Fig. 8.1
(a, b) Pedicle subtraction osteotomy: Three-column transpedicular vertebral wedge osteotomy, leaving the anterior cortex intact, which acts as a hinge for the closure of wedge defect. The osteotomy places two large cancellous surfaces together to achieve fusion and restore balance, and the two nerve roots exit through one large neural foramen
Fixed sagittal deformity can be iatrogenic, with etiologies including multilevel laminectomy, fixation of thoracolumbar fractures, distal lumbar arthrodesis for degenerative lumbar disease, segmental fixation for correction of scoliosis, and previous arthrodesis with Harrington rods extending into the lumbar spine. It can be secondary to ankylosing spondylitis, multilevel disc degeneration, and kyphosis from multilevel compression fractures due to osteoporosis or trauma, or secondary to neoplastic, infectious, metabolic, and congenital disorders. Determination of whether the spinal deformity is fixed or flexible helps significantly with the surgical decision-making process. Assessment of curve flexibility and compensatory ability of adjacent segments of the spine helps in determining the surgical approach, number of spinal levels to be fused, and the need for corrective osteotomies. Deformities that demonstrate more than 30 % correction on bending radiographs are generally considered flexible and less likely to require osteotomies, whereas curves that demonstrate less than 30 % correction with bending are generally considered fixed or rigid deformities and are more likely to require osteotomies [12]. Many adult deformity patients have had previous fusion surgery, which may contribute to rigidity of the deformity and may increase the requirement for osteotomy in order to achieve sufficient alignment. Flexibility should be evaluated in all curves that are considered for surgical correction.
Fixed sagittal deformity makes erect posture difficult without flexion of the knees and/or hyperextension of the hips. The sagittal vertical axis (SVA), which is the horizontal offset between the C7 plumb line and the posterior superior end plate of the S1 vertebrae, is often significantly positive in patients with fixed sagittal deformity. There is no clear consensus regarding normal SVA, but recently Schwab et al. in the Scoliosis Research Society (SRS)–Schwab adult spine deformity classification considered SVA <4 cm as normal, SVA between 4 and 9.5 cm as moderately increased and SVA greater than 9.5 cm as severely increased [13]. The T1 spinopelvic inclination (SPI) and T-9 SPI are used as alternative measures of global sagittal alignment that rely on angular measurements rather than distances. T1 SPI has been shown to correlate more accurately with health related quality of life (HRQOL) scores than the SVA, perhaps because SPI accounts for the relationship of the pelvis and lower extremities to the spine by measuring the offset of T1 from the pelvis in relation to the femoral heads and not the sacrum [14].
Recent studies have demonstrated the importance of pelvic parameters, including pelvic incidence (PI), pelvic tilt (PT), and sacral slope (SS), in assessment of sagittal spinal alignment [14, 15]. Progressive positive sagittal malalignment can lead to increased pelvic retroversion (increased PT) as a means of attempting to compensate for sagittal malalignment. Although increased PT may help to mitigate positive sagittal malalignment, abnormal pelvic retroversion can adversely affect ambulation and increase energy utilization, with resulting negative impact on HRQOL [16–18]. Irrespective of the etiology, in patients with adult spinal deformity, there is a strong correlation between sagittal malalignment and pain and disability based on standardized measures of HRQOL [19]. In a recent study, Lafage et al. showed that T1-SPI had the strongest correlation with HRQOL followed by SVA and PT [14]. Correction of both SVA and PT has been reported to result in better outcome scores than correction of SVA alone [14, 15]. Schwab et al. recommended that the goal of surgical correction for sagittal malalignment, based on normative data and HRQOL-based postoperative outcomes, should be SVA <50 mm, T1 SPI <0°, PT <20°, and lumbar lordosis within 9° of PI [20]. The results of a study from Blondel et al. [21] that assessed the amount of sagittal correction needed for patients to perceive improvement in HRQOL scores were consistent with the proposed correction goals proposed by Schwab et al. for realignment surgery. The best HRQOL outcomes for adult spinal deformity patients with severe sagittal plane deformity were achieved with correction >120 mm of SVA or at least 66 % correction of the SVA [21]. Furthermore, they found that the greater the magnitude of correction of SVA, the better the improvement was in SRS-pain and ODI scores. The goal of surgery should be to have a complete or near complete correction of sagittal malalignment if feasible; partial correction may in some cases increase the risks of instrumentation failure, proximal junctional kyphosis, and suboptimal improvement of HRQOL [20, 21]. Corrective surgery for fixed sagittal imbalance or kyphoscoliosis often involves osteotomies such as SPO, PSO, or VCR with transpedicular segmental instrumentation. The use of osteotomies to restore sagittal spinopelvic alignment in adults depends on the pathology, patient age, medical comorbidities, and experience of the surgeon. To effectively decide which osteotomy or combination of osteotomies to employ for deformity correction, it is important to have a working appreciation for the applications and limitations of all three key posterior osteotomies (SPO, PSO, and VCR).
