Summary of Key Points
- •
The thoracic spine has unique biomechanical and physiologic features.
- •
The thoracic spine is a relatively rigid transition zone between the more mobile cervical and lumbar regions.
- •
The thoracic spinal cord is less prone to dynamic compression than the cervical spinal cord.
- •
The thoracic spinal cord is more prone to vascular compromise than the cervical spinal cord.
- •
The narrow spinal canal and limited dorsal mobility make the thoracic spinal cord vulnerable to a variety of pathologies.
- •
Imaging techniques used to assess thoracic myelopathy vary based on etiology.
- •
Prognosis is generally favorable for most causes of thoracic myelopathy.
Similar to cervical myelopathy, thoracic myelopathy can cause severe and debilitating neurologic injury. However, unique anatomic and physiologic characteristics of the thoracic spinal column and spinal cord distinguish it from the better studied and better understood cervical myelopathy. An understanding of the unique combination of physiologic kyphosis, narrowed spinal canal, tenuous blood supply, and altered biomechanical forces have implications for diagnosis, treatment, and prognosis.
Biomechanical Anatomy of the Thoracic Vertebral Column
The thoracic region features the typical attributes found throughout the spine. Each spinal segment is composed of a load-bearing vertebral body and intervening intervertebral disc. On each respective side of the vertebral bodies and discs, the vertebral column is bound by an extension-limiting anterior longitudinal ligament and a flexion-limiting posterior longitudinal ligament. Laterally and posteriorly, the vertebral canal is enclosed by a vertebral arch with prominent transverse and spinous processes. Superior and inferior articular processes feature synovial facet joints between adjacent vertebrae.
The anatomy of the thoracic spine allows less flexibility than other spinal regions. Its flexibility is limited by its articulations with the ribs and, indirectly through them, the sternum. Its intervertebral discs are relatively thin. The spinous processes are long and, in the midthoracic spine, overlap the segment below. Its facet joint capsules are shorter and less lax than those in other regions. The facet joints are oriented relatively coronally, thus facilitating rotation (35 degrees) and, to a lesser degree, truncal lateral flexion (20 degrees). Forward flexion and extension are limited (35 and 25 degrees, respectively) compared with the lumbar spine (50 and 35 degrees, respectively). Consistent with its reduced flexibility, the vertebral canal is narrowest in the thoracic region.
The thoracic spine has been conceptually modeled as consisting of three longitudinal columns, two of which should be intact for stability. Spinal fractures can be intuitively classified by disruptions to these three columns. The first column consists of the anterior portions of the vertebral body and annulus fibrosus, and the anterior longitudinal ligament. The middle column consists of the posterior portions of the body and annulus fibrosus, and the posterior longitudinal ligament. The posterior column consists of the facet joints, laminae, interleaving ligamenta flava, and interspinous and supraspinous ligaments.
Because the facet joints are aligned with the coronal plane in the thoracic spine, thoracic vertebrae are unlikely to dislocate without concomitant fracture. Consequently, greater force is generally required to destabilize the thoracic spine in comparison with the cervical region with its axially oriented facet joints. When fracture with displacement of bone fragments does occur in the thoracic spine, the narrower vertebral canal renders the spinal cord more vulnerable to injury.
Histopathology of Spinal Cord Injury
Following spinal cord contusion, petechial hemorrhages often appear in the central gray matter, followed within 15 minutes by edema in the white matter immediately bordering the gray matter. The edema appears to follow a radial gradient, beginning in the internal white matter and extending toward the pial surface to an extent that depends on the magnitude of the force causing the injury. In cases of mild contusion, this edema may be limited to gray matter. In as few as 6 hours following injury, necrosis and cavitation may appear in the affected tissue.
Within 1 hour in affected white matter, periaxonal spaces become dilated, axoplasmic transport appears dammed, and myelin sheaths begin to disintegrate. It is during this time that retraction bulbs begin to appear within white matter. In the following days, Wallerian degeneration and argyrophilia appear in the fiber tracts distal to the site of lesion. At the site of the lesion, glial scarring develops, characterized by astrogliosis and the deposition of chondroitin sulfate proteoglycans that colocalize with abortive axonal regeneration. In more severe cases, a central fibrotic scar often appears, increasing the barrier to axonal regeneration.
