h1 class=”calibre8″>3 Pathophysiology of Spinal Cord Injury
Abstract
Spinal cord injury may result from traumatic or nontraumatic events. Treatment options for traumatic spinal cord injury depend on the pathophysiology of the spinal cord injury. In this chapter, we discuss the two pathophysiologic phases of injury to help determine appropriate treatment interventions. The secondary phase is further divided into four stages: the acute stage, the subacute stage, the intermediate stage, and the chronic stage. We briefly discuss the neuroprotective and neuroregenerative effects at the cellular and biochemical levels.
Keywords: spinal cord injury, pathophysiologic mechanism, phases of injury, neuroprotective effect, neuroregenerative effect
3.1 Introduction
Acute traumatic spinal cord injury (SCI) is a devastating sequela of physical trauma to the spinal cord. Depending on the severity of trauma, partial to complete loss of neurological functions will result at or below the level of the injury. It is estimated that of the 12,000 new patients diagnosed with SCI each year in the United States, 4,000 die before they reach hospital while 1,000 die during hospitalization. 1,2,3,4 Therefore, the sequelae of SCI extend beyond affecting patients and their families and carries a substantial socioeconomic impact globally. 5,6,7 Despite the demonstrated value of supportive care, particularly in reducing mortality due to SCI, 8 the natural history of irreversible paralysis due to SCI continues to burden both patients and health care systems. 5,9 Therefore, ongoing research efforts have attempted to investigate potential neuroprotective and neuroregenerative interventions through a better understanding of the pathophysiological events involved in SCI on both the macroscopic and the molecular scales. The purpose of this chapter is to elucidate the pathophysiologic mechanisms implicated in SCI. Detailed discussion of the clinical presentation as well as management options for SCI has not been provided as that is the focus of the other chapters in this text.
3.2 Epidemiology
The annual incidence of SCI is estimated to be 12 to 53 cases per million population individuals in developed countries and 15 to 40 cases per million individuals worldwide. 8,10,11,12 In the United States, 12,000 new cases of SCI are recorded each year. 7,13 Cervical spine injury is the most common anatomical location for injury and associated with the greatest morbidity and cost. Of all SCI cases, 54 to 75% occur in the cervical spine, while 15% are estimated to occur in the thoracic spine, and 10% in the lumbar spine. 5,7,8,14
Analysis of National Spinal Cord Injury Database (NSCID) records revealed important trends on the incidence and prevalence of SCI. Increasing mean age at injury has been observed from a mean of 28.7 years between 1973 and 1979 to 38.0 years between 2000 and 2003. 12 In addition, the prevalence has markedly risen in the elderly population from 4.7% between 1973 and 1979 to almost 11% between 2000 and 2003. 12 Conversely, the prevalence has fallen for children between 0 and 15 years from 6.4 to 2% during the same period. 12 The increasing incidence of SCI among the elderly population can have clinical implications on the management of this subset of patients with specific needs and age-related co-morbidities. However, young male patients continue to be the most often impacted by SCI, the majority of whom are healthy and at their physical peak. 7,14
Etiologically, motor vehicle accidents are the dominant cause of SCI accounting for 50% of all causes of injury in all age groups. Alcohol consumption is linked to 25% of cases. 8,9 In addition, falls are the second most common cause of SCI in all age groups and the most common cause for patients who are aged 60 years and more. 9 Furthermore, the overall incidence for SCI from falls and work-related injuries has consistently increased over the past 30 years and accounts for 30% of all SCIs. 12 Other causes for SCI include violence which accounts for 11% of injuries and sports-related injuries which accounts for 9% of all SCIs. 8,9
