7 Pathophysiology and Etiology of Intervertebral Disc Herniation Cary R. Templin, Yu-Po Lee and Steven R. Garfin Herniation of the intervertebral disc (IVD) is the most common cause of radicular leg pain in working-age adults.1 More than 250,000 elective lumbar spine operations are performed each year in the United States, and lumbar discectomy is the most common procedure.2 The absolute incidence of disc herniation, however, is probably underestimated given the high rate of asymptomatic disc herniations observed in magnetic resonance imaging (MRI) studies.3,4 Despite the common prevalence of disc herniation, much still remains unknown. It is unclear exactly what causes some herniations to present with pain and neurologic symptoms, whereas others are asymptomatic. In addition, what changes occur in a normal IVD that ultimately lead to a disc herniation? The purpose of this chapter is to review the structure and function of the IVD and its surrounding structures as they relate to the pathophysiology of disc herniation. The etiology of disc herniation, and the biomechanical aspects of disc herniation, both degenerative and traumatic, will also be reviewed. The state of the IVD is dynamic over its lifetime. Changes in the vascularity, nutrition, and cellular and molecular structure vary from early youth through adulthood. Physiologically, these changes seem to be patterned changes with age as the disc matures; however, “early degeneration” occurs quite frequently. This degenerative process leads to an increased inability of the disc to withstand physiologic loading. This can precipitate annular tearing and subsequent disc herniation. Disc degeneration, which commonly begins in early adulthood, may directly predispose the disc to herniation.5 Prior to the onset of the degenerative process, the nucleus pulposus (NP) is a homogeneous structure that serves a vital role in maintaining the mechanical function and structure of the disc. The healthy disc, with abundant hydration, largely hydrophilic proteoglycans, and a competent anulus, is ideal for absorbing complex loads early in life and in young adulthood. Beginning in the second decade, however, as the nucleus begins to lose its strongly hydrophilic proteoglycans, the disc becomes more solid and less adept at absorbing these loads and dispersing them to the surrounding structures.5–8 As a result of the degenerative process, the nucleus becomes heterogeneous and absorbs axial loads in a nonuniform manner, with an altered transfer of load to the anulus and vertebral end-plate. Loading moves from the more central endplate to the peripheral regions including the anulus (Fig. 7.1).9 This uneven distribution of forces across the endplate increases as the degenerative process progresses. Subsequently, increased compressive and shear forces are transmitted to the anulus, stressing its fibers.10,11 With continued strain on the annular fibers the result is fissuring and rupture of the annular complex. Stress concentration in the posterior anulus may predispose this region to disc prolapse.10 Fissuring and concentration of stress to the posterolateral region permit the migration of nuclear fragments to the periphery of the disc and herald the herniation of disc material. This migration of nuclear material may occur in a gradual, or stepwise, fashion.12 Although the nucleus becomes less homogeneously structured through changes in its collagen framework and proteoglycan structure, it may become fragmented. The fragmented nucleus, when placed under load, typically follows the path of least resistance through radial annular fissures and is prone to protrusion or extrusion through the anulus (Fig. 7.2).13 Mechanically induced herniation commonly occurs in patients around the age of 30 to 40 years.14 Such patients have degenerated discs that have maintained some degree of hydration. This explains why herniation is much more likely in this age group than in those who are older. Conversely, the disc in the older population has typically become hardened, solidified, and stiff, and is less prone to herniation. The inciting etiology of the herniation process is not fully understood but is thought to be multifactorial. Genetic inheritance, nutritional and metabolite supply, and mechanical forces are factors in the process. Recent studies have implicated genetic influences as a major factor in early-onset disc degeneration.15 Although physical loading in the form of lifting, torsional loading (e.g., lifting and twisting or golfing), and driving have been identified as risk factors for disc degeneration and subsequent herniation,16–18 some authors feel that these factors play only a minor role in the process in addition to genetic predisposition.19–21 Twin and family studies have shown a strong genetic predisposition to disc disease.20,22–26 Genotypical differences in collagen type IX have been shown to display advanced degenerative changes that may affect the crosslinking of type II collagen.27,28 Kawaguchi et al29 showed that multilevel disc degeneration occurred more frequently in a subset of patients with less genetic potential to form large aggrecan molecules. They did not, however, find an increased incidence of disc herniation in this population. This may be due to the loss of hydration and resultant increased stiffness of the disc.15 In addition, vitamin D receptor and matrix metalloproteinase-3 alleles have been shown to predispose certain populations to disc disease. Virtanen and colleagues have mapped a possible