Fig. 14.1
Expression in the paraventricular nucleus (PVN) of OT, as measured by ICC, is reduced in Fmr1 KO mice, compared to the wild-type (WT). (Reprinted from Brain Research, Francis et al. 2014, Copyright (2014) with permission from Elsevier)

Fig. 14.2
Expression in the paraventricular nucleus (PVN) of AVP. In Fmr1 KO mice, as compared to the wild-type (WT) AVP expression is reduced as measured by ICC. (Reprinted from Brain Research, Francis et al. 2014, Copyright (2014) with permission from Elsevier)
Table 14.1
Number of OT and AVP-positive cells in PVN of Fmr1 KO versus WT mice. (Reprinted from Brain Research, Francis et al. 2014, Copyright (2014) with permission from Elsevier)
OT | AVP | |||||
---|---|---|---|---|---|---|
WT | Knockout | WT | Knockout | |||
PVN | 17a ± 2b | 9 ± 3 | p= 0.047 | 10 ± 2 | 4 ± 2 | p= 0.05 |
Table 14.2
Number of OT and AVP-positive cells in SON of Fmr1 KO versus WT mice. (Reprinted from Brain Research, Francis et al. 2014, Copyright (2014) with permission from Elsevier)
OT | AVP | |||||
---|---|---|---|---|---|---|
WT | Knockout | WT | Knockout | |||
SON | 13a ± 2b | 8 ± 3 | p= 0.254 | 11 ± 2 | 6 ± 2 | p= 0.107 |
Although the preliminary data shown here for Fmr1 KO mice needs to be replicated in a larger sample size and in other animal models , we include these findings as an example of possible approaches to examining the role of peptides, including OT and AVP, in molecularly characterized genetic syndromes. Work across these models also could provide additional insight regarding the role of OT and AVP in early development, especially in syndromes in which atypical trajectories occur.
14.6 Conclusion and Next Steps
While each of the disorders described here (ASD, PWS, WS and FXS) is unique, each one is characterized by atypical social behaviors and in many cases a tendency toward high levels of anxiety. Given the importance of OT and AVP to mammalian social behaviors and anxiety, the neuropeptides’ investigative value in these syndromes is not unexpected. This review summarized the possible role of OT in these NDD through experiments conducted by others and ourselves (Table 14.3). Each of these early developmental disorders displayed alterations in the OT system and may represent many molecular pathways that lead to a commonly disrupted neuropeptide hormone system. Our preliminary data suggests decreased numbers of OT-positive and AVP-positive cells in the PVN of Fmr1 KO mice, a mouse model for FXS. Individuals with PWS have shown lower levels of OT in CSF and fewer OT producing cells in the PVN. Lower plasma OT levels have also been detected in some children or a subgroup of ASD affected children. In contrast with WS, which is characterized by hypersociability, a positive correlation was found between OT levels and increased stranger approach and decreased adaptive social behavior. Knowledge of the functionality of the OXTR in WS remains to be studied. Given the rarity of these disorders and the complex animal models needed to research these disorders, many of these studies have small sample sizes. These significant studies, however, can motivate future research on these disorders and other NDD, especially those disorders with dysfunctional social behaviors as a symptom.