Pathophysiology
Unlike cervical spondylotic myelopathy, the pathophysiology of thoracic myelopathy is less well understood. The pathophysiology of thoracic myelopathy is multifactorial and is unique due to the blood supply of the bony anatomy and the shear and stress forces present in the thoracic spine. The anatomy of the thoracic spine is unlike that of the cervical or lumbar spine. The thoracic spine is a relatively rigid transition zone between the mobile cervical and lumbar regions; its unique stability is the result of the surrounding thoracic rib cage. It is well known that the range of motion of the cervical spine can contribute to cervical myelopathy. This is known as dynamic compression. The combination of the splinting effect of the thoracic rib cage and the resistance of the vertically oriented thoracic facets to flexion loads lessens the potential for dynamic compression and instability. This stability limits the potential for intervertebral disc injury in the thoracic spine compared with the cervical or lumbar spine. Stretch and shear forces are another common contributor to the development of myelopathy. The dentate (denticulate) ligaments, which run longitudinally between the spinal cord and the nerve roots, limit posterior displacement of the spinal cord within the canal, thus making the thoracic spinal cord more sensitive to ventral compression from anterior disc and bone prominences. Furthermore, the natural kyphosis of the thoracic spine causes the spinal cord to lie directly on the posterior longitudinal ligament and the posterior aspects of both the vertebral bodies and the discs in this region. Therefore, thoracic disc herniations, ossification of the posterior longitudinal ligament (OPLL), tumors, or any other mass may cause significant ventral compression of the thoracic spinal cord. These shear forces are further magnified by local tethering of spondylotic bars, aberrant ossification of the posterior longitudinal ligament (PLL), and ligamentum flavum, as well as ligamentous redundancy. The combination of kyphosis with local tethering and limited dorsal migration causes stretch and shear forces to reach significant levels. These shear forces lead to axolemmal blebs, disruption of membrane associated proteins, and loss of microtubule structure leading to disrupted axonal transport. They also cause thinning of the myelin sheath and impaired nerve conduction.
The vascular supply to the thoracic spinal cord is also unique and contributes to its pathophysiology. The blood supply of the thoracic spinal cord is more variable and tenuous than that of either the cervical or the lumbar region. The thoracic spinal cord receives its intrinsic blood supply from the combination of one anterior and two posterior longitudinal arterial trunks. The midline anterior arterial trunk gives off central penetrating arterial branches, which supply most of the thoracic spinal cord. These vessels are smaller in caliber and fewer in number than those in the lumbar and cervical regions. An additional extrinsic arterial supply comes from vessels that arise variably from the segmental posterior intercostal arteries. Like the central arterial branches, these arteries are smaller in caliber and fewer in number than in other regions of the spine. Older studies have proposed that compression of radicular feeders, venous engorgement, and the elongation of the laterally oriented terminal branches of the anterior spinal artery all lead to decreased perfusion of the spinal cord. Although ischemia and compression alone have been discredited as being the sole root cause of myelopathy, they do contribute to the development of myelopathy. Local ischemia, although not the direct cause of injury, renders axons more susceptible to secondary injury from other processes, including stretch and shear forces.
Prevalence, Evaluation, Treatment, and Prognosis
The evaluation of thoracic myelopathy involves thorough investigation, due to the numerous distinct etiologies. To determine the biomechanical etiology of deformative stress precipitating myelopathy, a comprehensive neurologic examination, combined with proper imaging, is essential.
Several physical examination findings are highly suggestive of myelopathy: positive Babinski sign, upper motor neuron signs (altered tonus, weakness, spasticity, hyperreflexia), incontinence, and sexual dysfunction. Dermatomal involvement is extremely useful for lesion localization, especially when imaging is inconclusive or does not match symptomatic presentation. Magnetic resonance imaging (MRI) is the preferred imaging modality for evaluation of thoracic myelopathy, as it is highly sensitive, noninvasive, and provides the highest resolution of the spinal cord. In many cases, augmenting MRI with a computed tomography (CT) myelography can be useful for elucidating osseous features and abnormalities. Additionally, transcortical magnetic stimulation (TMS) has demonstrated diagnostic utility in detecting the presence of spinal cord myelopathy via measurement of central motor conduction time (CMCT).
