Reference
Country
No*
Samples
Main findings
Takahashi et al. [37]
JPN
15
CSF
bFGF ↑
Houkin et al. [38]
JPN
48
CSF
bFGF ↑ in bilateral MMD
Yoshimoto et al. [39]
JPN
38
CSF
bFGF ↑
Malek et al. [40]
USA
37
CSF
bFGF ↑
Hojo et al. [41]
JPN
20
Serum and STA culture
TGF ß-1↑
Soriano et al. [42]
USA
20
CSF and serum
VCAM-1, ICAM-1, E-selectin ↑ in CSF, not serum level
Kim et al. [43]
KOR
20
CSF
CRABP-1↑
Amano et al. [44]
JPN
29
Serum
α1-antitrypsin ↑
Nanba et al. [45]
JPN
39
CSF
HGF↑
Kang et al. [46]
KOR
20
Plasma
VEGF, PDGF BB, MMP-9, MCP-1, IL-1ß↑/ MMP-3, TIMP-1,2↓
Bernard et al. [47]
USA
7
Serum
D-dimer ↑in MMD and cardioembolic ischemic stroke
Jeon et al. [48]
KOR
77
CSF
CRABP-1↑in bilateral MMD in relation to decrease in basal collateral vessels

Fig. 2.1
A 30-year-old female presented with sudden onset right side weakness and dysarthria. Diffusion MR revealed acute infarction at Lt. Frontal anterior border zone (a). Angiography disclosed nearly total occlusion of the distal ICA occlusion on the left side (b). Single-photon emission computed tomography (SPECT) showed perfusion defect in the left frontal area with decreased basal perfusion in the resting state and decreased vascular reserve capacity in the acetazolamide challenge test (c and d).
Retinoid signaling pathway was reported to be related to MMD pathogenesis. Higher level of cellular retinoid acid-binding protein-I (CRABP-I) was noted in both pediatric [43] and adult MMD patients [48]. They hypothesized that CRABP-I inhibited the retinoid activity which resulted in neointimal thickening by enhancing growth factors. In particular, for adults, increased CRABP-I was related to typical bilateral MMD [48]. In addition, higher CRABP-I was related to a decrease in basal collateral vessel after bypass surgery. Accordingly, studies of retinoids as a therapeutic target for MMD are needed further.
2.7 Vascular Progenitor Cells
Circulating EPCs have been suggested as a pathogenic marker for MMD. Sugiyama et al. [53] directly stain the specimens of the distal ICA using antibodies of CD34, CD133, and vascular endothelial growth factor receptor-2 (VEGFR2) to localize the circulating EPCs in two adult MMD patients. Histopathological analyses showed that CD34− and VEGFR2+ cells were widely found in the thickened intima of the specimen. Nevertheless, the role of EPCS in MMD pathogenesis remains controversial. Jung et al. [54] reported that MMD patients revealed markedly decreased colony-forming unit (CFU) numbers on 7-day culture and elevated outgrowth cells during 2-month culture than control group. Similarly, decreased level of CD34+, CD133+, and KDR+ cells were noted in pediatric MMD, which were related to less tube formation and increased senescent-like phenotype [55]. Deregulation of retinaldehyde dehydrogenase 2 (RALDH2) of endothelial colony-forming cell was related to defective angiogenesis in pediatric MMD patients [56]. On the contrary, higher level of circulating EPCS was demonstrated in MMD patients [52]. Yoshihara et al. [57] found increased CD34+ cells which could attribute to neovascularization at sites of ischemic injury in MMD patients [57]. Ni et al. [58] also suggested that increased level of circulating CD34+, CXCR4+, and SDF-1α were related to MMD vasculogenesis. Heterogeneity of the ethnics, patients’ age, and experimental methods could lead to controversial results among the studies; therefore, further researches are required to yield the EPCs’ role in MMD pathogenesis [10].
