2 The Acquisition of Human Verticality
Abstract
The shift from facultative to permanent bipedalism was a pivotal step in human evolution. Skeletal adaptations to efficient sagittal balance of the trunk are therefore key to identify fossils as our ancestors, the hominids. Morphological modifications of the pelvis and spine had a major role in this process. Here, we review these evolutionary adaptations that resulted in the formation of the spinopelvic functional unit. We suggest that the double S-shape of the vertebral column evolved secondary to the functionally linked pelvic modifications. Together with the lumbar lordosis, the approximation of the sacroiliac and hip joints brought the center of body mass closer to the hip joints, thus minimizing muscular work to maintain equilibrium. A prerequisite for the adoption of lumbar lordosis in early hominids was a long and mobile lumbar spine. As great apes have a rigid spine with three to four lumbar vertebrae, different scenarios have been proposed for the evolution of the human spinal segmentation. We argue that the common ancestor of chimpanzees and humans already possessed five lumbar vertebrae, and a pelvic incidence of ~ 30° that increased during evolution as the sacro-acetabular distance decreased. The strong correlation in humans between pelvic incidence and lumbar lordosis points toward an elaborated functional link that was shaped by natural selection. A review of the hominid fossil record, including Sahelanthropus, Orrorin, Ardipithecus ramidus, Australopithecus afarensis, Australopithecus africanus, Australopithecus sediba, Homo erectus, and Neanderthals, suggests that this link between pelvis and spine was probably only established with H. erectus 1.5 million years ago.
2.1 Introduction
The adaptation to bipedalism represents the primary change during human evolution that permits to diagnose fossils as our ancestors, the hominids. This group includes all forms in the lineage leading to modern humans after the split from the chimpanzee lineage some 5 to 8 million years ago. Adaptation to bipedalism therefore occurred long before the production of stone tools, the loss of dense body hair, and the increase in brain size.
Nonhuman primates live mostly in trees and show a diverse locomotor repertoire. This polyvalence with versatile locomotor abilities is therefore also expected in our earliest hominid ancestors. Their varied arboreal locomotor repertoire included quadrupedalism, vertical climbing, suspension, and occasional bipedalism. It was the percentage of terrestrial bipedalism that increased in our first ancestors in relation to the environment, together with its advantages and its constraints. Only later during human evolution, our ancestors became permanent bipeds, which represents a strong specialization.
Sagittal balance of the trunk over the hip joints is essential for efficient bipedal locomotion. Consequently, its acquisition during the evolution of hominids was crucial in the shift from a facultative to a permanent form of bipedalism. The modification of the sagittal morphology of the pelvis and spine played a major role in the process of this evolutionary adaptation, which resulted in the formation of a spinopelvic functional unit. 1 We suggest that the double S-shape of the vertebral column, often considered as one of the most important evolutionary adaptations to bipedal locomotion, evolved secondary to the functionally linked modifications of the pelvis.
2.2 Compared Anatomy: The Axial Skeleton in Quadrupeds, Apes, and Hominids
To understand and interpret the evolution of the human morphology, we must use the tool of comparative anatomy. Here, we focus on a comparison of quadrupeds, great apes, and humans with australopithecines. This group of fossil hominids lived in South and East Africa 2 to 4 million years ago and is considered to represent the first true bipeds. Their growth period was short and they became mature at an age of around 12 years, similar to chimpanzees.
In the sagittal view, the skeletons of a quadrupedal monkey, a great ape, and a human reveal very different body proportions (Fig. 2‑1a). The prehensile foot of macaques and gorillas contrasts with the human foot that is strongly adapted to provide support and propulsion during bipedal locomotion. The cranial capacity is around 400 to 600 cm3 in great apes, while the mean is 1400 cm3 in modern humans (Fig. 2‑1b). Facial prognathism is strong in great apes and the canines are very salient. The occipital foramen is positioned posteriorly in the cranial base, and the nuchal musculature is powerful. This cranial morphology results in a distinctive balance of the head on the cervical spine. Australopithecines have a cranial capacity of around 400 cm3, which implies an increased brain size relative to their small body size compared to great apes. The prognathism is reduced, the position of the occipital foramen is more anterior and the nuchal musculature is modified. The small canines are aligned with the other teeth.