8.1.1 Smith-Petersen Osteotomy
Smith-Petersen et al. first described SPO in 1945 for correction of fixed sagittal deformity in patients with rheumatoid arthritis [22]. It has been referred to as “Chevron” osteotomy, “extension” osteotomy, or “Ponte” osteotomy. SPO involves removal of the posterior bony elements including the bilateral facet joints, inferior portion of the lamina, and inferior portion of the spinous process, and removal of the posterior ligaments at the osteotomy level (Fig. 8.2a) [4, 8, 23]. The osteotomy is closed with the axis of rotation at the posterior aspect of the disc space, which results in widening of the anterior disc space and disruption of the anterior longitudinal ligament and hence requires a mobile disc space (Fig. 8.2b). It is usually not effective if the disk space is immobile, as in the case of bridging anterior osteophytes [8]. SPO produces shortening of the posterior column and lengthening of the anterior column, with attendant risks, including vascular, neurological, pseudarthrosis, or gastrointestinal complication [4, 8, 22]. One modification of the SPO procedure is to use an interbody graft or spacer in the middle or anterior third of the disk space and then to compress posteriorly using the graft as a fulcrum; this provides a greater degree of lordosis, with less risk of compromising the neural foramina [8].
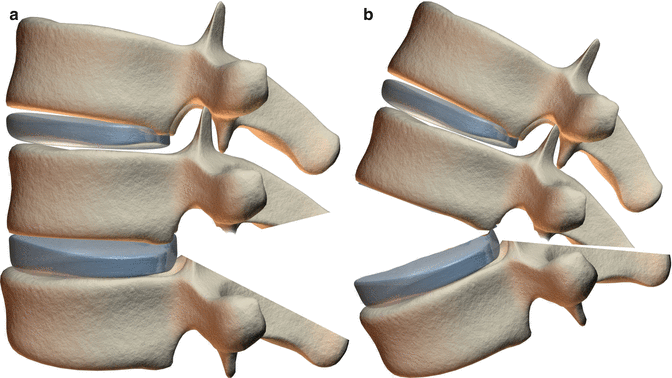
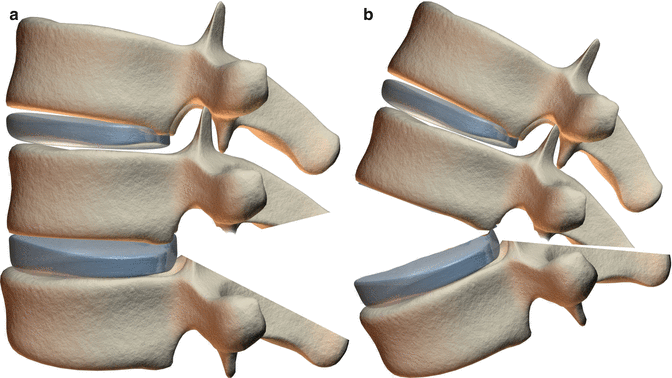
Fig. 8.2
(a, b) Smith-Petersen osteotomy: Correction is obtained though disruption of the disc space and anterior longitudinal ligament, causing lengthening of the anterior column
The amount of correction typically achieved with SPO is approximately 10° per level, or 1° of correction for each millimeter of bone removed [1, 4, 8, 22]. There have been reports of aggressive techniques of posterior extension osteotomy with anterior osteoclasis achieving more correction, but this is associated with higher risk of complications. SPO is typically indicated for cases with long, gradual sweeping deformity, with mobile intervertebral disc spaces, and no more than moderate elevation of SVA, features typically not associated with rigid deformities [24]. The classic indication for SPO is Scheuermann’s kyphosis, but it can be used in the treatment of mild iatrogenic fixed sagittal imbalance (6–8 cm) [8, 24]. The role of the SPO is limited in fixed sagittal deformity as these osteotomies have limited effectiveness in sharp, angular kyphosis and are not effective in the presence of anterior bridging osteophytes or across previously fused segments due to lack disc mobility. In addition, SPO is not without risks, as anterior column lengthening puts vascular structures at risk, particularly in the elderly and in patients with ankylosing spondylitis, who may have calcified major vessels [4, 8]. There is also risk of gastrointestinal complication secondary to superior mesenteric artery syndrome. Neurological complications have been reported in as high as 30 % of cases, mainly radiculopathy secondary to compression of nerve roots in the foramen from closure of the SPO [4]. Other complications include, but are not limited to, pseudarthrosis, bleeding, durotomy, and pedicle fracture. Compared with three-column osteotomies, SPOs are usually associated with less operative time, blood loss, and rates of neurological complication [25].