Imaging characteristics in various causes of thoracic myelopathy are outlined next. Physical examination findings of each are highly dependent on the location and severity of deformative stress on the spinal cord, and as such there is considerable overlap between the pathologies covered.
Disc Herniation
Disc herniations, although prevalent in other regions of the spine, are relatively rare in the thoracic spine. Thoracic disc herniations make up approximately 1% of all disc herniations, with a prevalence of approximately 1 per 1,000,000 patients per year. Although MRI is highly sensitive in identifying thoracic disc herniation, its usefulness in determining clinical correlation is limited by its relatively high false-positive rate. For this reason, Dietze and Fessler have recommended CT myelography to characterize thoracic disc herniations. Features of CT myelography often include extradural thecal sac defect adjacent to the disc herniation and soft-tissue density mixed with calcification indicating the presence of vertebral end plate osteophytes. Of note, on physical examination in the patient with disc herniation, pain or radicular symptoms can often be elicited via palpation or percussion of posterior spinal elements. Otani and colleagues also have reported a high incidence of ossification of the ligamentum flavum (OLF) in association with thoracic disc herniation (see the following section, “ Ligamentous Ossification ”). Various anterior and posterolateral surgical approaches including minimally invasive techniques have been developed to provide spinal cord decompression, with generally favorable postoperative prognosis.
Ligamentous Ossification
OPLL and OLF, both rare causes of thoracic stenosis and associated myelopathy, are mainly associated with elderly patients of East Asian descent. OPLL occurs more frequently in the cervical spine but can occasionally extend into the thoracic spine. OLF, however, occurs mostly in the thoracic spine. In each, the choice of diagnostic imaging is sagittal T2-weighted MRI showing spinal cord compression caused by low signal intensity lesions in the anterior (OPLL) or posterior (OLF) aspects of the spinal canal. Signal intensity is also low on T1-weighted MRI scans. Axial CT imaging is used to make an exact diagnosis and can be used to classify the progression of ligament ossification. Prompt decompression of the affected levels is critical to a favorable outcome, as prolonged cord compression is associated with poor functional recovery.
Trauma
Spinal fractures that disrupt the continuity of the spinal canal can lead to spinal cord injury via spinal cord compression, stretch, and shearing. Due to the narrowing of the spinal canal in the mid to upper thoracic spine, the majority of thoracic spinal cord injuries occur at these levels. The resulting neurologic injury varies depending on the spinal level and the severity of the spinal column disruption.
In the trauma patient with myelopathic symptoms, CT is the standard to evaluate vertebral fracture and retropulsed fragments that may involve the spinal canal and cord. Anteroposterior and lateral radiographs are a useful adjunct in assessing overall spinal alignment. X-ray and CT are preferred for initial analysis due to the speed in which they can be obtained; however, MRI remains the most sensitive for detection of spinal cord compression, traction, or injury resulting in myelopathy.
Neurologic deficit is classified as complete or incomplete, with complete injuries having very poor prognosis for recovery and incomplete injuries more likely to show significant functional recovery over time. Progressive neurologic deficit is an indication for prompt decompression of the spinal canal.
Tumor, Cyst, Abscess
Compression of the spinal cord due to the presence of an epidural abscess, tumor, or intradural (arachnoid) cyst is addressed with varied treatments and prognoses, depending on the individual scenario. Various tumors, cysts, and abscesses that may cause thoracic myelopathy are best visualized through sagittal and axial MRI. Intensities will vary on T1- and T2-weighted MRI depending on the composition of the lesion. In general, abscesses and cystic lesions will appear as well-circumscribed, hyperintense masses on T2, and will not enhance on T1 with contrast. Tumors are more likely to enhance with contrast; however, they may not enhance, and their baseline characteristics on T1- and T2-weighted MRI can vary depending on the tissue origin of the neoplasm.
