Congenital heart disease
Vasculopathies
Moyamoya disease/syndrome
Traumatic arterial dissection
Connective tissue disorders
Radiation-induced vasculopathy
Hypercoagulable states
Antithrombin deficiency
Protein C deficiency
Protein S deficiency
Activated protein C resistance with or without factor V Leiden mutation
Prothrombin gene mutation G20210A
MTHFR mutations
Anticardiolipin antibodies and lupus anticoagulant
Sickle cell disease
Infection
Meningitis
Vasculitis
Intracranial tumors and hematologic malignancies
Genetic and metabolic disorders
Mitochondrial disease
Trisomy 21
Neurofibromatosis type 1
Cerebrovascular disease in children can result in significant neurologic morbidity. Nearly 20 % of children with AIS after the perinatal period can have a recurrent stroke within 5 years [8]. In a prospectively collected data set, > 70 % of survivors of AIS had neurologic deficits at the time of discharge, and more than half of the patients were treated with antiseizure medications during the acute hospitalization, emphasizing the important contribution of seizures in childhood stroke [9]. Data for outcomes at 1 year or longer also demonstrate that residual disabilities including both cognitive impairment and sensorimotor deficits persist in a majority of pediatric stroke patients [10–18]. A recent analysis of pediatric stroke survivors at very long follow-up also showed that a majority still exhibited neurologic deficits in adulthood, and while most were independent in their activities an important fraction of at least 20 % had moderate-to-severe deficits resulting in dependence on others in some aspect of their daily life [19].
Seizures Associated with Pediatric Stroke
Seizures commonly occur in the context of pediatric stroke . Cerebrovascular disease is more commonly associated with seizures in infants and children than in adults. In fact, seizures are often the presenting symptom of stroke in this population, but they can occur later after stroke as well. The current knowledge of seizures in the acute setting of stroke in infants and children is discussed here.
Incidence of Seizures in Perinatal Stroke
One of the earliest reports of seizures associated with perinatal stroke identified cerebral infarction on autopsy in 29 preterm or term infants and found that convulsions were described in the records of five of these patients [20]. In the early 1980s, widespread use of diagnostic neuroimaging allowed for the antemortem diagnosis of perinatal stroke , and a number of case reports and small series quickly revealed a strong association between early-life seizures and perinatal cerebral infarction [21–23]. Clancy and colleagues drew attention to the fact that seizures may in fact be a heralding sign for ischemic stroke, as 8 of 11 neonates in their series exhibited seizures as the only localizing sign [24].
In a more recently published series of seven neonates with stroke, all presented with focal clonic seizures within the first 3 days of life with a mean presentation of 26 h [25]. Sreenan et al. described 46 term neonates with stroke, of whom 91 % exhibited clinical behaviors concerning for seizures [26]. These manifested as primarily apnea in 36 % and focal clonic movements in 38 %. Less frequent presentations were seizures described as generalized tonic–clonic and focal tonic seizures. The diagnosis of seizure in this population relied solely on clinical observations (i.e., the lack of continuous electroencephalography (EEG) recording means that many seizures may have been missed, and thus the burden of seizures likely was underestimated). Only 11 patients demonstrated epileptiform activity on EEG, and 16 demonstrated burst suppression. Low-voltage background was the most evident abnormality in 12 patients, and 7 had normal EEG recordings emphasizing the limitations of intermittent EEG recordings in these patients.
The data discussed above clearly indicate that seizures during the neonatal period should raise suspicion for stroke, but a subset of patients with perinatal stroke do not present with early seizures. In such cases, hemiparesis often does not become evident until several months of age as motor systems mature, and it has been estimated that up to 30 % of perinatal strokes may present in this delayed fashion and are then referred to as “presumed perinatal stroke” [27]. One study of an Estonian population of children formally assessed differences in the presentation of these two distinct groups [28]. They found that while seizures were commonly a presenting sign in children diagnosed with perinatal stroke, children who were retrospectively diagnosed later in life came to medical attention for hemiparesis far more often than for seizures. One possibility for this difference in presentation may be that there is a specific and relatively narrow time window in which the neonatal brain is at risk for seizures due to acute focal infarction, a point that warrants further investigation as it may prove important in guiding treatment of these infants.