2.2.1 Pelvis and Rib Cage in Frontal View
Pelvis
In frontal view, the marked reduction of the distance between the hip joint and the sacroiliac joint is clearly visible in humans. This played a key role in the evolution of bipedalism and was present in australopithecine pelves (Fig. 2‑1c). The approximation between the sacroiliac and hip joints reduces the rotational moments of the iliac segment transmitting trunk weight to the lower limbs, thus reducing muscular work to maintain the equilibrium. 2
Another important difference is the loss of the tail in hominoids (i.e., great apes and humans). Monkeys such as macaques possess a long tail with strong caudal muscles that originate on the sacrum. Their erector spinae muscles are inserted on the very developed iliac tuberosities (Fig. 2‑1d). In hominoids that have lost their tail, the erector spinae is inserted on the iliac crest and the sacrum. Humans differ from great apes by a broad sacrum with an extended insertion area of the erector spinae. The entire iliac blade of great apes and humans is considerably broader than that of monkeys. In the brachiating great apes, this provides an extensive area of origin for the latissimus dorsi and the quadratus lumborum, important muscles that support the trunk and pelvis during arm suspension (Fig. 2‑1d). In humans, the latissimus dorsi has a smaller area of origin, and the iliac crest can be divided in three sections that provide origin for the muscles balancing the trunk. From the lateral section of the iliac blade originate the oblique and transverse abdominal muscles, in the intermediate section latissimus dorsi and quadratus lumborum, and in the medial section the trunk erectors and gluteus maximus (Fig. 2‑2). While in great apes the origin of the gluteus maximus does not reach higher than the base of the sacrum, it has in humans a very high origin on the pelvis at the level of the iliac crest. The capacity of this muscle for trunk erection is thus far greater in humans than in great apes (Fig. 2‑2).

Humans also have an anteriorly curved lateral section of the iliac blades that modified the position of the lesser gluteal muscles. They thus became abductors on an extended hip while they were medial rotators on a flexed hip in great apes. This implies a different mechanism of lateral pelvic balance during bipedalism 3 (Fig. 2‑1).
The pelvis of australopithecines has laterally flaring and frontally oriented iliac blades similar to great apes with an extensive area of origin of the latissimus dorsi. 4 This implies a different hip abductor mechanism and thus a different mode of bipedalism than in modern humans. 3 On the other hand, they resemble the human condition in having a broad sacrum, and the gluteus maximus muscle originated from the posterior ilium. 4
Rib Cage
The rib cage is funnel-shaped in great apes and barrel-shaped in modern humans 5 (Fig. 2‑1c). This conforms to the wide and frontally oriented iliac blades in great apes and an anteriorly curved iliac crest in modern humans. The long and robust last rib of great apes provides an ideal attachment for the quadratus lumborum. Together with the reduced gap between the thorax and the iliac crest, this provides the necessary rigidity for the trunk in the forelimb-dominated locomotion of great apes. 6 Humans have a relatively wide upper thoracic aperture. This allows a greater respiratory efficiency and heavy breathing used for running while it reduces shoulder mobility. On the other hand, the lower thorax is narrower compared to great apes, and together with the longer lumbar spine this leads to a slim waistline. This plays an important role for our ability to run as it allows to exploit the greater flexibility of the lumbar spine. 6
Australopithecines such as the 3.2 million years old Lucy (Australopithecus afarensis, Ethiopia), also show a funnel-shaped thorax. Together with their cranially oriented shoulder girdle, this reflects their adaptation for climbing that they retained from the common ancestor with chimpanzees. 6 , 7 However, these characters make them unable to run long distances. 6 , 7
2.2.2 Sagittal View of Spine and Pelvis
Spine
Spinal Curvature
Modern humans have a double S-shaped vertebral column with four curvatures (i.e., a cervical lordosis, a thoracic kyphosis, a lumbar lordosis, and a sacral kyphosis). Great apes, in contrast, possess a weak kyphotic thoracolumbar curvature and a relatively short and stiff neck, which mainly results from a highly positioned shoulder girdle and massive pectoral muscles 6 (Fig. 2‑1, Fig. 2‑3). In association with a narrow sacrum and a short lumbar column there is an “entrapment” of the lumbar vertebrae between the iliac blades in great apes and thus a reduced lumbar mobility.

