© Springer International Publishing Switzerland 2016
Richard L. Applegate, Gang Chen, Hua Feng and John H. Zhang (eds.)Brain Edema XVIActa Neurochirurgica Supplement12110.1007/978-3-319-18497-5_29Signaling Pathway in Cerebral Vasospasm After Subarachnoid Hemorrhage: News Update
(1)
Department of Neurosurgery, The First Affiliated Hospital of Soochow University, 188 Shizi Street, Suzhou, 215006, P.R.China
Keywords
Signal transductionSubarachnoid hemorrhageCerebral vasospasmAneurysmsIntroduction
Cerebral vasospasm (CVS) after subarachnoid hemorrhage (SAH) caused by ruptured intracranial aneurysms is a major cause of death and disability [12]. After SAH, signs of CVS can be revealed in the angiograms of 70 % of patients, whereas the remaining 30 % of patients show clear clinical manifestations and symptoms of CVS [8]. With the rapid development of molecular biology and molecular immunology techniques, studies on CVS have gradually moved to the level of molecules and genes, and the importance of deciphering the relevant signal transduction pathways for understanding the mechanisms of CVS has become clear [20]. This chapter briefly reviews current progress in the studies of the different signal transduction pathways relevant to CVS after SAH.
Mitogen-Activated Protein Kinase (MAPK) Signaling Pathway and Its Regulation
MAPKs are a group of intracellular serine (Ser)/threonine (Thr) protein kinases commonly found in a variety of organisms. MAPKs maintain their active and inactive states typically via the phosphorylation and dephosphorylation of specific sites [17]. Human MAPKs are divided into three subfamilies: ERK, JNK, and p38 MAPK.
JNK/SAPK Pathway
C-Jun N-terminal kinases (JNKs) can be divided into three subtypes: JNK1, JNK2, and JNK3. The MAPK kinases MKK4 and MKK7 are the kinases upstream of JNK. MKK7 activates JNK specifically [26], whereas MKK4 can activate JNK1 and p38.
p38 MAPK Pathway
MKK3, MKK4, and MKK6 are the kinases upstream from p38 MAPK. The observation that MKK3 and MKK4 are upstream activators of both the p38 and JNK pathways suggests that there may be crosstalk between these two pathways. p38 MAPK is also involved in the activation of NF-κB activation.
Raf/MEK/ERK Signaling Pathway
Raf Kinase Family
The mammalian Raf family contains predominantly proteins (A-Raf, B-Raf, and C-Raf [Raf-1]). Raf-1 is expressed in almost all tissues, A-Raf is expressed mainly in the genitourinary tissues, and B-Raf is expressed in the nerves, testis, spleen, and hematopoietic tissues.
Downstream Regulation of Raf Kinases
Ras kinases are activated mainly by Ras and other small GTPases. All Ras subtypes can interact with Raf [21]. Activated Raf transduces the activation signal mainly via the phosphorylation of two dual specificity MAPKKs: MEK1 (MKK1) and MEK2 (MKK2) [13].
Raf/ERK1/2 Pathway
The substrates of MEK1 and MEK2 are ERK (p44 MAPK) and ERK2 (p42 MAPK), respectively. After activation, ERK1 and ERK2 are translocated from the cytoplasm to the nucleus, where they directly phosphorylate and activate transcription factors to induce the transcription of a series of cytoplasmic and nuclear proteins [1].
Raf/NF-κB Pathway
Raf-1 can stimulate the NF-κB pathway in two ways: (1) a direct and rapid pathway via the phosphorylation and activation of MEKK1 and I kappa B kinase p (IKKp), which stimulates the degradation of IκB via phosphorylation; and (2) in some cell lines, the activation may be achieved via an autocrine loop [18].
Relationship Between the MAPK Signaling Pathway and CVS after SAH
MAPK may be involved in the occurrence of CVS via the following mechanisms:
1.
Proliferation of vascular wall cells. Borel et al. [5] demonstrated that, after SAH, cerebral vascular walls exposed to the blood clot showed substantially increased cell proliferation and wall thickening, suggesting that these processes might participate in CVS.
2.
Sustained contraction of vascular smooth muscle. After SAH, increased intracellular Ca2+ concentrations in cerebral vascular cells activate calmodulin-dependent myosin light-chain kinase (MLCK), which leads to smooth muscle contraction [11].
3.
Immune and inflammatory responses.
4.