Early seizures in the acute setting of perinatal stroke are a well-defined occurrence, but late seizures and chronic epilepsy after perinatal stroke are less well understood. Variable rates of late seizures or chronic epilepsy have been reported, but many of these are provided by small case series and are difficult to reconcile because of small populations and variable periods of follow-up. An Australian registry with more than a decade of follow-up data for some patients found a cumulative incidence of epilepsy of 55 % by 10 years of age, although only 15 % had active epilepsy (defined as a seizure within the past year) after 10 years of follow-up [29]. Another study retrospectively reviewed the charts of 61 infants with perinatal stroke and at least 6 months of follow-up [30]. In this population, 67 % were classified as having epilepsy at some point after discharge, but a majority of these patients were seizure free at the time of last follow-up raising questions about how chronic their epilepsy truly was. In their population, 9 % of patients did not have any neonatal seizures but did develop later seizures. Specifically looking at patients with a delayed presentation of perinatal stroke, 38 % still were diagnosed with epilepsy at some point during a median follow-up of 49 months [31]. This study contrasts to some extent with a more recent prospective study of perinatal stroke that estimated a seizure-freedom rate of 73 % at 3 years [32].
Incidence of Seizures in Childhood Stroke
As in perinatal stroke, seizures are commonly associated with stroke during childhood. One population-based study found that children were 18 times more likely than adults to have a seizure within the first 24 h after stroke [33]. The larger studies of childhood stroke in the USA identified seizures in up to 50 % of patients, most of which occurred within a day after presentation [34, 35]. In a Taiwanese cohort of childhood ischemic stroke, about 41 % exhibited at least one seizure, and of these three quarters developed their seizures within 7 days after stroke [36].
Two groups have analyzed different prospectively collected US childhood stroke cohorts specifically for seizures as the presenting sign of stroke, each finding that more than 20 % of patients actually presented with seizures [37, 38]. A Chinese study found that 52 % of their childhood stroke patients presented with seizures, but this study was retrospective utilizing discharge diagnostic coding which may not be as accurate as prospectively collected samples [39]. Further analysis in one of these studies found that all patients presenting with seizures ultimately developed a hemiparesis, but seizures were the heralding sign and preceded any other symptoms [37].
Compared to the acute setting of stroke, there are few studies describing long-term epilepsy outcomes in children with stroke. Yang et al. found that 21 of their 73 children with stroke experienced recurrent seizures, but noted that 12 of these patients had only a few seizures. Of the remaining nine patients with chronic epilepsy, five were well controlled on antiseizure medication, while four patients suffered from treatment-refractory epilepsy [35]. A more recent study similarly found about 29 % of childhood stroke patients with poststroke epilepsy [40]. While this study included both perinatal stroke and childhood-onset stroke, there was no difference in the prevalence of epilepsy between the two different groups .
Risk Factors for Seizures in Pediatric Stroke
One could reasonably surmise that particular patient characteristics or stroke features may confer an increased risk for seizures, and this has to some extent been borne out in the pediatric literature. Although seizures are more common in pediatric stroke than adult stroke, the relationship of age within the pediatric population is less clear. Studies have found variable conclusions related to age, with one of the more significant studies identifying an age less than 3 years as significantly associated with seizure [37]. Some studies have variably seen increased incidence of seizures in patients less than 1 year of age [41] or greater than 1 year of age [42], and yet others have failed to identify any significant difference between age groups.
