significantly higher than the amount of CO2 inside the alveoli, and CO2 diffuses from the RBC into the alveoli to be excreted or expired during exhalation. Under normal conditions, this takes place very quickly at the level of the ACM, usually in about 0.25 seconds. The amount of contact time that the average RBC has with the ACM is about 0.75 seconds.
Internal Respiration
After the exchange of gas in the lungs, oxygenated blood returns to the heart to be distributed throughout the body through the left ventricle, arteries, and the body’s systemic circulation. The blood travels the arterial circulation to be distributed throughout the body and to its many organ systems. As the RBCs make their way through the smaller blood vessels, they eventually reach the arterial capillaries of the body. These capillaries are the end point of a network of blood vessels that feed the entire body with nutrients, electrolytes, and oxygen. At the capillary level, much as in the capillaries of the lungs, the RBC comes into contact with the capillary wall and the adjoining tissues.
Under normal circumstances, internal or cellular respiration utilizes oxygen to create energy, with the waste product primarily being CO2 through the aerobic pathway of the Krebs cycle. At the tissue level, the amount of oxygen inside the RBC is greater than the amount outside the RBC in the tissues, and O2 moves out of the RBC and into the tissues. Again, simultaneous exchange of CO2 is taking place as CO2 leaves the tissues and moves into the plasma and RBC for return to the lungs and elimination; then the process starts all over again. The concentration of CO2 is greater in the tissues compared with the RBC, so CO2 leaves the tissues and diffuses out into the plasma and RBC. The transport of both CO2 and O2 within the body is a bit more complex than described here, but it sets the foundation for further discussion.
ATMOSPHERIC GAS AND PARTIAL PRESSURES OF OXYGEN
Depending on where you live, the atmosphere of the air you breathe exerts a pressure that is equal to about 760 mm Hg, 1 atm, or 14.7 pounds per square inch (psi). At higher altitudes above sea level, that pressure gradually begins to decrease. As you go below the sea level, the opposite occurs and the pressure actually increases by 760 mm Hg, 1 atm, or 14.7 psi for every 33 ft you are below the water’s surface! The concentration of gas or the amount of oxygen in room air of 21% never changes, but rather the pressure that it is under in the atmosphere changes, causing the gas to behave differently. Take a balloon and blow it up. You have a balance of air pressures that keep the balloon inflated. You have air inside the balloon exerting a force outward in all directions against the inside wall. You have air in the atmosphere exerting pressure on the balloon at 760 mm Hg. Keeping all other variables constant, if you were to take the balloon and submerge it under a pool of water, the balloon would shrink in size. Elevating the same balloon several thousand feet will cause it to expand. The gas in the balloon never went anywhere, but the environmental pressure on the outside of the balloon changed! Now notice the phrase keeping all other variables constant because that is very important when you are dealing with any gas and demonstrating its physical properties.
Regardless of the units of measure used, the atmospheric pressure (760 mm Hg) is a combination of all the gases in room air. Because room air is made up primarily of nitrogen and oxygen, these two gases make up the greatest percentage of the atmospheric pressure of 760 mm Hg. For example, room air is 21% oxygen and 79% nitrogen. There are additional “trace” gases or elements also present in extremely small amounts, but the air we breathe is primarily nitrogen and oxygen. Of the 760 mm Hg pressure exerted by the atmosphere, 160 mm Hg pressure is exerted by oxygen and 600 mm Hg pressure is exerted by nitrogen. Because air does not comprise 100% pure gas but rather is a mixture of these two elements, we refer to the pressures exerted by nitrogen and oxygen as partial pressures. Adding these two pressures together, we get the 760 mm Hg of total pressure exerted by our atmosphere on an average day. This calculation is known as Dalton’s law of partial pressure: The partial pressure of a gas is equal to the concentration of that gas (expressed as a decimal) × the atmospheric pressure (760 mm Hg in our example).
