An African American male infant born at 23 6/7 weeks gestation via vaginal delivery with APGAR scores of 7 and 7 at 1 and 5 minutes, respectively, was intubated shortly after delivery. An echocardiogram showed a small patent ductus arteriosis and a small patent foramen ovale with left to right shunting. The patient’s initial hospital course was complicated by necrotizing enterocolitis for which he was treated with a Penrose drain and a course of intravenous (IV) piperacillin/tazobactam. On his second day of life, an ultrasound of the brain showed grade III bilateral intraventricular hemorrhage (IVH) without ventriculomegaly. Over the course of the next few days, his head circumference increased each day, and his fontanelle became full with splayed sutures. A follow-up ultrasound of the brain demonstrated significant ventriculomegaly that had not been present on the initial ultrasound (Figure 28-1).
IVH is a challenging problem for physicians caring for premature infants. Approximately 45% of neonates born before 30 weeks gestation with a low birth weight (≤ 1500 g) have IVH.1 Several studies have shown that the incidence of IVH decreases with increasing gestational age and birth weight.2
In full-term infants, causes of IVH include spontaneous hemorrhage from the choroid plexus, tumors, or arteriovenous malformations (AVMs). In premature infants such as the patient described above, the predominant cause of IVH is hemorrhage from the immature germinal matrix.3 The germinal matrix is located in the subependymal region found at the external angle of the lateral ventricles. The germinal matrix reaches its maximal size at 23 weeks gestation, halves in sizes by 32 weeks, almost completely involutes by 36 weeks, and disappears by the 39th week. Risk of hemorrhage corresponds with birth, while the germinal matrix is still present, with the highest risk in infants born at 23 weeks gestation and the lowest risk after 35 weeks. The timing of IVH has a bimodal distribution in which approximately 50% of IVH occurs within 12 hours of birth and 95% by day 4 of life.4,5 Both obstetrical and neonatal causes of ICH in premature infants have been proposed, but IVH in these patients is most likely multifactorial. Possible obstetrical factors include vaginal delivery, amnionitis, and maternal preeclampsia. Potential neonatal factors include respiratory distress syndrome, hypoxia, hypercarbia, and fluctuations in blood pressure.
Efforts to prevent IVH when a low-birth-weight premature infant is born have previously centered around medications such as phenobarbital, vitamin K, antenatal steroids, vitamin E, and indomethacin, each of which have all been studied with some efficacy.7 Currently, however, the focus has shifted to prenatal and postnatal measures to prevent IVH and to treat IVH effectively when it occurs. Prenatal measures include preventing premature birth when possible, prenatal administration of glucocorticoids, and optimal labor and delivery. Postnatal measures include adequate resuscitation, maintaining stable cerebral blood flow velocity, correction of other hemodynamic disturbances, and correction of any abnormal coagulopathy.8
The following are the grades of IV: grade I, germinal matrix hemorrhage only; grade II, grade I + IVH in < 50% of ventricles; grade III, grade II + ventricular distension with > 50% involvement of ventricles; and grade IV, grade III + intracerebral hemorrhage11. The risk of posthemorrhagic hydrocephalus after the initial IVH for each grade is 5% for grade I, 20% for grade II, 55% for grade III, and 80% for grade IV.11
Given the high probability of IVH in severely premature infants, we recommend a screening brain ultrasound for all infants born prior to 34 weeks gestation. Regardless of the findings of this screening ultrasound, subsequent ultrasounds should be performed if the patient’s fontanelle becomes fuller or if the head circumference crosses percentile lines. If the initial screening study demonstrates grade III or IV IVH, we recommend weekly ultrasounds regardless of clinical signs because of the high probability of developing hydrocephalus. Ultrasonography of the brain has a high sensitivity and specificity for IVH in the lateral ventricles, 94% to 100%, respectively,6 and can easily identify ventriculomegaly.
At 25 6/7 days of life (14 days of postnatal life), our patient’s fontanelle was bulging and his head circumference was 24 cm, markedly enlarged from birth records. A repeat ultrasound demonstrated progressive ventriculomegaly. The patient’s weight at this time was 990 g.
What should be done at this point? What are the options for treating hydrocephalus associated with IVH in low-weight premature infants?
Patients weighing < 1500 g do not tolerate shunting procedures because of malabsorption of diverted cerebrospinal fluid (CSF) and/or skin problems from shunt passage. Thus, for inpatients < 1500 g who need CSF diversion, temporizing measures can be considered to control hydrocephalus until the patient has gained enough weight for permanent CSF diversion. In a minority of patients (23%), the hydrocephalus will resolve after temporizing measures alone, and permanent CSF diversion will not be required.