MMD is characterized by the proliferation of SMC in the affected arteries. Accordingly, isolation of specific smooth muscle progenitor cells (SPCs) and its differentially expressed genes (DEG) analyses can be a dynamic model for MMD research [49]. Kang et al. [59] purified SPCs from peripheral blood in MMD patients (n = 25) and investigated DEGs. The SPC outgrowth cells in MMD patients revealed higher expression of alpha-smooth muscle actin, myosin heavy chain and calponin, and lower expression of CD 31 with more irregular and thickened tubules of SPCs than healthy control group. DEG analyses also showed increased expressed gene related to cell adhesion, cell migration, immune response, and vascular development in MMD SPCs. Further studies to identify relationship to specific change of SPCs in MMD pathogenesis are needed [60].
2.8 Recent Proteomic and Metabolomic Analyses
Two studies for identifying CSF biomarkers have been published using surface-enhanced laser desorption/ionization time-of-flight mass spectrometry (SELDI-TOF-MS) and metabolomics [61, 62]. Maruwaka et al. [61] reported increased three peptides of 4,473 Da, 4,475 Da, and 6,253 Da in 20 MMD patients (11 pediatric and 9 adult cases) by SELDI-TOF-MS. In particular, 4,473 Da peptide showed high correlation to postoperative angiogenesis and higher peak in younger MMD patients. Although precise role of 4,473 Da peptide remains unclear, they thought that 4,473 Da peptide could be related to anti-hypoxic effect or inflammation degree in MMD pathogenesis [61]. Jeon et al. [62] compared the CSF metabolites of adult bilateral MMD than those of unilateral MMD and atherosclerosis using a hydrogen-1 nuclear magnetic resonance spectroscopy. Bilateral MMD revealed higher level of glutamine than atherosclerotic stenosis. Considering the association between increased glutamine and intima-media thickness of the carotid and coronary artery disease [63], they postulated that increased glutamine in MMD could be related to more an abnormal SMC proliferation and intima thickness than atherosclerotic stenotic disease, although precise mechanisms is not well understood [62].
2.9 MMD in Association with Thyroid Disease
Several studies have illustrated MMD and concurrent autoimmune diseases, in particular thyroid diseases [64–66]. T-cell dysregulation [64] or increased sensitivity to the sympathetic nervous system of the vessel [65] has been suggested as pathomechanisms of the abnormal SMC proliferation and collateral vessel formation in MMD. Kim et al. [66] found that thyroid autoantibodies were significantly increased in MMD patients. They postulated that immune aberrancies related to thyroid autoimmunity may have a role in MMD pathogenesis. Recently, Chen et al. [67] reported that overall autoimmune diseases were significantly highly observed in unilateral MMD than bilateral MMD. Nevertheless, the actual pathogenic mechanisms of the autoimmune diseases in the development of MMD are still poorly understood. Accordingly, studies about autoimmune mechanism in MMD development, in particular the role of elevated thyroid autoantibodies in MMD development and progression, and its therapeutic target, are needed further [66, 68].
Conclusion
Although a better understanding of MMD has been achieved, the pathophysiology of MMD still remains fully understood. Heterogeneity of the ethnicity, patient age at presentation, and small sample size could lead to controversial results. Accordingly, high-throughput technologies in the effective biomarker for MMD, in particular disease severity or treatment outcomes in more homogeneous condition, are necessary.
References
1.
2.
3.
Kim T, Lee H, Bang JS, et al. Epidemiology of moyamoya disease in Korea: based on National Health Insurance Service Data. J Korean Neurosurg Soc. 2015;57:390–5.CrossRefPubMedPubMedCentral
4.
5.
6.
7.
8.