Most studies of pediatric stroke have not identified any difference in the occurrence of seizures in different subtypes of stroke (i.e., ischemic vs. hemorrhagic). Cortical involvement, however, is significantly associated with the occurrence of at least one seizure regardless of timing of onset (early or late) and also with recurrent seizures and epilepsy [35, 40]. Location, in terms of either supratentorial versus infratentorial or anterior versus posterior, was not associated with seizures being the presenting sign of stroke [37].
Use of these epidemiologic studies to define risk factors for seizures has significant limitations with the primary one being a lack of statistical power to identify differences. The conclusions of such studies must be analyzed with this in mind. Identifying risk factors for seizures should certainly be a priority, as it may impact the way we treat children with cerebrovascular disease [6]. For example, identifying specific characteristics of stroke patients who present with seizures may help us determine which patients presenting with seizures should be evaluated for stroke. Similarly, identifying patient or stroke characteristics that are predictive of recurrent seizures and epilepsy may help to better select patients that will require maintenance antiseizure medications. Achievement of these goals, however, will require assessment of larger multicenter populations using consistent criteria for the identification of such risk factors.
Seizure Semiology in Pediatric Stroke
Findings in children post stroke illustrate that stroke should be considered in any child presenting with new-onset seizures in conjunction with focal neurological deficits [37]. The type of seizure after stroke may depend on whether seizures occur early versus late (defined previously). Gupta et al. reported that early poststroke seizures were more likely to be focal (57 %) with generalized seizures (65 %) more commonly seen in the late poststroke seizure group [43]. Contrarily, Horner and colleagues reported that early poststroke seizures were more likely to be generalized [44]. Others have found no significant difference in seizure type based on early versus late [36].
Generally, the semiology of seizures with lateralizing signs has been shown to be concordant with the infarcted hemisphere [31, 37]. In an evaluation of epilepsy in hemiplegic cerebral palsy due to perinatal AIS, the authors found that these children may have seizure characteristics similar to children with non-symptomatic epilepsies (e.g., benign myoclonic epilepsy of infancy, benign childhood epilepsy with centrotemporal spikes, benign childhood occipital epilepsy) [29]. Given the similar electrophysiological and clinical features of such self-limited epilepsies of childhood, a more conservative approach towards management could be employed with regard to drug choice and length of therapy and less urgency for surgical consideration.
Pathophysiology of Seizures in Pediatric Stroke
In discussing the epidemiology of seizures in pediatric stroke, a point has been made to distinguish between seizures occurring early after stroke and those occurring later because of a presumed difference in the underlying pathophysiology. The International League Against Epilepsy (ILAE) defines acute symptomatic seizures after a cerebrovascular accident as that which occurs within 7 days of stroke [45]. But studies have variably defined them as occurring within either 1 or 2 weeks after stroke. Nevertheless, the pediatric literature shares the hypothesis of the adult literature that early and late seizures represent distinct pathophysiologic mechanisms [35, 46, 47]. Acute or early seizures are thought to be secondary to metabolic derangements related to the stroke, while late seizures are more likely to be the sequelae of structural changes that have resulted in established epileptogenic foci. In several studies, the timing of initial seizure onset was related to the ultimate development of recurrent seizures or epilepsy [35, 40]. Here, we discuss the unique nature of the immature brain with respect to commonly proposed mechanisms for both acute seizures and later epileptogenesis .
Various factors contribute to the common occurrence of seizures in perinatal and childhood stroke (Fig. 9.1). It is important to remember that the brain already has a reduced seizure threshold early in life. This is in part due to the developmental regulation of neurotransmitter systems. γ-Aminobutyric acid (GABA)ergic transmission, the primary inhibitory system in the adult brain, is poorly developed early in life and can even be excitatory in the first days after birth [48, 49]. Coupled with the fact that glutamatergic receptors exhibit distinct subunit compositions that promote facilitated and sustained activation [50], the balance between excitation and inhibition in the immature brain is shifted strongly in favor of excitation. Furthermore, the synaptic pruning occurs during postnatal brain development , and children’s brains may therefore have an overabundance of synapses [51]. This likely compounds the effects of the excitotoxic cascade that is initiated by ischemic injury, promoting both neuronal injury and seizures.