Apoptosis of vascular endothelial cells. Elevated expression of JNK and p38 MAPK is associated with endothelial cell apoptosis in brain vessels [3]. However, the mechanism by which MAPK modulates vascular endothelial cell apoptosis requires additional clarification in future studies.
PKC-Mediated Pathways
Structure and Biological Properties of PKC
PKC is a calcium/phospholipid-dependent protein kinase that regulates cell metabolism, growth, proliferation, and differentiation by catalyzing the Ser/Thr phosphorylation of many different proteins [25]. All PKC subtypes are composed of a single peptide chain, which is divided into four conserved domains, C1–C4 (in nPKC and aPKC the C2 domain is missing), and five variable regions, V1–V5 [14]. Based on their structures and activators, PKC isoforms are divided into three related families: groups A, B, and C.
PKC Activation
The pathways of PKC activation after SAH include the following:
1.
Increased endothelin (ET) expression. Studies have suggested that after SAH, high concentrations of OxyHb can directly stimulate ET expression in vascular endothelial cells, which catalyzes phosphatidylinositol bisphosphate (PIP2) decomposition to generate diacylglycerol (DAG). DAG then activates PKC [7].
2.
Decreased nitric oxide (NO). NO enters adjacent SMCs after its release from endothelial cells, where it activates soluble guanylate cyclase (GC) to produce cyclic guanosine monophosphate (cGMP). cGMP activates the intracellular calcium pump driving free calcium into the cell, relaxing smooth muscles.
3.
Formation of the “complement membrane attack complex.”
Relation Between PKC and CVS
Increasing evidence supports essential roles for PKC in delayed CVS. After SAH, sustained DAG increase activates PKC, which maintains sustained vascular SMC [7]. PKC inhibits the activation of potassium channels in vascular SMCs. This depolarizes vascular SMCs, leading to contraction. Adhesion of extracellular collagen matrix in the cerebral arteries after SAH affects the vessel diameter. PKC can activate protein tyrosine kinase (PTK), which activates Ras. PKC may also directly activate Raf-1 and thus activate MAPK, which results in sustained vascular SMC contraction.
Rho/Rho Signaling Pathway
Structure and Biological Properties of Rho Molecules
Rho proteins are small GTPases. Based on their primary structure, Rho proteins can be divided into at least five families: the Ras, Rho, Rab, Sarl/Arf, and Ran families. RhoA regulates the assembly of actin stress fibers, whereas Rac and Cdc42 regulate actin polymerization to form specific peripheral structures. Rho proteins also participate in the regulation of many basic cellular functions, including contraction, movement, adhesion, the maintenance of cell shape and polarity, gene transcription, and apoptosis [9].
Regulation of Rho Protein Activity
During cell metabolism, Rho proteins act as molecular switches and exist in the GTP-bound state (active state) or GDP-bound state (inactive state). Three major proteins regulate the activation of Rho: (1) GTP exchange factor, which promotes the phosphorylation of GDP and its exchange with GTP; (2) GTPase-activating protein, which activates the endogenous GTPase activity of Rho protein, hydrolyzing GTP into GDP; and (3) GDP dissociation inhibitor, which inhibits the conversion between GDP and GTP [27].
Relationship Between Rho-Related Signaling Pathways and CVS
After SAH, spasm-inducing substances in the blood can activate cell surface receptors and the downstream Rho/Rho kinase signaling pathway, resulting in increased MLC phosphorylation and SMC contraction. OxyHb and ET-1 are the two spasm-inducing substances that are well understood. In SAH, OxyHb released from self-dissolved red blood cells can cause persistent contraction of cerebral arteries in vitro. Studies suggest that activation of the OxyHb-dependent Rho/Rho kinase pathway is one step in the signaling cascade that leads to CVS [28]. ET-1 is another main cause of CVS after SAH. In the blood-containing CSF of SAH patients, ET-1 levels are elevated compared with control patients. In addition, cisternal injection of ET-1 in vivo produced CVS similar to that observed in humans. Lan et al. [15] examined rabbit basilar arteries with endothelial cells removed and found that ET-1 could significantly increase the amplitude of OxyHb-induced vasoconstriction. Importantly, the Rho kinase inhibitors Y-27632 and fasudil could reduce this effect.