Medicines such as acetazolamide and furosemide have proven ineffective in this population and can cause electrolyte imbalances.12 Serial lumbar punctures are painful to the patient, impractical, and potentially technically difficult in some cases. However, lumbar punctures are a reasonable temporizing measure if neurosurgical care is delayed because of systemic illness or other reasons. Serial ventricular taps are an option but can cause porencephaly as each tap passes a needle through brain parenchyma in a slightly different trajectory. The two most common temporizing options for low-weight premature infants (< 1500 g) are ventricular access devices (reservoirs) and ventriculosubgaleal shunts. These two procedures are approximately equivalent in efficacy in avoiding a ventriculoperitoneal (VP) shunt and have similar complication rate.13
Our patient had a reservoir placed and underwent daily reservoir taps over the next month. These taps stopped the progression of macrocephaly and the fontanelle became and remained relatively soft. Serial ultrasounds were stable for ventricular size, and the patient now weighed 2.5 kg. If not for the hydrocephalus, the patient was otherwise ready to be discharged.
The majority of patients (approximately 77%) who undergo temporizing measures such as reservoir placement or ventriculosubgaleal shunt will require permanent CSF diversion.13 Typically, a “tap holiday” is performed prior to deciding whether permanent CSF diversion is required. During the tap holiday, reservoir taps are not performed, and the fontanelle, head circumference, and ventricular size on ultrasound are observed. Patients must be observed carefully during tap holidays to ensure that they do not develop signs and symptoms of elevated intracranial pressure (ICP) such as sun-setting eyes, bradycardia, or at times, apnea. In most patients, the fontanelle will become progressively fuller, and it will be clear that permanent CSF diversion is required.
At many centers, VP shunting is the most common treatment offered once the decision has been made that permanent CSF diversion is necessary. Most pediatric neurosurgeons will offer a shunt when the patient’s weight is at least 1.5 kg and the patient is infection free. Others wait until the patient weighs at least 2.0 kg. Some pediatric neurosurgeons wait until the CSF protein is < 1.5 g/L,9 but there is no definitive proof that shunting needs to be deferred based on high CSF protein levels.
VPs have a high complication rate in premature infants. Shunt infection rates are much higher in premature than full-term infants, and shunt malfunctions may require multiple surgeries throughout childhood. Moreover, shunting to the abdomen may be challenging or not feasible in patients in this population (such as the one presented here) who have necrotizing enterocolitis. For these reasons, ETV/CPC (endoscopic third ventriculostomy with choroid plexus cauterization) has been offered recently at some centers as an alternative to shunting.10 In one study, nearly 40% of patients avoided a VP shunt with this procedure.14 Although this is not a high rate of success, the overall complication rate for the procedure is much lower than for ventriculoperitoneal shunting. At our center, we obtain a magnetic resonance (MR) image of the brain at the time point in which permanent CSF diversion is being considered. We assess the anatomic feasibility of endoscopic third ventriculostomy by reviewing the space anterior to the brainstem, the anatomy of the third ventricular floor, and the size of the foramen of Monro on each side. If the patient is a candidate for endoscopic surgery anatomically, we offer this to the parents along with a full discussion of the high failure rate in this patient population as well as the risks and benefits associated with shunts.
In our patient, an endoscopic third ventriculostomy with choroid plexus cauterization and septostomy was performed at approximately 3 months of age (adjusted). Postoperatively, his fontanelle was initially soft and his head circumference stabilized. Over the next 2 weeks, however, head circumference enlarged with radiographic evidence of increasing ventriculomegaly. A right parietal VP shunt was placed without complication, and the patient has done well since.
Figure 28-2 shows our overall treatment algorithm for management of premature infants with IVH.
A 14-year-old boy presents to pediatric neurosurgery clinic with a 1-month history of headaches and occasional word-finding difficulties. A magnetic resonance imaging (MRI) scan had been performed that demonstrated an enhancing lesion located in the left temporal lobe adjacent to Wernicke’s speech area, and there was significant adjacent edema (Figure 28-3).