Fig. 9.1
The immature brain has an already low seizure threshold due to multiple factors of development. Pathological changes occurring after ischemic injury interact with these inherent characteristics to cause acute seizures and contribute to the process of epileptogenesis
Glutamate is increased in the extracellular milieu after acute ischemia, and excitotoxicity has been associated with secondary neuronal injury and a permanent epileptiform phenotype in an in vitro model of stroke-induced epilepsy [52–54]. In an ischemic rat model, high extracellular potassium leading to neuronal depolarization was postulated to mediate postischemic seizures [55].
Simply having a lower seizure threshold may help to explain the frequency with which acute seizures occur after childhood stroke, but epileptogenesis (i.e., the predisposition to having recurrent, unprovoked seizures) following stroke is much more complicated. This process has been intensely investigated over the years, yet we still have no effective treatments to prevent the establishment of epileptic foci following stroke or other types of brain injury. A number of processes have been postulated to underlie epileptogenesis, many of which exhibit important differences between the immature and mature brain as reviewed by Rakhade and Jensen [56]. The most important consideration is that pediatric stroke occurs during a critical time period when neuronal networks are being established, and disruption of this process can result in aberrant networks that promote epileptogenesis.
“Plasticity” is a common term utilized when discussing recovery from stroke or other brain injury. This concept is invoked at many different levels and can refer to molecular changes such as alterations of neurotransmitter and signaling systems, proliferation and differentiation of progenitor cells, or adaptation of surviving neural networks. It is widely accepted that the capacity for plasticity in the brain varies over the life span [57]. Immediate early genes expressed following stroke include Activity-regulated cytoskeleton-associated protein (Arc), brain-derived neurotrophic factor (BDNF), and others that have been implicated in synaptic reorganization and epileptogenesis [58–60]. Ischemic injury to the immature brain also can lead to increased neurogenesis and gliogenesis [61]. While these processes are normal components of brain development and may represent reparative strategies following injury, they also may contribute to the maladaptive consequence of aberrant structural changes that result in epileptogenesis .
Pediatric stroke is a unique entity that deserves its own study separate from adult stroke. The young or immature brain is markedly different from the aged brain in which adult stroke typically occurs. Children demonstrate different patterns of injury and, importantly, different patterns of recovery. The establishment of epileptic foci following injury is likely to be influenced by these factors; therefore, a better understanding of these processes may be helpful in understanding the differences in seizure occurrence following stroke in children and adults. Unfortunately, there is a paucity of data describing specific differences between stroke recovery in adults and children.
Electroclinical Manifestations in Pediatric Stroke
Role of Electroencephalography in Diagnosis
Neuroimaging is the most widely used modality in the early evaluation of stroke ; however, EEG is an ancillary tool in the early evaluation of poorly defined poststroke focal neurologic symptoms, and both clinical and basic science data suggest that it may help with predicting neurologic recovery [62, 63]. In combination with MRI, an EEG also may be useful in distinguishing between a focal, structural, or vascular disturbance and a postictal paralysis in a patient presenting with a hemiparesis (i.e., a normal MRI combined with an EEG showing focal epileptiform discharges suggests the latter). Similar to other aspects of childhood stroke, there is a paucity of strong evidence to guide the use of EEG in the evaluation of these patients.