Table 14.3
A Summary of OT Affects on NDD (Modified from Brain Research, Francis et al., 2014, Copyright (2014) with permission from Elsevier)
Disorder | Neuropeptide system affected |
---|---|
Autism spectrum disorders | ↓↑ or atypical levels of OT in blood (human) |
IN-OT ↑ social task performance and ↓ repetitive behaviors (human) | |
To be studied:human neuropathology and animal models | |
Prader-willi syndrome | ↓ OT producing cells in the PVN (human) |
↓ level of OT in CSF (human) | |
IN-OT ↑ trust and ↓ disruptive behaviors (human) | |
Williams syndrome | ↑ OT levels (human) |
To be studied:human neuropathology and animal models | |
Fragile X Syndrome | ↓ OT + and AVP + cells in the PVN (Fmr1 KO mice) |
↓ OXTR + cells in several areas of the brain related to learning, memory and emotion (Fmr1 KO mice) | |
IN-OT ↑ eye gaze frequency (human) | |
IN-OT ↓ salivary cortisol (human) |
ASD, as described above, is the primary NDD presumed to be associated with dysregulation of the OT system. It is striking, however, that other disorders with phenotypes marked by abnormal social behavior as well as anxiety (manifested in RRB) have abnormalities in OT production as measured in blood and CSF (Table 14.3). For example, individuals with PWS, like those in ASD, have difficulty with social competence (Dimitropoulos et al. 2013), are aloof and avoid eye contact (Dimitropoulos et al. 2009). Furthermore, RRB is also evidenced in PWS (Greaves et al. 2006), although to a lesser degree than in ASD as measured using the Repetitive Behavior Scale-Revised (RBS-R; Flores et al. 2011). A subset of the chromosomal region associated with PWS is also associated with an increased risk for ASD, as maternally inherited duplications of the 15q11-13 region are associated with 1–3 % of ASD cases (Bolton et al. 2001; Cook et al. 1997; Vorstman et al. 2006).
Williams syndrome and ASD also share commonalities, namely abnormal social phenotypes and anxiety. Individuals with WS, unlike those with PWS, show a phenotype that is markedly different from ASD. Although both groups are at risk for anxiety, individuals with ASD show higher levels of social phobia and separation anxiety, as well as higher rates of RRB. However, children with WS have higher scores on measures of generalized anxiety (Rodgers et al. 2012). WS is characterized by an increase in OT levels (Dai et al., 2012), as well as a deletion of the 7q11.23 region as opposed to a de novo duplication which leads to ASD (Sanders et al., 2011). Thus, it is likely that some ASD and WS symptoms are related to genetic dosage effects. Studies of FX and ASD mechanism may also inform each other, as mutations in mGluR5 can contribute to the diagnosis of FXS or ASD, and mGluR5 antagonists have shown promise in alleviating ASD symptoms in mouse models (Silverman et al. 2012) as well as Fragile X pathology.
As summarized in this review, animal and human research to date has shown that dysregulation of the OT system is associated with marked deficits in social behavior as well as anxiety. This commonality across multiple NDD may indicate a shared OT pathway that is affected during development. The use of animal models , particularly those developed for FXS, WS and PWS, will provide insight into such a pathway, as these disorders have well characterized genetics, whereas there are over 103 disease genes and 44 genomic loci reported to be involved in ASD (Betancur 2011). However, unlike in ASD, there is a lack of human data on the pathophysiology of FXS, WS and PWS, as well as pharmacological interventions. Ideally, scientists want to identify specific molecular pathways to target distinct syndromes and disorders for treatment. However, many effective treatments modulate common neurochemical or hormone pathways that are downstream from etiologically contributing factors (e.g. drugs for hypertension). Combining the strengths of human and animal model studies across these NDD may provide important clues into the role of OT in development, in addition to elucidating the complex neurophysiology and treatment targets for FXS, PWS, WS and ASD.
Acknowledgements
This work was supported in part by NIH K23MH082121 (SJ). The authors would also like to thank Jeanine Leary and Jennifer Speak for their assistance in preparing the text.