location of the gene responsible to chromosome 21q, a finding that may offer further insight into the genetic influences of disc degeneration.30 Fig. 7.1 (A) Depicts the transfer of force from an axial load in the central nucleus pulposus radially to the anulus fibrosus, where the force is directed circumferentially. Note the lamellar structure of the anulus with an alternating directional pattern of fibers, which resists multidirectional tensile forces. (B) With degeneration, the disc loses height, the nucleus has decreased potential for absorption and transfer of load, and the anulus bears more compressive loads. The cellular morphology of the disc is distinct, based upon the region of the disc. Cells of the anulus are largely fibroblast-like cells, which are aligned parallel to the collagen within the lamella. In the nucleus, the cells, which resemble chondrocytes, become less abundant, more rounded, and less structured, forming clusters. In the inner annular layers, the cells display characteristics of both the nuclear and annular cells and appear fibrocartilaginous in nature.14,31 Cells of the disc are responsive to mechanical loading and respond to both the type of load (i.e., compression or strain) as well as the direction and magnitude of the load.32 Based on their typical loading patterns, disc cells are responsible for the processes that maintain the extracellular matrix through continued degradation and regeneration. In the healthy disc, these processes are balanced and serve to maintain the disc.31 In the degenerate disc, however, the cells are unable to maintain and repair the degraded disc. Fig. 7.2 (A) A transverse section of a well-preserved disc showing the posterior extent of the nucleus pulposus, which lies in a more posterior position. The annular fibers are less abundant posteriorly and the thin posterior longitudinal ligament is also present. (B) A fairly well preserved disc specimen displaying a disc prolapse into the anulus. The material has passed through the inner layers but is still confined by the outer layers of the anulus. (C) A degenerated disc specimen with extrusion of nuclear material. Note the fragmented nucleus has passed beyond the outer fibers of the anulus. (From Resnick D. Degenerative disease of the spine. In: Resnick D, ed. Diagnosis of Bone and Joint Disorders, 4th ed. Philadelphia: Saunders, 2002:1434–1435. Reprinted with permission.) As the degenerative process takes place, there is alteration of the load pattern as areas of the anulus experience greater compressive loads, whereas a decompressed and heterogeneous nucleus is loaded irregularly. The response is an alteration of cellular signaling and thus in protein production. Herniated discs have been shown to contain increased matrix metalloproteinases (MMPs), nitric oxide, prostaglandin E2 (PGE-2), and interleukin-6 (IL-6).33 The cells of the nucleus and the anulus respond differently to variable loads depending upon the intensity, duration, frequency, and direction of loading.34–39 These cellular responses can affect the structure and composition of the extracellular matrix by stimulating the production of specific collagen types, as well as proteases and proteoglycans that alter the disc’s structural properties. Though paradoxical, the disc’s attempts to fortify its structure cause it to become stiffer and thus alter its loading mechanics. Alteration of loads can lead to annular disruption and eventual disc collapse. As the degenerated discs become structurally altered, they do not function optimally; hence, the NP becomes more susceptible to herniation. Degenerative discs have been shown to have a 25% decreased resistance to torsion, likely because of loss of tension in the collapsed nucleus and annular tissues.40 Mimura et al41 demonstrated that disc degeneration leads to increased laxity in motion in flexion-extension, axial rotation, and lateral bending. The anulus is also altered in degenerated discs, specifically posterolaterally, where the elastic modulus is found to be decreased in relation to the severity of the degeneration.42 It is reasonable to think that increased strain on the anulus is likely a large factor in producing the annular defects necessary for herniation. In a human cadaveric study Iencean found that herniation occurs at a significantly lower intradiscal pressure in discs with preexisting degeneration.43 They also found that discs with more advanced degeneration sustained even lower pressures prior to herniation than those discs with early degenerative changes. Positioning in flexion further reduced the pressure required for herniation by additionally stressing the already degenerated fibers. Herniations in this study were found to occur most frequently in a paramedian direction.43 Tsuji et al44 found that the paramedian or posterolateral aspect of the anulus had a less structured collagen arrangement, possibly predisposing it to annular tear and particulate herniation. Ohshima et al45 suggested that the morphology of lumbar disc herniation was dependent on the size and development of the posterior longitudinal ligament (PLL). In the lower lumbar spine the PLL is less developed, and posterolateral disc displacement with PLL rupture was common. Kuga and Kawabuchi46 studied the effects of repetitive flexion and axial rotation in the IVD of the rat, which is histologically similar in structure to the human disc. They found histologically that the anulus of herniated discs showed disorganization of the lamellar structure