Generalized slowing, focal epileptiform discharges, and electrographic seizures on EEG are more likely to be seen in children with stroke who had a clinical seizure. Focal cortical dysfunction on interictal EEG has been associated with late poststroke seizures in children and a higher rate of poststroke epilepsy; however, this finding is not consistently seen in all studies [38]. Much of the adult literature reveals focal or diffuse slowing of background activity in those patients with seizure at the onset of stroke with approximately 16–23 % having interictal epileptiform discharges [64, 65]. Importantly, infrequent recurrence of seizures is reported. Adults with EEG detecting status epilepticus (SE) were often found to have fragments of periodic lateralized epileptiform discharges (PLEDs) interrupted by seizure activity. This might suggest that antiseizure drugs are not necessary in stroke patients with single seizures; however, they may be appropriate in those with SE or PLEDs [65]. Detailed characterization of interictal EEG findings in pediatric stroke is lacking.
Seizure propagation in both the infarct core and the penumbra after stroke as well as epileptiform discharges such as PLEDs generated in the penumbra have been demonstrated in animal models [55]. PLEDs have been identified in adults with acute cortical lesions; however, subcortical lesions may also trigger this electrographic pattern [55, 66]. In a rat model, there was a significant association between the occurrence of PLEDs before nonconvulsive status (NCS) and prolonged NCS [55]. PLEDs detected in the early phase of stroke may be prognostic, as they typically are associated with a poor outcome and increased risk of seizures and status epilepticus [67]. Rat models have also demonstrated intermittent rhythmic delta activity (IRDA) in the setting of ischemic insult [55]. In the adult population, patients with frontal intermittent rhythmic delta activity (FIRDA) and diffuse slowing on the poststroke EEG had a higher risk of developing late poststroke seizures [68].
Continuous EEG monitoring is a tool often utilized in the adult population to analyze stroke evolution and predict clinical course. However, investigations on acute pediatric stroke and its associated electroclinical manifestations are scant. While EEG in the setting of acute pediatric AIS may have limited utility in predicting future epilepsy, for those patients with stroke who have altered mental status, it may be particularly helpful in evaluating for nonconvulsive status epilepticus (NCSE) [38]. NCSE in children has a known association with stroke [69]. Abend et al. found that 23 % of children with clinically evident seizures after ischemic stroke also had nonconvulsive seizures detected on EEG. Importantly, however, only four of their 13 patients underwent continuous EEG monitoring. Of those that did, 75 % demonstrated electrographic seizures without any clinical correlate [37]. Given that acute structural brain lesions such as infarction and nontraumatic hemorrhage are reported to be the most common etiologies of NCSE in children, continuous EEG monitoring may be of paramount importance in this population [69, 70]. Studies suggest that NCSE is typically detectable immediately or within 24 h of monitoring [38]. Unfortunately, we still lack data that definitively indicate that treating early seizures or NCSE results in better outcomes.
Role of EEG in Prognostication
Quantitative EEG (QEEG) as a marker for brain dysfunction post stroke in children has also not been studied. In a comprehensive review of the adult literature by Finnigan and Putten, it was determined that QEEG has “substantial value” in ischemic stroke. Reductions in delta power, delta/alpha power ratio (DAR), (delta + theta)/(alpha + beta) power ratio (DTABR), or brain symmetry index (BSI) during the acute ischemic stroke interval were generally associated with better functional outcomes. Notably, these QEEG changes were found to have higher predictive value than concurrent neurological scales and assessments, and they were detected even before changes in patients’ neurological symptoms or upon repeat imaging [71]. QEEG parameters such as the alpha-to-delta ratio (ADR) have been evaluated in a rat model of ischemic stroke and found to be closely correlated with functional recovery suggesting this type of monitoring may be useful in predicting outcome [63].
EEG monitoring is a relatively inexpensive bedside diagnostic study that can detect specific patterns and QEEG changes in electrical brain activity after cerebral injury such as stroke. Although it can help identify specific patterns such as PLEDs, it is still unclear what these pathologic EEG rhythms and changes might portend, especially in children who have different neuronal maturity. With further investigation into the specific electroclinical manifestations in children post stroke, EEG monitoring may substantially aid in therapeutic decisions.