References
Abramson RK, Wright HH, Carpenter R, Brennan W, Lumpuy O, Cole E, Young SR (1989) Elevated blood serotonin in autistic probands and their first-degree relatives. J Autism Dev Disord 19:397–407PubMed
American Psychiatric Association (2013) Diagnostic and statistical manual of mental disorders, 5th edn. American Psychiatric Publishing, Arlington
Anagnostou E, Soorya L, Chaplin W, Bartz J, Halpern D, Wasserman S, Wang AT, Pepa L, Tanel N, Kushki A, Hollander E (2012) Intranasal oxytocin versus placebo in the treatment of adults with autism spectrum disorders: a randomized controlled trial. Mol Autism 3:16PubMedCentralPubMed
Andari E, Duhamel JR, Zalla T, Herbrecht E, Leboyer M, Sirigu A (2010) Promoting social behavior with oxytocin in high-functioning autism spectrum disorders. Proc Natl Acad Sci U S A 107:4389–4394PubMedCentralPubMed
Appenrodt E, Schnabel R, Schwarzberg H (1998) Vasopressin administration modulates anxiety-related behavior in rats. Physiol Behav 64:543–547PubMed
Ashley CT Jr, Wilkinson KD, Reines D, Warren ST (1993) FMR1 protein: conserved RNP family domains and selective RNA binding. Science 262:563–566PubMed
Bailey A, Le Couteur A, Gottesman I, Bolton P, Simonoff E, Yuzda E, Rutter M (1995) Autism as a strongly genetic disorder: evidence from a British twin study. Psychol Med 25:63–77PubMed
Bakker CE, Verheij C, Willemsen R, Vanderhelm R, Oerlemans F, Vermey M, Bygrave A, Hoogeveen AT, Oostra BA, Reyniers E, Deboulle K, Dhooge R, Cras P, Vanvelzen D, Nagels G, Martin JJ, Dedeyn PP, Darby JK, Willems PJ (1994) Fmr1 knockout mice—a model to study fragile-X mental-retardation. Cell 78:23–33
Bartz JA, Hollander E (2006) The neuroscience of affiliation: forging links between basic and clinical research on neuropeptides and social behavior. Horm Behav 50:518–528PubMed
Baumgartner T, Heinrichs M, Vonlanthen A, Fischbacher U, Fehr E (2008) Oxytocin shapes the neural circuitry of trust and trust adaptation in humans. Neuron 58:639–650PubMed
Bernardet M, Crusio WE (2006) Fmr1 KO mice as a possible model of autistic features. Scientific World Journal 6:1164–1176PubMed
Berry-Kravis E, Grossman AW, Crnicz LS, Greenough WT (2002) Understanding fragile X syndrome. Curr Paediatr 12:316–324
Berry-Kravis E, Knox A, Hervey C (2011) Targeted treatments for fragile X syndrome. J Neurodev Disord 3:193–210PubMedCentralPubMed
Betancur C (2011) Etiological heterogeneity in autism spectrum disorders: more than 100 genetic and genomic disorders and still counting. Brain Res 1380:42–77PubMed
Bittel DC, Kibiryeva N, McNulty SG, Driscoll DJ, Butler MG, White RA (2007a) Whole genome microarray analysis of gene expression in an imprinting center deletion mouse model of Prader-Willi syndrome. Am J Med Genet A 143:422–429