with rupture, and that herniation occurred despite absence of focal compression to the nucleus.46 Previously, in a similar study involving human discs in vitro, Gordon et al47 developed a reliable model of disc herniation by repetitive loading in flexion, axial rotation, and compression. Discs failed by annular protrusion of the nucleus through annular tears, supporting the hypothesis that disc prolapse is peripheral in origin, with the anulus fibrosus serving as the primary site of pathologic change.47 Flexion-extension and lateral bending of the spine generates alteration of steady-state pressure in the IVD (Fig. 7.3). Flexion causes migration of the nucleus to the posterior aspect of the disc, whereas lateral bending causes the nucleus to be displaced contralateral to the side of bending as the tissues move from the area of high pressure to low.48–50 Concurrently, the anulus, which is weaker dorsally than ventrally, is stretched, further lowering its ability to resist herniation.48 Axial loading of the spine in conjunction with flexion-extension and rotational moments combine to induce disc herniation.51 Torsional movements cause shifting of the spine’s center of rotation to the posterolateral portion of the disc, and torsional loads have been proposed to induce lateral herniation.52,53 Certainly, although in vitro studies suggest the anulus as the primary site responsible for herniation, it is the combination of nuclear degeneration coupled with these complex forces upon the anulus that account for herniation. As the nucleus degenerates and more stress is transferred to the anulus and its lamellar structure, the disc has a greater propensity for peripheral breakdown and rupture. Degenerated annular fibers have a 30% decrease in yield and ultimate stress when compared with normal discs.54 Reuber et al55 showed that degenerated discs subjected to combined compression and lateral bending were more prone to annular bulging than normal-appearing discs, showing their decreased structural integrity. Brinckmann suggested that degeneration and fragmentation of the NP with its separation from the endplate is a prerequisite for herniation.56 In his study, after radial sectioning of the anulus with the spine placed into complex loading positions, without fragmentation of the nucleus or separation from the endplate, herniation did not occur. Radial annular fissuring alone was not sufficient to cause herniation.56 Simunic et al, however, showed that healthy discs that were compressed while fully flexed and fully hydrated were highly susceptible to intraannular prolapse through an existing annular division.57 They found that flexion is a primary risk factor in disruption of the healthy disc nucleus. This is consistent with other literature in that flexion is a risk factor for herniation, primarily in discs that maintain their hydration, as in the young adult population.49,58,59 Simunic et al further contend that degeneration which occurs in herniated disc specimens may occur after the prolapse event, rather than before, suggesting that the nucleus may be healthy and well hydrated prior to herniation.57 Using finite element analysis, Schmidt et al60 found that combined moments in flexion-extension, lateral bending, and axial torsion led to higher stresses than pure moments alone, especially posterolaterally. Shear strain was maximal with lateral bend and flexion, whereas fiber strain was maximized with lateral bending with axial rotation.60 It has been previously shown in cadaveric testing that peak stresses develop at the posterior anulus lateral to the midline of the disc.61 It is a combination of moments to which the in vivo spine is most commonly subjected that likely predisposes to disc failure, especially posterolaterally. In addition to complex directional loading causing herniation, Wilder et al62 studied the disc’s response to static and vibration loading in the seated position. A 1-hour seated exposure to vibration was able to produce significant changes in the mechanical properties of the lumbar disc. Static loading in this position was able to incite sudden motion segment instability in flexion or lateral bending and could apply tensile impact loading on the posterolateral aspect of the disc. They also found that loading after sustained vibration was sufficient to cause tracking tears proceeding from the nucleus to the peripheral posterolateral anulus.62 This study emphasizes the role that both vibration and static loads may play in the etiology of disc herniation and helps to explain the increased incidence of disc herniation in truck drivers and others exposed to vibrational loads. Ninomiya and Muro63 studied the pathoanatomy of lumbar disc herniation using computed tomographic (CT) discography. They found that as the disc degenerates, radial fissures develop, and the path of herniation proceeds directly parallel to the sagittal plane at the central or paracentral portion of the disc (Fig. 7.4). Herniations following this path protruded to the dura and nerve roots. Herniations in the foraminal and extraforaminal region proceed obliquely to the sagittal plane at the posterolateral portion of the disc. Discography has shown that most herniations occurred in the inferior aspect of the disc.63 The development of radial fissures was proposed to occur with the confluence of posterior annular circumferential fissures and perinuclear annular fissures. Discograms can confirm the pathway of migration of nuclear material to the spinal canal (Fig. 7.5).