Treatment of Seizures in Pediatric Stroke
Given limited data on poststroke seizures and epilepsy in children , current management is broadly extrapolated from the larger volume of adult literature that incompletely addresses many questions related to pediatric therapy. Physicians are faced with making difficult therapeutic decisions, such as whether to treat an isolated seizure and what antiseizure medications to use. Moreover, the potential neuroprotective role of antiseizure medications versus their potential negative impact on stroke recovery must be considered.
Acute Seizure Management
There is evidence to suggest that acute brain injury and seizures work synergistically to worsen outcome by contributing to metabolic stress and exacerbating injury in affected neurons [72]. Experimental studies in laboratory animals advance this concept demonstrating that recurrent seizure-like activity in the setting of cerebral ischemia considerably increases infarct size and can preclude functional recovery. Treatment with certain antiseizure drugs, also considered neuroprotective, tempered these effects [73, 74]. In studies of both humans and rats, increased cerebral metabolism following seizures has been associated with an enhanced mismatch between an already compromised energy supply and demand ultimately leading to mitochondrial damage and possible irreversible cognitive and neurological deficits [72].
Although there is such experimental evidence promoting treatment with antiseizure therapy, determining which patients would benefit from prophylactic treatment is difficult. Even in the adult literature, there is insufficient evidence to reliably predict those who will have development of poststroke epilepsy. Data from Abend et al. suggest that children without seizure on presentation with AIS may not require prophylactic antiseizure therapy [37]. Given that early poststroke seizures, although common, tend to not recur, therapy with antiseizure medication could possibly be avoided. Conversely, late poststroke seizures may be a risk factor for poststroke epilepsy; therefore, these patients may warrant additional prophylactic therapy [64]. These concepts are bolstered by data suggesting that treatment of early seizures in the setting of traumatic brain injury does not impact the development of late seizures or epilepsy in that setting [75].
Given the known association of NCSE in children with acute structural brain lesions such as infarction and nontraumatic hemorrhage, antiseizure drug treatment should be considered in any child with altered mental status after stroke. Although these patients have electrographic seizures without overt motor manifestations, the possibility of long-term neurologic consequences should be kept in mind. In an in vivo rat model of focal brain ischemia-induced NCSE, there was a higher mortality rate within the 24-h recovery period in the presence of NCSE, and this was effectively reduced by 73 % with effective blockade of epileptic activity [72].
Similarly, studies have demonstrated that PLEDs contribute to local increases in metabolism for several days after termination of SE; therefore, they have potentially harmful implications [76]. Stroke subtype (i.e., ischemic vs. hemorrhagic) should potentially be considered when making therapeutic decisions. Although not statistically significant, in an animal study, brain hemorrhage was found to have a 3.5-fold increase in the number of NCS events and a 2.3-fold higher mortality rate (consistent with clinical findings of increased seizures in patients with intracranial hemorrhage when compared to ischemic stroke) [72]. Interestingly, high doses (twice the reported effective dose 50, ED50) of ethosuximide and gabapentin effectively attenuated the NCS activity and provided neuroprotective effects, compared to commonly used drugs for status, fosphenytoin and valproate.