Bittel DC, Kibiryeva N, Sell SM, Strong TV, Butler MG (2007b) Whole genome microarray analysis of gene expression in Prader-Willi syndrome. Am J Med Genet A 143:430–442
Boccaccio I, Glatt-Deeley H, Watrin F, Roeckel N, Lalande M, Muscatelli F (1999) The human MAGEL2 gene and its mouse homologue are paternally expressed and mapped to the Prader-Willi region. Hum Mol Genet 8:2497–2505PubMed
Bolton PF, Dennis NR, Browne CE, Thomas NS, Veltman MW, Thompson RJ, Jacobs P (2001) The phenotypic manifestations of interstitial duplications of proximal 15q with special reference to the autistic spectrum disorders. Am J Med Genet 105:675–685PubMed
Borghgraef M, Fryns JP, Van Den Berghe H (1990) Psychological profile and behavioural characteristics in 12 patients with Prader-Willi syndrome. Genet Counsel 1:141–150PubMed
Born J, Lange T, Kern W, McGregor GP, Bickel U, Fehm HL (2002) Sniffing neuropeptides: a transnasal approach to the human brain. Nat Neurosci 5:514–516PubMed
Brown WT (1990) The fragile X: progress toward solving the puzzle. Am J Hum Genet 47:175–180PubMedCentralPubMed
Campbell DB, Datta D, Jones ST, Batey Lee E, Sutcliffe JS, Hammock EA, Levitt P (2011) Association of oxytocin receptor (OXTR) gene variants with multiple phenotype domains of autism spectrum disorder. J Neurodev Disord 3:101–112PubMedCentralPubMed
Carter CS (1998) Neuroendocrine perspectives on social attachment and love. Psychoneuroendocrinology 23:779–818PubMed
Carter CS (2007) Sex differences in oxytocin and vasopressin: implications for autism spectrum disorders? Behav Brain Res 176:170–186PubMed
Cascio CJ, Foss-Feig JH, Heacock JL, Newsom CR, Cowan RL, Benningfield MM, Rogers BP, Cao A (2012) Response of neural reward regions to food cues in autism spectrum disorders. J Neurodev Disord 4:9PubMedCentralPubMed
Cassidy SB, Schwartz S, Miller JL, Driscoll DJ (2012) Prader-Willi syndrome. Genet Med 14:10–26PubMed
Ceman S, Nelson R, Warren ST (2000) Identification of mouse YB1/p50 as a component of the FMRP-associated mRNP particle. Biochem Biophys Res Comm 279:904–908PubMed
Chaste P, Leboyer M (2012) Autism risk factors: genes, environment, and gene-environment interactions. Dialogue Clin Neurosci 14:281–292
Chen L, Toth M (2001) Fragile X mice develop sensory hyperreactivity to auditory stimuli. Neurosci 103:1043–1050
Chini B, Mouillac B, Balestre MN, Trumpp-Kallmeyer S, Hoflack J, Hibert M, Andriolo M, Pupier S, Jard S, Barberis C (1996) Two aromatic residues regulate the response of the human oxytocin receptor to the partial agonist arginine vasopressin. FEBS Lett 397:201–206PubMed