Number of Vertebrae
The length of the spinal regions and particularly of the lumbar region plays an important role for its mobility and capacity for lordosis. A long lumbar region is thought to be fundamental for having facilitated the adoption of bipedal locomotion in early hominids. The evolution of a human-like segmentation of the vertebral column is therefore of particular interest. In primates, the total number of precaudal vertebrae is surprisingly stable at 29. Quadrupedal monkeys have 12 to 13 thoracic vertebrae coupled with a long and flexible lumbar spine of six to seven elements and a short sacrum of three segments (Fig. 2‑1). 5 This allows them extensive flexion–extension movements needed for quadrupedal climbing, running, and springing. In contrast, apes show a stiffer and more rigid trunk adapted to forelimb-dominated locomotion. By integrating the last one or two lumbar vertebrae into the sacrum, their lumbar spine is accordingly reduced to five segments in gibbons and to four in orangutans, chimpanzees, and gorillas, while the sacrum became compensatorily elongated to four in gibbons and five elements in great apes on average. 5 A large percentage of chimpanzees and gorillas even possesses only three lumbar vertebrae and a correspondingly elongated sacrum. Such vertebral border shifts are associated with alterations in the expression of Hox genes that control the regional identity of vertebrae.
Another border shift occurred at the thoracolumbar junction in the ancestors of modern humans, leading to 12 thoracic and 5 lumbar segments on average, which makes the lumbar spine longer and more flexible than in great apes. This flexibility is a prerequisite for our lumbar lordosis, which cannot be achieved with the short and stiff lumbar spine of chimpanzees and gorillas. Different scenarios have been proposed for the evolution of this characteristic of modern humans: the short-backed scenario 8 suggests that the short lumbar column of chimpanzees and gorillas is primitive. The long-backed scenario proposes that a six-segment-long lumbar spine was retained in the ancestors of apes and humans. 9 This hypothesis was rejected by the discovery of new vertebral fossils that demonstrate the presence of five lumbar vertebrae in early hominids and not six as claimed before. 10 , 11 , 12 We therefore argue for an intermediate scenario with five lumbar vertebrae as the primitive condition in great apes and humans. Such a scenario is further supported by Oreopithecus, a great ape that lived 8 million years ago in Italy and also had five lumbar vertebrae. 13 The scenario has the advantage that the last common ancestor of chimpanzees and humans already had five lumbar vertebrae, which facilitated the adoption of lumbar lordosis and thus of bipedal locomotion.
Orientation of Facet Joints at the Thoracolumbar Junction
Early hominids seem to have differed from humans in the orientation of the facet joints at the thoracolumbar junction. In modern humans, the transition from thoracic to lumbar-like orientation is usually at T12, but in up to 40% of the population at T11. In contrast, all early hominid fossils show the transition at T11, 11 , 12 , 14 , 15 and it is likely that in the future hominid fossils will be discovered with the transitional vertebra at T10. Lumbar-like facet joints allow flexion–extension but restrict spinal rotation, while thoracic-like facet joints do not restrict mobility. The functional implication of a more cranially located transitional vertebra in early hominids might be related to a greater rotational stability of the trunk. 16 This might represent a climbing adaptation in early hominids, while a greater rotational capacity was needed in later Homo for running.