In an attempt to cause less disruption of the anterior column, polysegmental osteotomy (Fig. 8.3a) can be performed to correct sagittal deformity. Hehne et al. first described polysegmental lordosis osteotomy, with posterior osteotomy at each level, producing approximately 10° of correction per segment [26]. By closing multiple posterior osteotomies over multiple segments with transpedicular fixation, a more gradual correction of kyphosis is achieved with less disruption of the anterior longitudinal ligament (Fig. 8.3b) [4, 8, 23]. This technique has similar limitations as the standard SPO for correction of rigid deformities including limited effectiveness in the setting of a previously fused level, bridging anterior osteophytes, or sharp kyphosis.
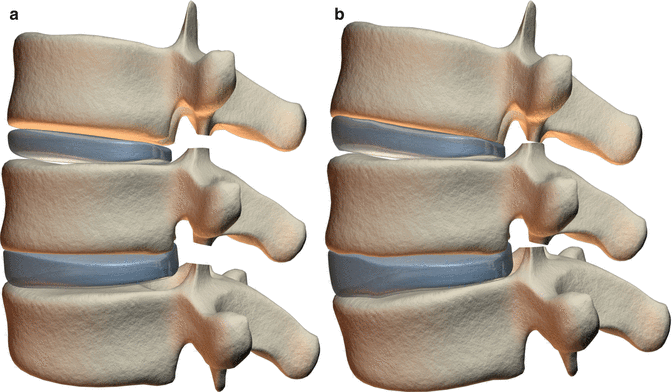
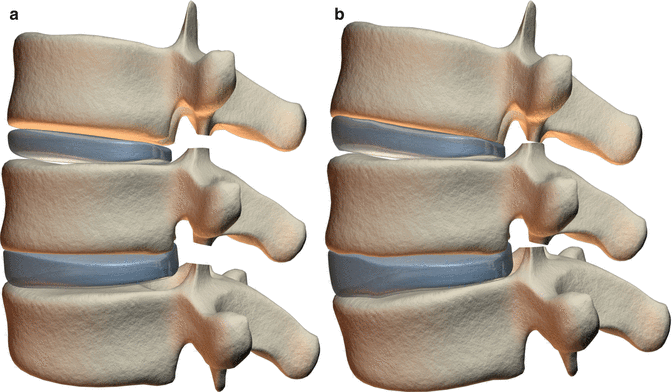
Fig. 8.3
(a, b) Polysegmental posterior osteotomy: Correction is obtained through multilevel disc deformation, with only limited or no disruption of the disc space and anterior longitudinal ligament
8.1.2 Pedicle Subtraction Osteotomy
PSO is typically used to correct fixed sagittal deformity with sharp or angular kyphosis in patients with SVA >8 cm and is often applied for the treatment of acquired or iatrogenic flat back, ankylosing spondylitis, and for rigid deformities that lack anterior flexibility needed for SPO to be effective [4, 23, 24]. PSO can be technically challenging, with substantial blood loss from the epidural venous plexus and cancellous bone during the osteotomy, and it is more commonly performed below the conus in the lumbar region to reduce neurological complication from thecal sac manipulation and wedge closure, but can be performed in thoracic and cervical level if indicated but with increased risk [1, 10, 24, 27]. If it is performed in thoracic or cervical spine, extra precaution should be taken when working around the spinal cord and the thecal sac should not be retracted [24]. Resecting a portion of the rib bilaterally along with the costovertebral joints during PSO in the thoracic spine can help to facilitate a safe approach to the vertebral body laterally without thecal sac retraction. PSO tends to be avoided in distal lumbar vertebra because there are fewer fixation points available distally [24]. PSO is most commonly performed at L2 or L3, as these levels are generally below the conus and have a sufficient number of fixation points distally. Nevertheless, the vertebral level of the PSO should be guided by the type and location of the pathology.