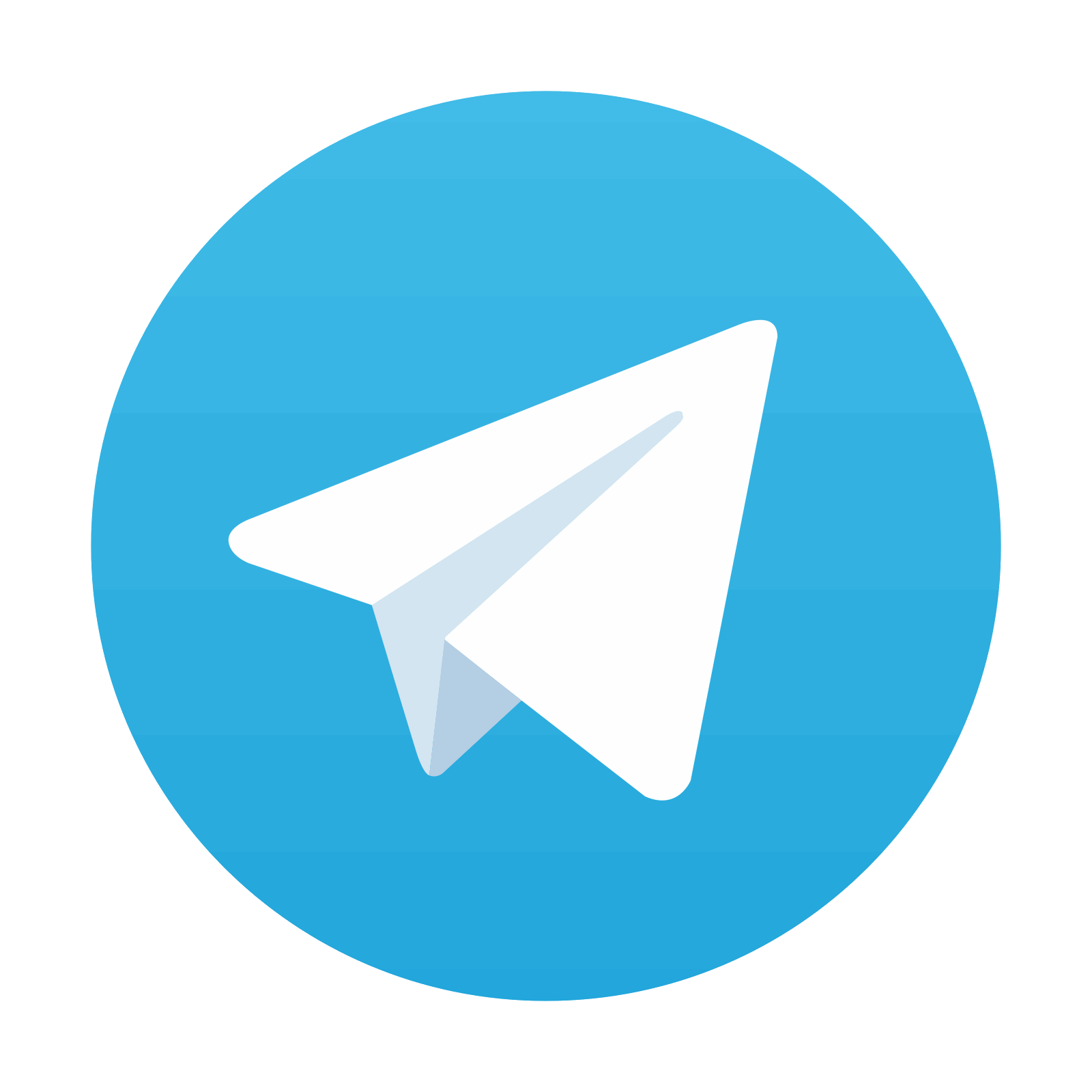
Stay updated, free articles. Join our Telegram channel

Full access? Get Clinical Tree
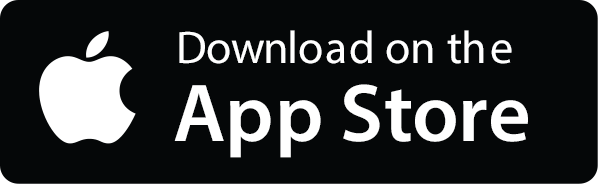
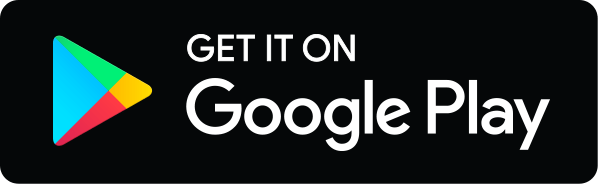