After a long discussion about risks and benefits of surgery, the patient and his family decided to proceed with surgery for resection of this mass. A awake craniotomy for tumor resection with intraoperative language mapping was planned given the eloquent location. At surgery, a fibrous mass was noted that was clearly distinguishable from normal adjacent brain. Unfortunately, the patient did not tolerate being awake during surgery. The decision was made to proceed with tumor resection after frozen section revealed the diagnosis of meningioma, and a gross total resection was performed. Postoperatively, the patient was neurologically intact with normal receptive and expressive language function. He was observed in the pediatric intensive care unit and kept on dexamethasone (4 mg q6h) and levetiracetam (750 mg q12h).
The next morning, the patient remained neurologically nonfocal with the exception of intermittent difficulty with language repetition. Throughout the morning, his language function worsened considerably. By mid-morning, he had a near global aphasia. He remained awake and alert and otherwise neurologically intact.
The timing of the patient’s aphasia is very important in determining the likely cause. If a patient awakens immediately after surgery with a new neurologic deficit, the most likely etiologies are direct injury to the eloquent brain or ischemic damage to the brain from sacrificing an artery that supplies the relevant area of brain.19 In such cases where a new deficit occurs in a delayed fashion, postoperative hemorrhage must be ruled out immediately with an imaging study. The most likely etiology, however, is brain edema. Patients with meningiomas are particularly prone to postoperative edema and can, at times, have a difficult postoperative course.20 A stat MRI scan was ordered (Figure 28-4).
A complete resection of the tumor was noted on the postoperative MRI scan without significant hemorrhage. Diffusion-weighted imaging demonstrated no ischemic event, and the FLAIR (fluid attenuation inversion recovery) MRI sequence showed edema similar to the preoperative study or perhaps slightly worse.
Based on the MRI scan and the timing of the patient’s aphasia, the presumptive etiology of his aphasia was brain edema in the adjacent eloquent language cortex of the superior temporal gyrus. Because the patient was only at 24 hours after surgery, we were concerned that his edema may worsen because peak edema after surgery is often 48 to 72 hours postoperatively.19 The patient was kept in the intensive care unit and observed carefully. His dexamethasone dose was increased (to 10 mg q6h), and we observed his sodium level carefully. His sodium level was currently 136 mEq/L, so hypertonic saline (3% normal saline) was started with a goal of keeping his sodium > 140 meq/L. Speech therapy was initiated.
On postoperative day 3, the patient continued to have profound aphasia. He followed commands, but he articulated few words, and these words were incomprehensible. In addition, he had two witnessed seizures that consisted of jaw deviation and lip smacking lasting approximately 1 minute each. Pediatric neurology was consulted and recommended increasing the levetiracetam dose to 1000 mg BID and ordering an electroencephalogram (EEG), which demonstrated intermittent seizure activity. In spite of the increased levetiracetam dose, the patient began having increased intermittent seizure activity over the next 24 hours. On postoperative day 4, his levetiracetam dose was increased to 1500 mg BID, and he was additionally loaded with fosphenytoin. His sodium level was kept > 140 meq/L with hypertonic saline. On postoperative day 5, his seizure activity worsened, and he was found to be in status epilepticus.
Status epilepticus is defined as a seizure lasting ≥ 5 minutes clinically or electrographically or recurrent seizures without recovery to baseline.15 The speed of diagnosis and treatment of status epilepticus is of paramount importance. Patients should be kept in an intensive care unit under continuous observation as interventions are initiated to stop the seizure activity as soon as possible (Figure 28-5). First, the basics of airway, breathing, and circulation (ABCs) should be assessed and managed. If a patient is not protecting his/her airway, early intubation should be performed. While simultaneously administering medications to stop the seizures, stat laboratory studies should be performed to rule out an electrolyte abnormality, which could be contributing to seizure activity. Laboratory studies should include blood glucose concentration, complete blood count, basic metabolic panel, and calcium, magnesium, and anticonvulsant levels. Depending on the clinical history, a toxicology screen or liver function tests should be additionally ordered. The patient should be on continuous EEG monitoring, and a stat portable head computed tomography (CT) scan should be ordered.16
Medications should be administered in a stepwise fashion with the primary goal of stopping clinical and electrographic seizures as soon as possible. Patients should be given an initial fast-acting anticonvulsant therapy such as IV lorazepam or diazepam PR. Simultaneously, if not already on an anticonvulsant, the patient should be loaded with fosphenytoin or levetiracetam. If seizure activity continues, a third-line medication such as phenobarbital, pentobarbital, or continuous infusion of midazolam or propofol is given until burst suppression is achieved on EEG. Patients are intubated electively if third-line medications are required or if seizure activity continues beyond 30 minutes.17 In children < 2 years of age with status epilepticus, pyridoxine-dependent seizures (an autosomal recessive condition) should be included in the differential diagnosis and treated with IV pyridoxine, 100 mg.18
In the current patient, when status epilepticus began, he was given several doses of IV lorazepam. Because he was already on several anticonvulsants, the decision was made to intubate the patient. A third anticonvulsant, phenobarbital, was added, and IV midazolam was infused. At this point, his seizure activity stopped. He was kept intubated until he was seizure free for 2 days. He was then extubated but was continued on all 3 anticonvulsants. Phenobarbital was quickly weaned, and fosphenytoin was switched to phenytoin, 200 mg BID. Over the course of the next few days, his hypertonic saline was weaned. His dexamethasone was weaned off slowly (over approximately 2 weeks). He never had any further seizure activity, and his language function returned completely to normal over approximately 2 weeks. His additional anticonvulsants were weaned off, and he was sent home on only levetiracetam. Follow-up appointments were arranged with both pediatric neurosurgery and pediatric neurology.