In patients with focal fixed-angle sagittal deformity, PSO is typically performed at the level of kyphosis; however in the absence of focal thoracolumbar kyphosis, lumbar PSO is the preferred option. In addition to providing greater correction of deformity, PSO has an advantage over SPO, as it does not require a mobile anterior segment or disc space and can be performed in patients with circumferential fusion across multiple segments, previous laminectomy, or area of rotation, although these features do potentially increase the technical difficulty and the risk of complications [24]. The extensive cancellous bony contact secondary to wedge osteotomy increases stability and reduces the risk of pseudarthrosis. Also, by performing an asymmetrical wedge resection osteotomy correction of both sagittal and coronal plane deformity can be achieved. Extended PSO is a modification that involves extension of the bony resection cranially to include the rostral vertebral endplate with removal of the rostral intervertebral disc. The wedge osteotomy is closed over the vertebral endplate of the rostral vertebral body providing a greater degree of correction [3, 4, 7]. If necessary, an interbody spacer can be placed in the middle or anterior third of the disc space to act as a fulcrum to help prevent compromise of the neural foramina.
The degree of deformity correction with PSO has been studied in multiple cadaveric, clinical, and radiographic studies. Li et al. obtained a mean of 36.4° correction with standard PSO and a mean of 48.5° correction with the modified extended PSO in a cadaveric study [4, 28]. Multiple clinical studies have shown deformity correction between 26.2° and 40.1°, with an average correction of 32° with PSO [1, 2, 4, 11, 23, 24, 29, 30]. The expected correction for PSO in the thoracic spine is less than in the lumbar spine, likely due to the shorter heights of thoracic versus lumbar vertebral bodies.
8.1.3 Posterior Vertebral Column Resection
MacLennan first described vertebral column resection in 1922 for treatment of severe scoliosis [31]. Suk et al. developed the posterior-only approach for VCR, with complete resection of one or more vertebral segments through a posterior-only approach, with the goal of achieving sagittal and coronal balance with shortening of the spinal column [32–34]. This technique involves stabilization of the vertebral column with transpedicular screw fixation, followed by compete resection of all posterior elements at the level of the VCR, the vertebral body at the apex of deformity, and the adjacent rostral and caudal intervertebral discs [34]. In most cases, anterior fusion is performed via the posterior approach with structural support using an anterior cage that preserves anterior column height and enhances the degree of correction [4].
VCR is a complex procedure with significant risk of morbidity and neurological injury, especially when performed in the thoracic and upper lumbar region, as the spinal cord and conus may be vulnerable to injury during vertebral body resection, and they may be already compromised secondary to the deformity [31]. Therefore, VCR is generally reserved for severe, complex, rigid three-dimensional deformities that are not amenable to treatment with other techniques. It is primarily used for severe fixed sagittal deformity with severe coronal plane deformities, congenital kyphosis, hemivertebra, L5 spondyloptosis, and resection of spinal tumor [4, 6]. Suk et al. [34] reported correction of 61.9° in the coronal plane and 45.2° in the sagittal plane, whereas Lenke et al. [35, 36] in their two studies, noted major curve improvements of 57–61° in scoliosis cases, 45–56° in global kyphosis, 59–51° in angular kyphosis and a combined 98–109° in kyphoscoliosis cases. VCR remains the most powerful method for three-dimensional deformity correction, but this technique is associated with relatively higher risk of morbidity and neurological injury [25].