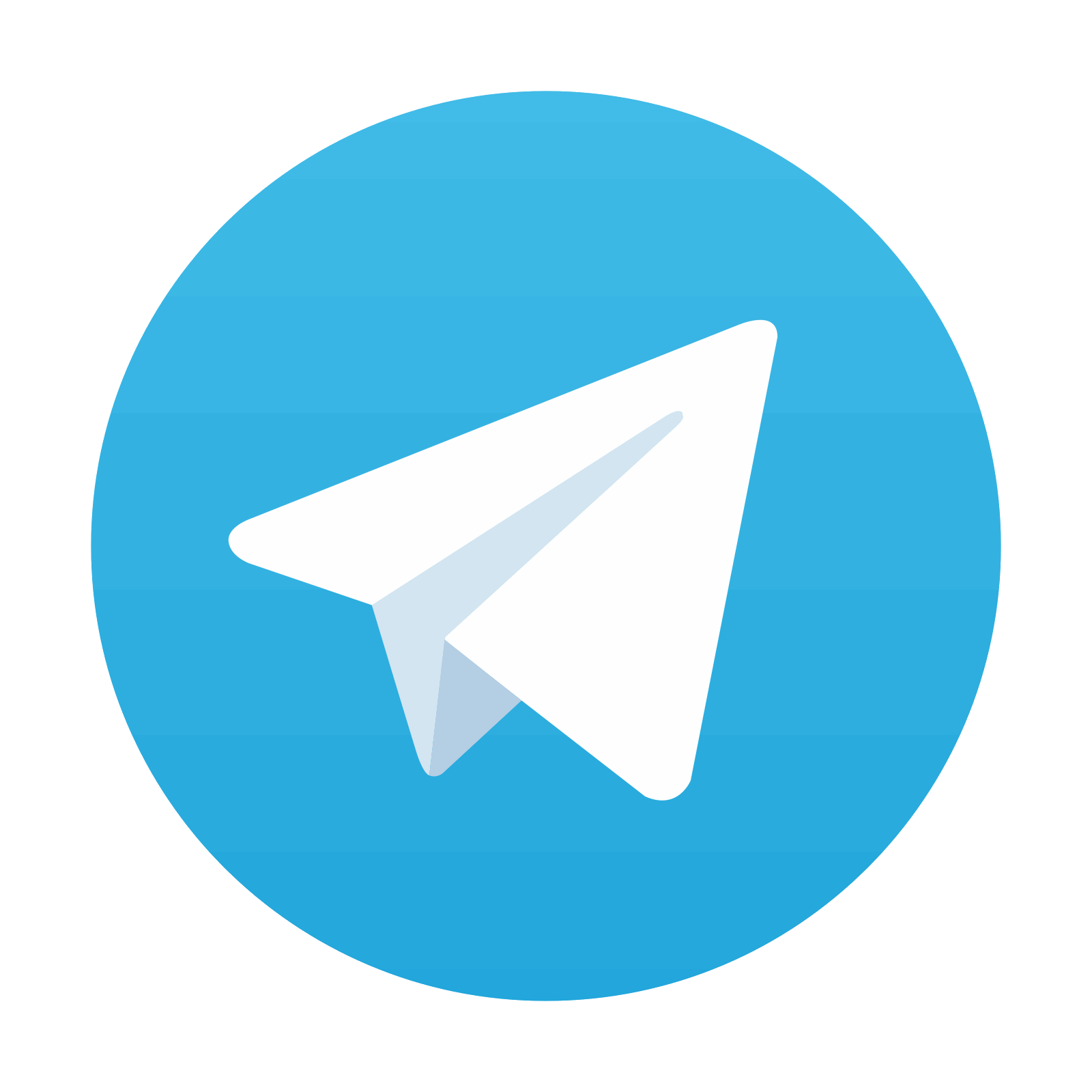
Stay updated, free articles. Join our Telegram channel

Full access? Get Clinical Tree
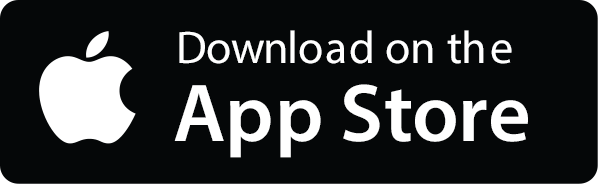
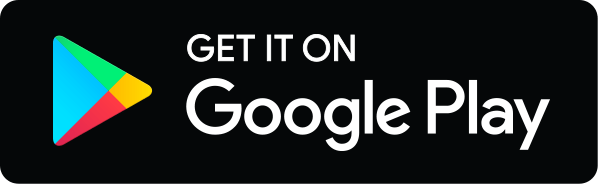