Cho MM, DeVries AC, Williams JR, Carter CS (1999) The effects of oxytocin and vasopressin on partner preferences in male and female prairie voles (Microtus ochrogaster). Behav Neurosci 113:1071–1079PubMed
Chugani DC, Muzik O, Behen M, Rothermel R, Janisse JJ, Lee J, Chugani HT (1999) Developmental changes in brain serotonin synthesis capacity in autistic and nonautistic children. Ann Neurol 45:287–295PubMed
Comery TA, Harris JB, Willems PJ, Oostra BA, Irwin SA, Weiler IJ, Greenough WT (1997) Abnormal dendritic spines in fragile X knockout mice: maturation and pruning deficits. Proc Natl Acad Sci U S A 94:5401–5404PubMedCentralPubMed
Cook EH Jr, Lindgren V, Leventhal BL, Courchesne R, Lincoln A, Shulman C, Lord C, Courchesne E (1997) Autism or atypical autism in maternally but not paternally derived proximal 15q duplication. Am J Hum Genet 60:928–934PubMedCentralPubMed
Corbin F, Bouillon M, Fortin A, Morin S, Rousseau F, Khandjian EW (1997) The fragile X mental retardation protein is associated with poly(A)+mRNA in actively translating polyribosomes. Hum Mol Genet 6:1465–1472PubMed
Cornish KM, Munir F, Cross G (1999) Spatial cognition in males with Fragile-X syndrome: evidence for a neuropsychological phenotype. Cortex 35:263–271PubMed
Crawley JN, Chen T, Puri A, Washburn R, Sullivan TL, Hill JM, Young NB, Nadler JJ, Moy SS, Young LJ, Caldwell HK, Young WS (2007) Social approach behaviors in oxytocin knockout mice: comparison of two independent lines tested in different laboratory environments. Neuropeptides 41:145–163PubMed
Cronbach LJ, Meehl PE (1955) Construct validity in psychological tests. Psychol Bull 52:281–302PubMed
D’Hooge R, Nagels G, Franck F, Bakker CE, Reyniers E, Storm K, Kooy RF, Oostra BA, Willems PJ, De Deyn PP (1997) Mildly impaired water maze performance in male Fmr1 knockout mice. Neuroscience 76:367–376PubMed
Dadds MR, Macdonald E, Cauchi A, Williams K, Levy F, Brennan J (2014) Nasal oxytocin for social deficits in childhood autism: a randomized controlled trial. J Autism Dev Disord 44:521–531PubMed
Dai L, Carter CS, Ying J, Bellugi U, Pournajafi-Nazarloo H, Korenberg JR (2012) Oxytocin and vasopressin are dysregulated in Williams Syndrome, a genetic disorder affecting social behavior. PloS One 7:e38513PubMedCentralPubMed
Davidovic L, Navratil V, Bonaccorso CM, Catania MV, Bardoni B, Dumas ME (2011) A metabolomic and systems biology perspective on the brain of the fragile X syndrome mouse model. Genome Res 21:2190–2202PubMedCentralPubMed
De Boulle K, Verkerk AJ, Reyniers E, Vits L, Hendrickx J, Van Roy B, Van den Bos F, de Graaff E, Oostra BA, Willems PJ (1993) A point mutation in the FMR-1 gene associated with fragile X mental retardation. Nat Genet 3:31–35PubMed