Spinal or paraspinal abscesses have traditionally been addressed with both surgical decompression to prevent prolonged cord compression as well as antibiotic therapy. Evidence suggests, however, that antibiotic therapy alone may be equally as effective in some populations. The surgical approach varies greatly with the location of the abscess. Tumors of the thoracic spine are treated with various combinations of chemotherapy, radiation therapy, and surgical resection, with consideration given to the origin, location, and size of the tumor. Intradural spinal cysts (arachnoid cysts), which occur most commonly in the thoracic spine, are addressed with surgical excision if they are symptomatic. However, fenestration or shunting may be preferable for large cysts spanning multiple levels, anteriorly located cysts, or recurring cysts.
Dural Arteriovenous Fistula
Venous congestive myelopathy associated with dural arteriovenous fistula (AVF) often presents as a linear intramedullary lesion with high signal intensity on sagittal T2-weighted MRI. Clinically, venous congestion is quite heterogeneous, with features common to a variety of spinal pathologies—including paraparesis and sensory disturbance—making definitive diagnosis difficult. Diagnosis is somewhat more straightforward if strokelike events occur as the initial symptoms, in which case prompt angiography may be indicated to elucidate vascular abnormalities responsible. Once the diagnosis has been made, treatment options include microsurgical exclusion or endovascular embolization with a generally favorable prognosis.
Spinal Deformity
Patients with kyphotic deformity of the spine often report myelopathic symptoms. However, isolated scoliotic deformity is only rarely associated myelopathy. This may be due to changes in intramedullary pressure caused by kyphosis but not scoliosis. In the scoliotic or kyphotic patient, imaging assessment begins with static standing anteroposterior and lateral radiographs of the whole spine, iliac crests, and hip joints, in order to assess the degree of spinal deformity. MRI is then used to evaluate for spinal canal stenosis and spinal cord compression. CT myelography can be used in place of or as an adjunct to an MRI and offers the advantage of better visualization of rotational deformity and bony anatomy. Spinal fusion is the preferred strategy for improving spinal alignment and relieving symptoms, with specific surgical strategies varying greatly with each clinical scenario.
Spinal Myelopathy Secondary to Intrathecal Catheterization
Intrathecal catheter-associated inflammatory masses (CIMs) can form near the outlet slits of intrathecal catheters in patients with implanted drug pumps with a prevalence rate as high as 3%. These masses can be asymptomatic or can cause spinal cord compression and myelopathy. Though CIMs are often referred to as granulomas, they are not true granulomas as they lack characteristic giant cells. CIMs can best be detected using sagittal T2-weighted MRI or CT myelogram, if the spinal cord medical apparatus prevents the use of MRI. Early resection of symptomatic CIMs can improve the neurologic deficit incurred. Simple removal of the intrathecal catheter or de-activation of the pump may be used in selected cases—with subsequent resolution of the of the CIM, which presumably occurs as a result of the eliminated exposure to the inflammatory inducing effect associated with the preservative included with the infused drug.
Although rare and difficult to detect, myelopathy can also be caused by an unknown mechanism by intrathecal chemotherapy used to treat acute lymphoblastic leukemia (ALL). Dermatomal somatosensory-evoked potential (DSEP) conduction abnormalities correlate well with the clinical level of thoracic myelopathy in these patients. Sagittal and axial T2 and short T1 inversion recovery (STIR) MRI with gadolinium enhancement can provide visualization of hyperintense lesions; however, MRI early in the clinical course may be normal.
Idiopathic Spinal Cord Herniation
Idiopathic spinal cord herniation, though extremely rare, occurs most frequently in the thoracic spine, causing thoracic myelopathy. This disease presents the unique feature of an anterior or posterior shift in the spinal cord on MRI, indicating herniation with potential tethering. CT myelogram often shows no subarachnoid space anterior (with a ventral herniation) or posterior (with a dorsal herniation) to the spinal cord. Surgical intervention leads to improved neurologic outcome in the majority of patients, whereas patients treated with conservative management have unchanged neurologic outcomes.