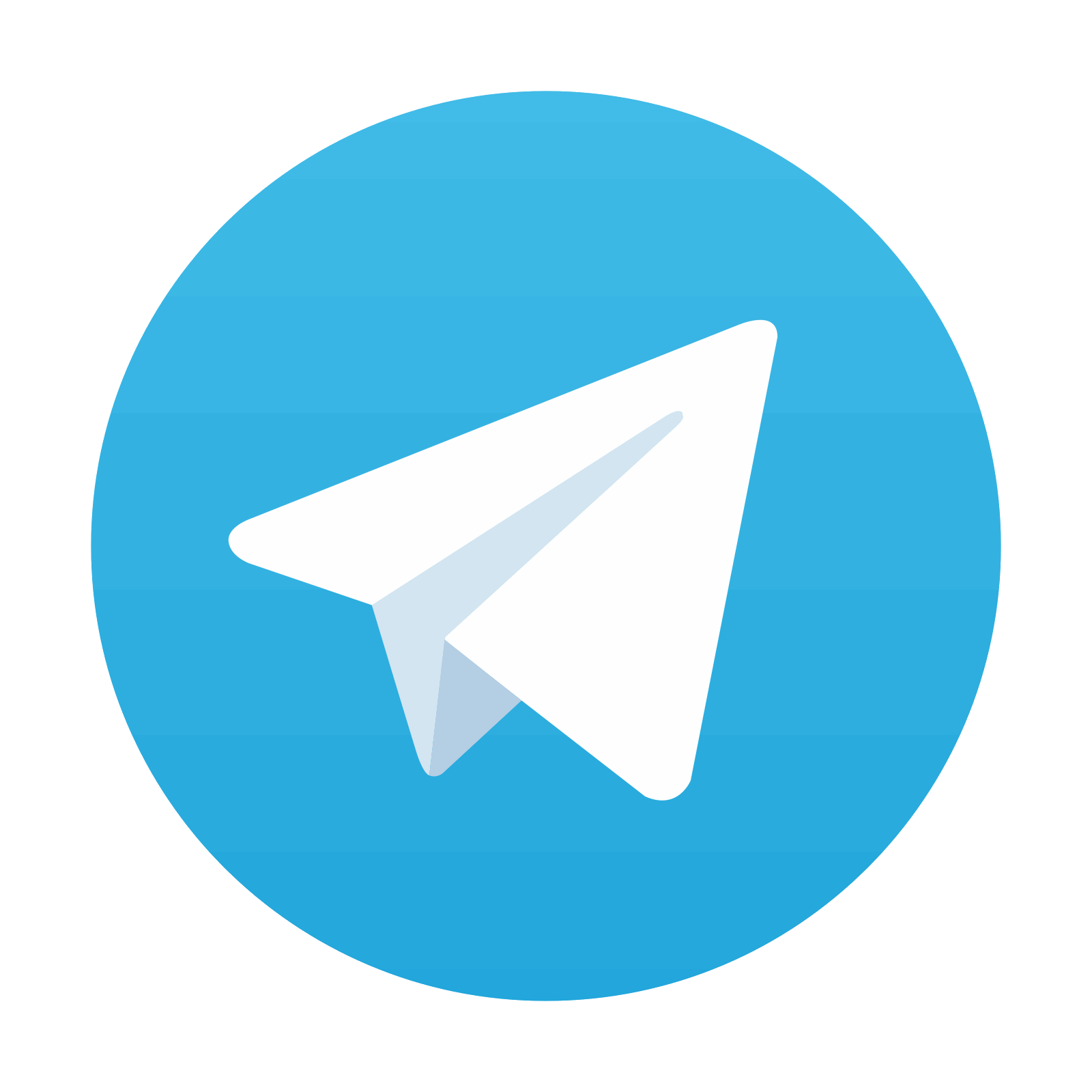
Stay updated, free articles. Join our Telegram channel

Full access? Get Clinical Tree
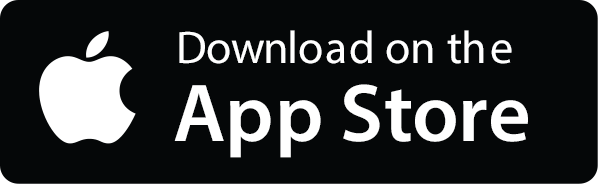
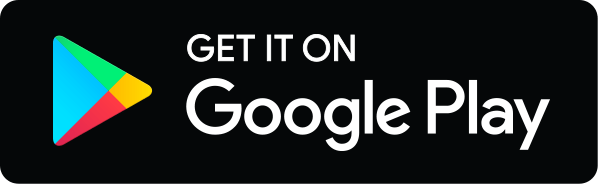