Devys D, Lutz Y, Rouyer N, Bellocq JP, Mandel JL (1993) The FMR-1 protein is cytoplasmic, most abundant in neurons and appears normal in carriers of a fragile X premutation. Nat Genet 4:335–340PubMed
Dimitropoulos A, Feurer ID, Roof E, Stone W, Butler MG, Sutcliffe J, Thompson T (2000) Appetitive behavior, compulsivity, and neurochemistry in Prader-Willi syndrome. Ment Retard Dev Disabil Res Rev 6:125–130PubMed
Dimitropoulos A, Ho AY, Klaiman C, Koenig K, Schultz RT (2009) A comparison of behavioral and emotional characteristics in children with autism, Prader-Willi syndrome, and Williams syndrome. J Ment Health Res Intellect Disabil 2:220–243
Dimitropoulos A, Ho A, Feldman B (2013) Social responsiveness and competence in Prader-Willi syndrome: direct comparison to autism spectrum disorder. J Autism Dev Disord 43:103–113PubMed
Dombret C, Nguyen T, Schakman O, Michaud JL, Hardin-Pouzet H, Bertrand MJ, De Backer O (2012) Loss of Maged1 results in obesity, deficits of social interactions, impaired sexual behavior and severe alteration of mature oxytocin production in the hypothalamus. Hum Mol Genet 21:4703–4717PubMed
Domes G, Heinrichs M, Michel A, Berger C, Herpertz S (2007) Oxytocin improves “mind-reading” in humans. Biol Psychiatry 61:731–733PubMed
Domes G, Sibold M, Schulze L, Lischke A, Herpertz SC, Heinrichs M (2013) Intranasal oxytocin increases covert attention to positive social cues. Psychol Med 43:1747–1753PubMed
Eberhart DE, Malter HE, Feng Y, Warren ST (1996) The fragile X mental retardation protein is a ribonucleoprotein containing both nuclear localization and nuclear export signals. Hum Mol Genet 5:1083–1091PubMed
Ebstein RP, Israel S, Lerer E, Uzefovsky F, Shalev I, Gritsenko I, Riebold M, Salomon S, Yirmiya N (2009) Arginine vasopressin and oxytocin modulate human social behavior. Ann N Y Acad Sci 1167:87–102PubMed
Ebstein RP, Knafo A, Mankuta D, Chew SH, Lai PS (2012) The contributions of oxytocin and vasopressin pathway genes to human behavior. Horm Behav 61:359–379PubMed
Einfeld SL, Tonge BJ, Florio T (1997) Behavioral and emotional disturbance in individuals with Williams syndrome. Am J Ment Retard 102:45–53PubMed
Ewart AK, Morris CA, Atkinson D, Jin W, Sternes K, Spallone P, Stock AD, Leppert M, Keating MT (1993) Hemizygosity at the elastin locus in a developmental disorder, Williams syndrome. Nat Genet 5:11–16PubMed
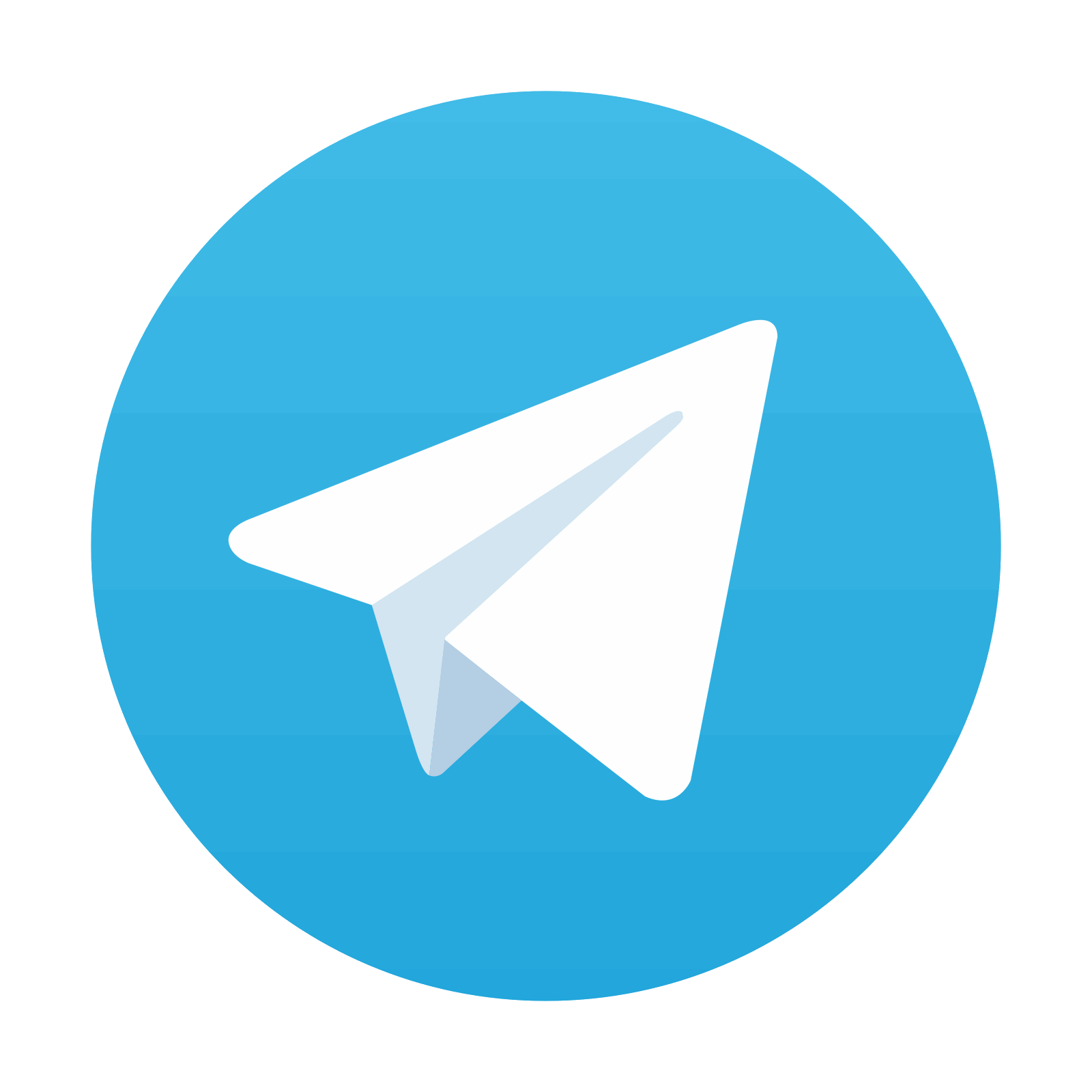
Stay updated, free articles. Join our Telegram channel

Full access? Get Clinical Tree
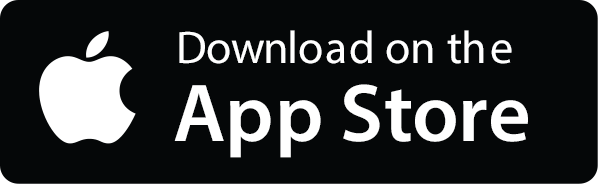
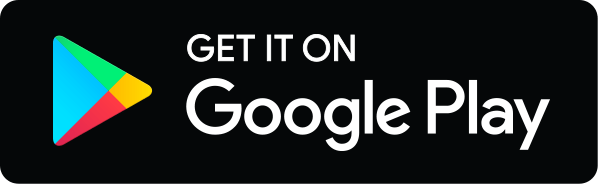