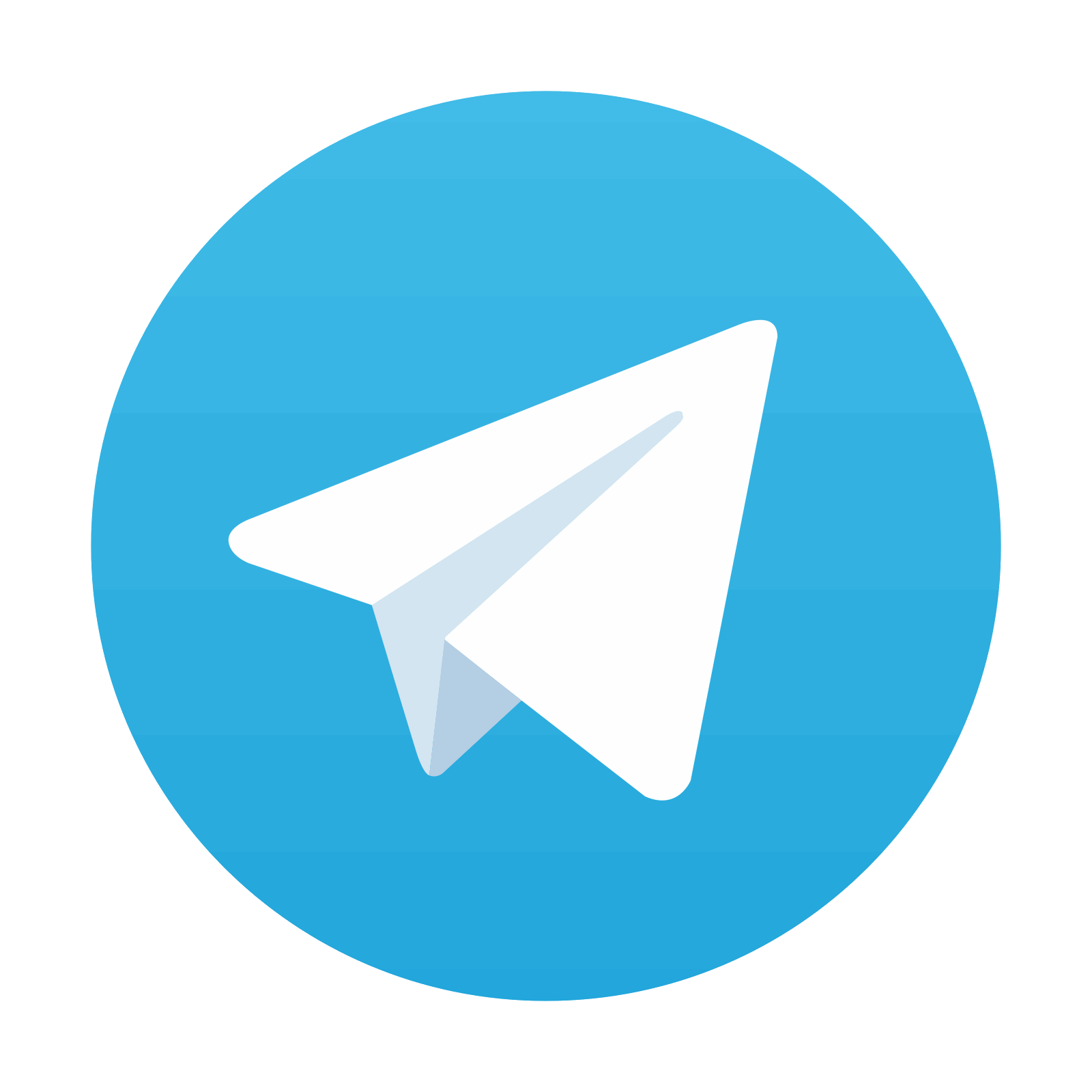
Stay updated, free articles. Join our Telegram channel

Full access? Get Clinical Tree
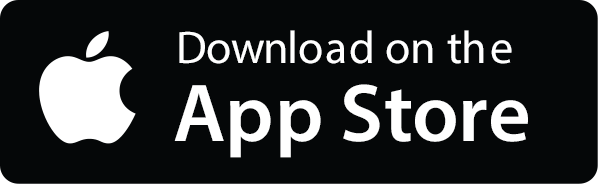
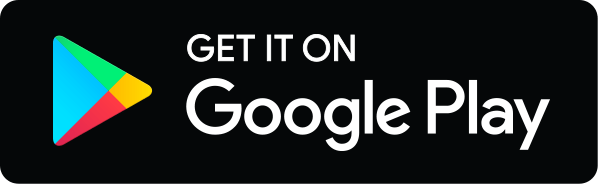