A 5-year-old boy with no significant past medical history was transferred from an outside hospital. He initially presented with low-grade fever (100.6°F) and cough and was sent home with the presumptive diagnosis of a viral upper respiratory infection and tonsillitis. He returned to the emergency department the next day with new symptoms of urinary incontinence and altered gait. The patient denied headache or any other symptoms other than difficulty keeping his eyes open. On examination, he was neurologically intact with the exception of unsteady gait and bilateral ptosis (without cranial nerve III palsy). A CT scan of the brain was performed that demonstrated profound ventriculomegaly caused by a homogenous hypodense mass in the region of the posterior midbrain with obstruction of the cerebral aqueduct (Figure 28-6).
It is striking that, despite the patient’s profound hydrocephalus, he was wide awake and did not complain of headaches. This implies that the patient’s ventriculomegaly was long standing and that the tumor was likely benign, as children with slowly progressive hydrocephalus from benign tumors may be relatively asymptomatic because of their ability to accommodate to slowly increasing ICPs.21 Because of the potential for precipitous decline, admission to the pediatric intensive care unit with hourly neurologic checks is warranted in even asymptomatic patients with significant hydrocephalus. Initiation of IV dexamethasone may be considered. There should be a low threshold for placing a ventriculostomy urgently if the child begins vomiting, has severe headaches, or becomes lethargic. In this case, if the child is stable, an MRI scan with and without gadolinium should be obtained to gather more anatomic detail about the tumor before determining the appropriate treatment plan (Figure 28-7).
Figure 28-7.
MRI of the brain of the 5-year-old boy (shown in Figure 28-6) to obtain anatomic detail to determine an appropriate treatment plan.




The MRI demonstrated a mass lesion emanating from the dorsal midbrain and creating a cleft in the midbrain structures as best demonstrated on the FLAIR sequence. Hydrocephalus with transependymal reabsorption of CSF was demonstrated. We hypothesized that displacement of midbrain tissue was the likely etiology of the patient’s bilateral ptosis, as the levator palpebrae superioris is innervated by the oculomotor nuclear complex, which resides in the midbrain.22 The tumor did not enhance with gadolinium and was hypointense on diffusion-weighted imaging, suggesting that it was likely benign. The anterior aspect of the tumor abutted the posterior third ventricle.