Pathophysiology
Genetic Influences
Disc Biomechanics
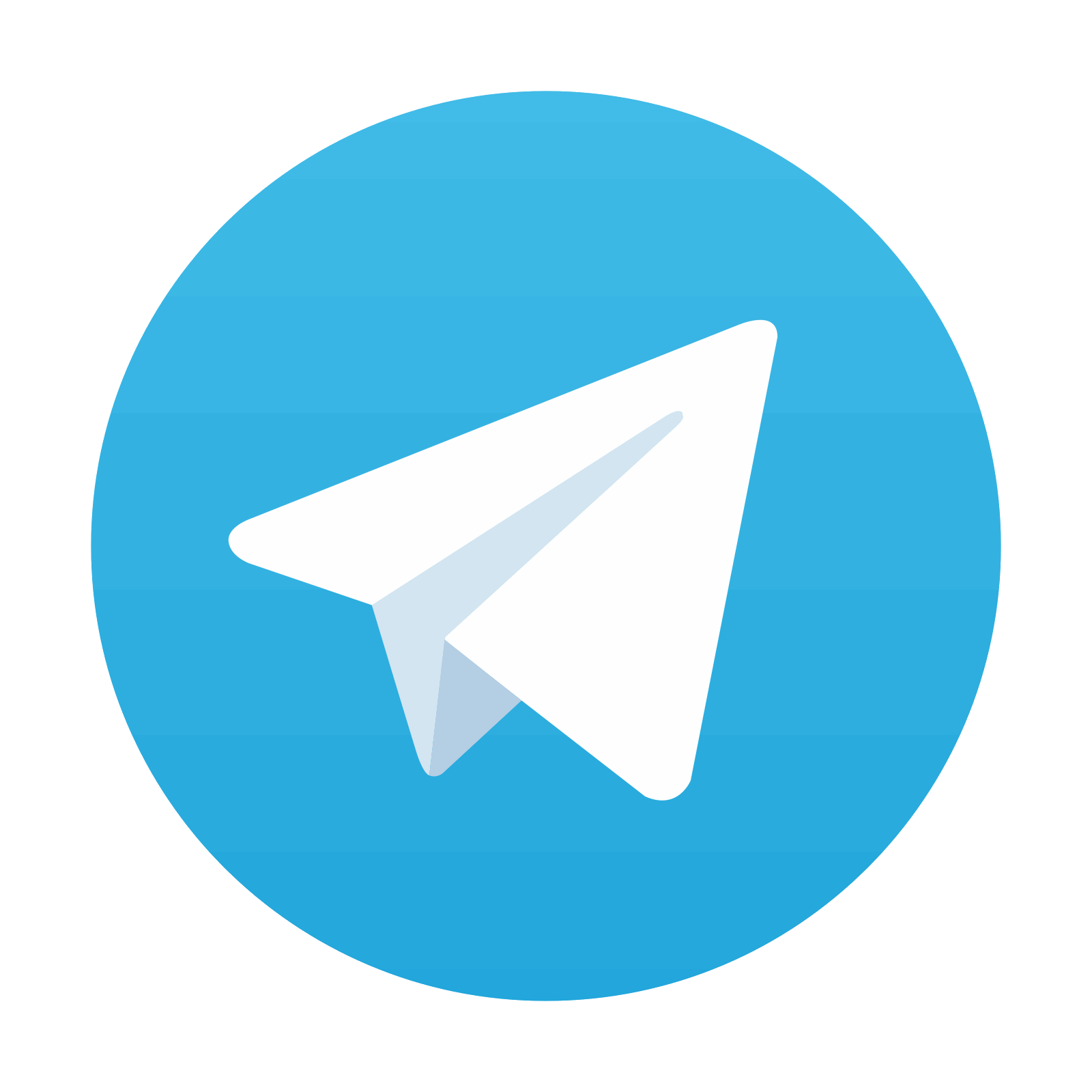
Stay updated, free articles. Join our Telegram channel

Full access? Get Clinical Tree
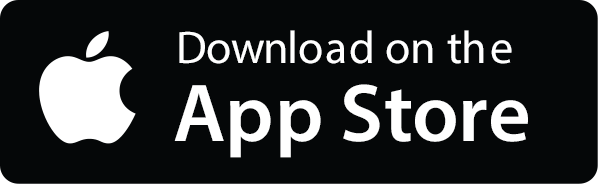
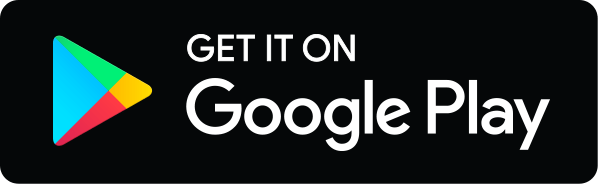