SCI is associated with significant mortality and morbidity with a mortality rate ranging between 4 to 17% during the initial admission. 2 In addition, morbidity is largely affected by the high incidence of cervical spine injuries among patients with acute SCI with the most common injury pattern being incomplete tetraplegia (34.5%), followed by complete paraplegia (23.1%), complete tetraplegia (18.4%), and incomplete paraplegia (17.5%). For these patients, quality of life is determined by pain, extent of loss of visceral functions, and hand functions. 15
3.3 Pathological Phases of Injury
The pathogenesis of acute traumatic SCI is a biphasic process. 16,17,18 In the primary phase, immediate damage is caused by the initial physical trauma leading to local deformation of the neural elements and disruption of the local circulation. This is followed by a secondary phase of progressive injury caused by an interplay of complex biochemical, cellular, and systemic reactions to the primary insult. 16,19,20 Although the changes in the secondary phase aim to provide a neuroprotective effect, the true end result is progressive neural destruction that can continue for several weeks or months following the initial trauma. Understanding the order and timing of pathophysiologic changes in the secondary phase may help guide different treatment regimen instituted in the context of acute SCI. Historically, the model of primary and secondary phases of injury was first demonstrated in animal studies. 21 Since then, multiple mechanisms have been uncovered, some of which have been implicated in the secondary phase of acute SCI. 18,22,23,24,25,26,27,28,29
3.3.1 Primary Spinal Cord Injury
In this phase, primary injury forces can result from acute compression, shearing, laceration, distraction, or acceleration-deceleration, all of which can subject the spinal cord to contusion and persistent compression. 30,31 Common clinical scenarios include burst fractures, flexion–distraction injuries, and penetrating missile injuries. Anatomical disruption can lead to complete spinal cord transection, but more commonly partial disruption is encountered. 7 In this situation, spared neural axons may traverse the damaged area. However, these axons are frequently demyelinated and are subsequently dysfunctional. 32,33,34,35,36 Biochemically, this phase is marked by local elevation of proinflammatory mediators including tumor necrosis factor-α (TNF-α), interleukin 1-beta (IL-1β), and cytotoxic levels of glutamate due to cellular disruption. 37 The injury process involved in this phase can last for up to two hours following the initial trauma. 37
3.3.2 Secondary Spinal Cord Injury
A growing body of evidence has demonstrated that a cascade of complex cellular and biochemical reactions follows the primary phase of injury and leads to progressive and delayed local neural elements destruction. 18,20,22,23,24,25,26,33,38,39,40 Multiple studies have attempted to identify key processes that may be targeted by neuroprotective interventions to improve clinical outcomes. Chronologically, the secondary phase can be divided into the early acute stage (first 48 hours), the subacute stage (2 weeks), the intermediate stage (6 months), and the chronic stage (beyond 6 months). 41 Each stage is mediated by specific pathological mechanisms. Ionic dysregulation, apoptosis, vascular mechanisms, and free radical generation are among the most widely accepted pathways implicated in the pathogenesis of this phase. 8,18,25,26
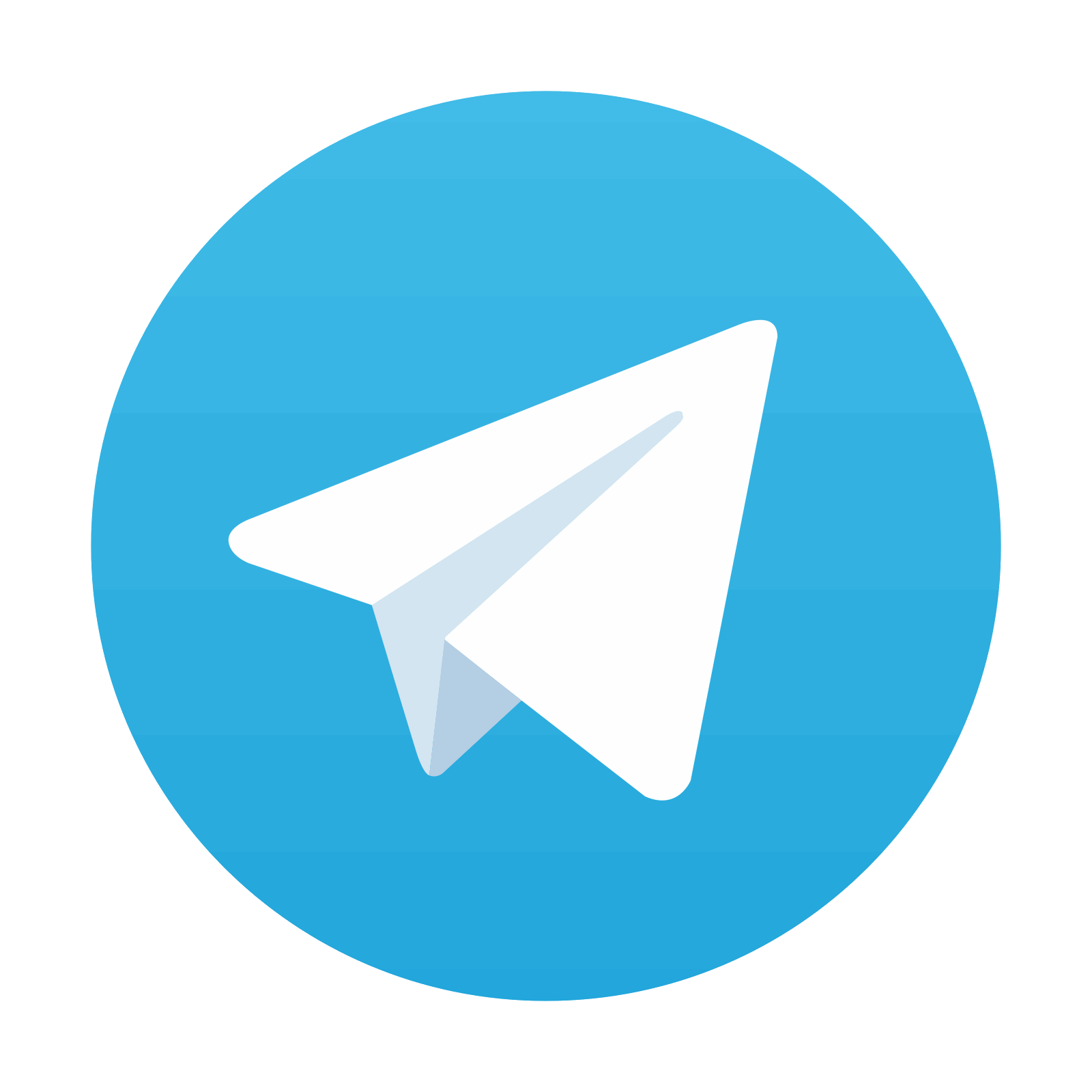
Stay updated, free articles. Join our Telegram channel

Full access? Get Clinical Tree
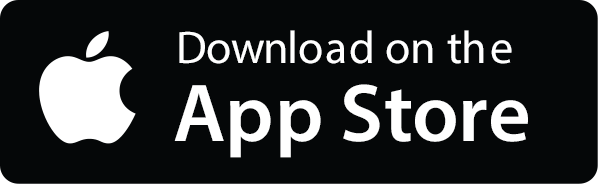
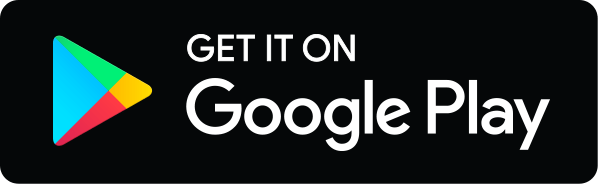