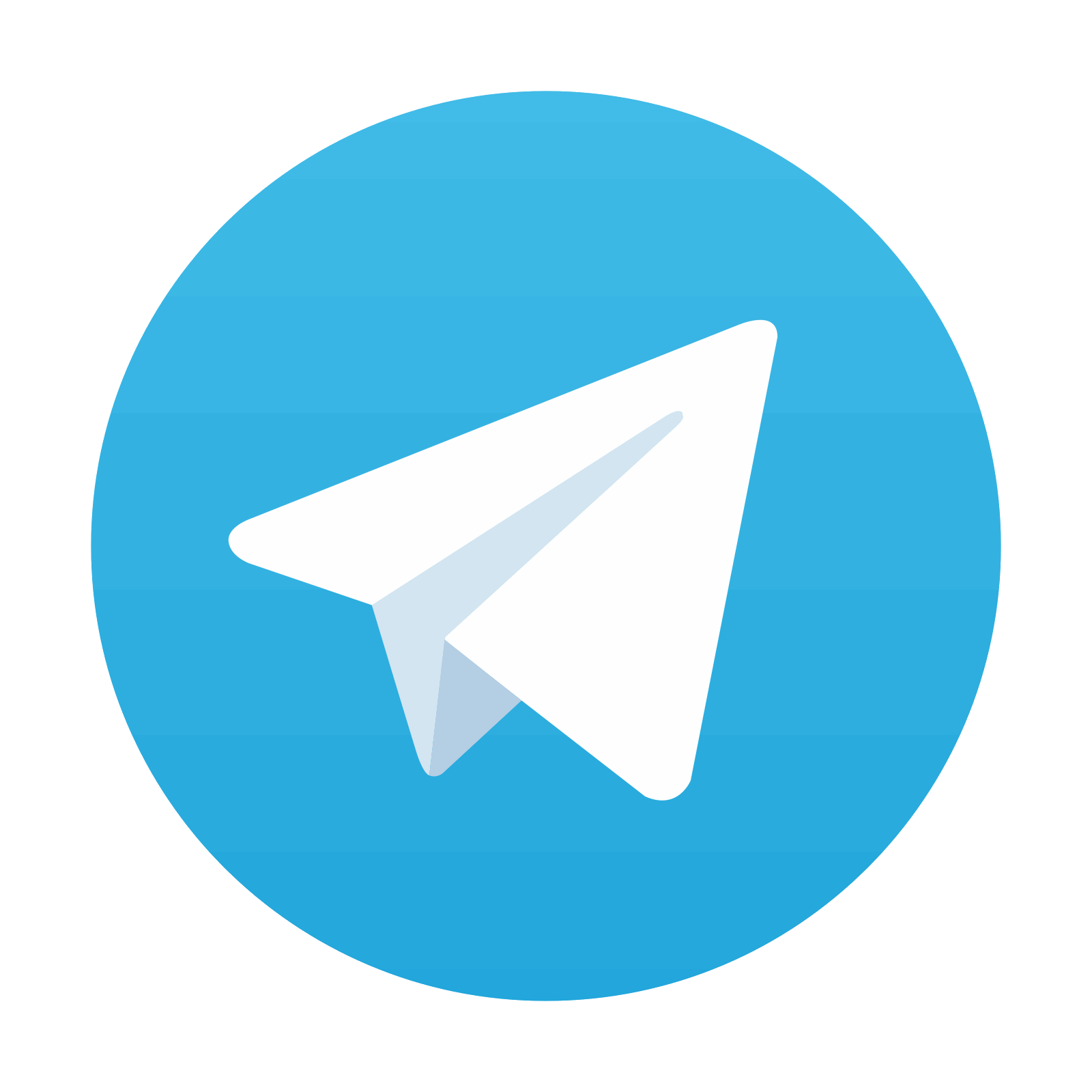
Stay updated, free articles. Join our Telegram channel

Full access? Get Clinical Tree
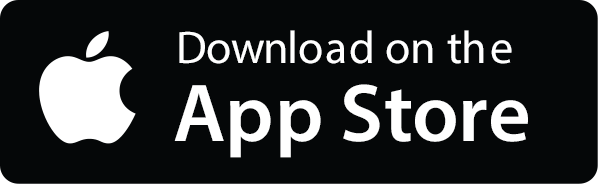
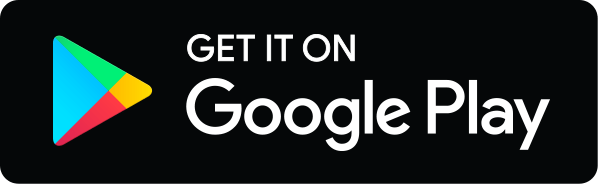