Clearly the patient needs a pathological diagnosis and likely resection of the tumor. The patient also has obstructive hydrocephalus that needs to be treated. One option is to proceed to craniotomy for tumor resection with the hope that removing the mass will alleviate the hydrocephalus. A second option, which the authors selected, is to treat the hydrocephalus with an endoscopic third ventriculostomy and leave an external ventricular drain (EVD) both to monitor and to treat elevated ICP. The EVD can additionally be used to drain CSF to relax the brain during a subsequently staged craniotomy for tumor resection. During the endoscopic procedure, we visualized the brain mass in the posterior third ventricle but did not attempt a biopsy because the mass was covered by a normal appearing ependymal surface. Violating a normal ependymal surface to biopsy a brain tumor close to the ventricle is associated with a higher rate of neurologic deficits than if the tumor is directly visualized within the ventricle.23,24
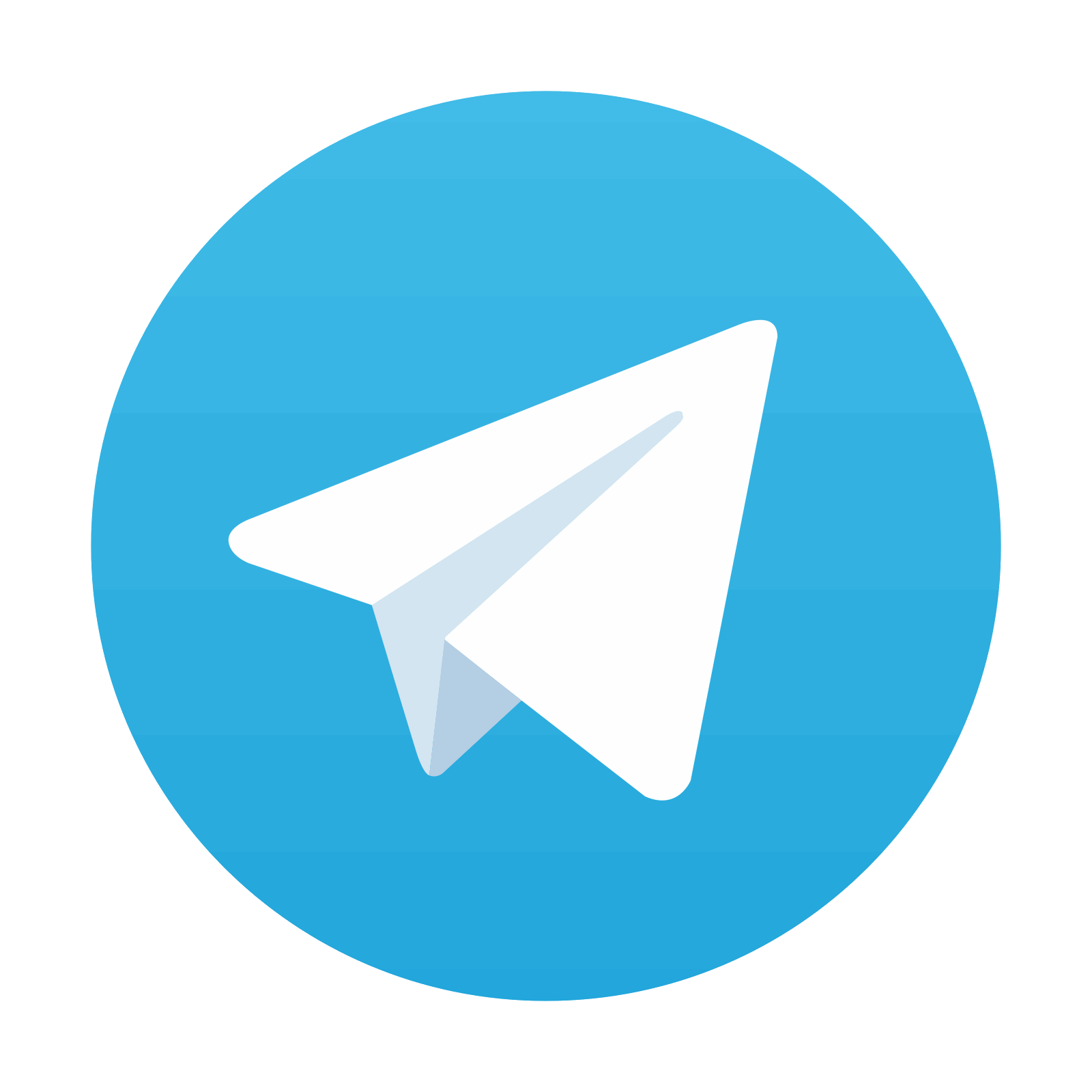
Stay updated, free articles. Join our Telegram channel

Full access? Get Clinical Tree
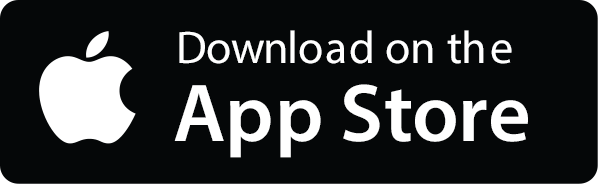
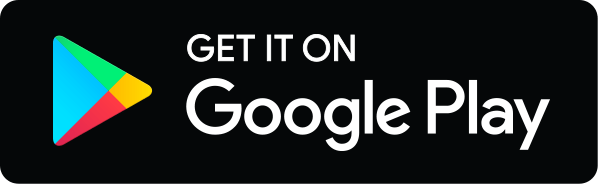