Kuroda S, Hashimoto N, Yoshimoto T, et al. Research Committee on Moyamoya Disease in J. Radiological findings, clinical course, and outcome in asymptomatic moyamoya disease: results of multicenter survey in Japan. Stroke. 2007;38:1430–5.CrossRefPubMed
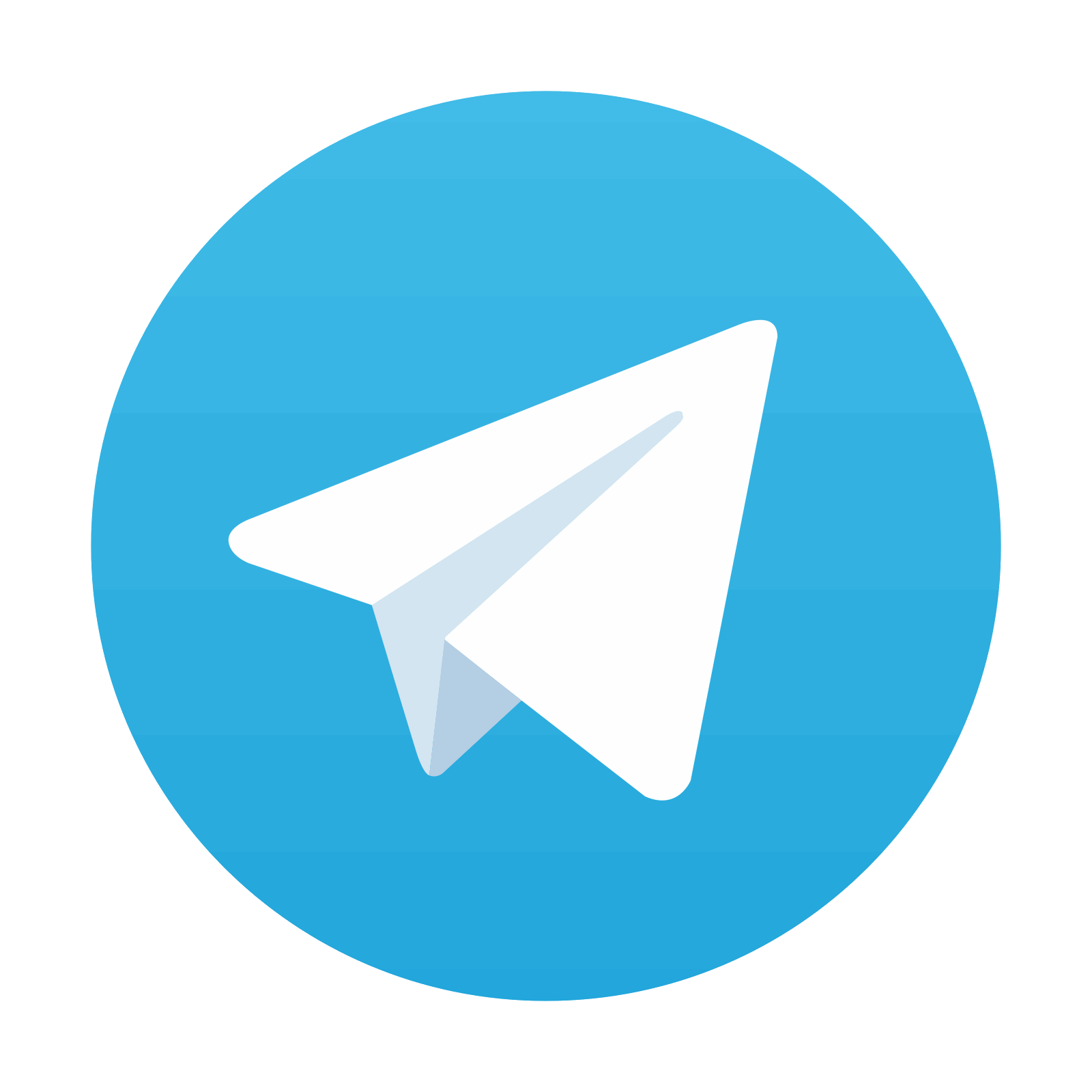
Stay updated, free articles. Join our Telegram channel

Full access? Get Clinical Tree
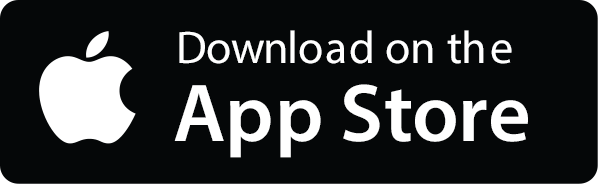
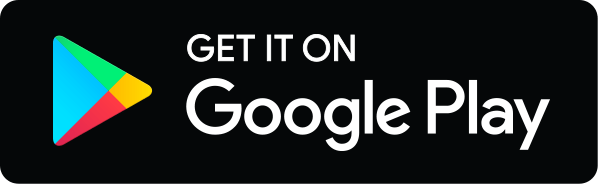