8.2 Osteotomies and Complications (Table 8.1)
Table 8.1
Examples of complications of lumbar pedicle subtraction osteotomy
Early complications | Late complications |
---|---|
Transient or permanent neurological deficit | Pseudarthrosis |
Excessive blood loss/coagulopathy | Junctional kyphosis |
Dural tear | Rod fracture |
Postoperative respiratory distress | Breakdown of the L5-S1 disc distal to long posterior fusion |
Wound infection | Prominent iliac screws |
Deep vein thrombosis | |
Incomplete correction | |
Upper extremity compartment syndrome | |
Brachial plexus injury | |
Myocardial infarction | |
Fluid overload | |
Great vessel injury | |
Ileus |
Even though potential benefits of surgery for adult scoliosis patients have been shown in multiple studies [37–42], these procedures are not benign and need careful planning and understanding of the risks and benefits by both patients and surgeons [40]. Smith et al. reviewed the SRS morbidity and mortality database for cases of thoracolumbar fixed sagittal plane deformity (FSPD) and analyzed the short-term complication rates in patients undergoing osteotomy, based on correction technique, surgical approach, surgeon experience, patient age, and history of prior surgery [25]. A total of 578 cases of FSPD were identified, of which 402 (70 %) were treated with an osteotomy, including 215 with PSO, 135 with SPO, 19 with anterior discectomy with corpectomy (ADC), 18 with VCR, and 15 with unspecified osteotomies. There were 170 complications (29.4 %) in 130 patients, and a mortality rate of 0.5 %. The more common complications included durotomy (5.9 %), wound infection (3.8 %), neurological deficit (3.8 %), implant failure (1.7 %), wound hematoma (1.6 %), epidural hematoma (1.4 %), and pulmonary embolism (1 %). The overall complication rates associated with cases including an osteotomy were significantly higher than those not requiring osteotomy (34.8 % vs. 17 %), and this remained significant after adjusting for patient age, surgeon experience and history of prior surgery. There was a progressive increase in complication rates with more aggressive osteotomy, from no osteotomy (17.0 %), to SPO (28.1 %), to PSO (39.1 %), to VCR (61.1 %). In addition, there was a higher rate of complication in patients who underwent revision procedures (24.5 % versus 18.2 %). These risks should be carefully considered in patient counseling and surgical planning. To what degree a patient needs to be corrected and how much he or she can tolerate varies with each individual. Evaluation of this risk–benefit balance should be personalized, with careful discussion with the patient. When considering surgical treatment, one should carefully consider patient age, overall health of the patient, severity of the symptoms, impact on patient quality of life, and willingness of the patient to accept the risk of surgery. A recent multicenter study has looked into the risk factors for major perioperative complication in adult spinal deformity surgery and found significantly higher rates of complications were associated with staged and combined anterior–posterior surgeries [43]. Although these complex surgical procedures have been shown on average to be significantly beneficial in treating these patients, surgery is not necessarily indicated for every patient with sagittal deformity, and nonoperative methods should generally be the first line of treatment.
8.3 PSO Indications and Contraindications
The main indication for PSO is fixed sagittal imbalance that needs more than 30° correction of lumbar lordosis [11]. Many of these patients have a history of previous fusion and a rigid flat back that requires three-column osteotomy for alignment correction. Patients with sharp angular kyphosis, ankylosing spondylitis with cervicothoracic kyphosis or thoracolumbar kyphosis, and progressive untreated idiopathic scoliosis may require PSO for adequate correction of deformity.
PSO is a technically demanding procedure, with relatively longer operative time and blood loss in comparison with SPO. When considering PSO, the patient’s overall health should be properly assessed to evaluate if he or she can tolerate the procedure. Patients with fixed sagittal deformity with severe coronal plane deformity may benefit more with a VCR. Similarly, patients with a gradual curve and an SVA <8 cm, or requiring only 10° to 20° of lordosis correction may be better treated with one or more SPOs, which are relatively less morbid.
8.4 Preoperative Clinical Assessment
All patients with spinal deformity should have a global assessment of the spine with long cassette (36-in.) standing posteroanterior (PA) and lateral radiographs, with the hips and knees fully extended, arms flexed and hands positioned along the clavicle, head facing forward, and feet placed shoulder width apart [44]. The pelvis and hip joints should be included in the film to demonstrate the true extent of the deformity. Changes in the joint angle of the lower extremity may change the sagittal alignment; hence, it is important to follow strict standard protocols for patient X-ray positioning. The lateral radiographs should include C2 to the pelvis along with femoral head. The sagittal parameters, including SVA, regional spinal alignment (lumbar lordosis and thoracic kyphosis), and pelvic parameters, including pelvic incidence and pelvic tilt, should be measured. On the PA view, the margins of the rib cage and the pelvis along with the femoral heads should be clearly visible. Coronal curves and coronal imbalance should be measured. Visualization of the ribs aids in identifying associated rib cage deformity and helps in the assessment of vertebral body rotation at the apex of the coronal curve. These radiographs can be complemented with additional studies including flexion/extension views, side bending views, supine full-length views, and use of traction or bolster radiographs to assess flexibility. Flexibility should be evaluated in all curves that are considered for surgical correction, as it helps in predicting the magnitude of curve correction that can be achieved, the number of spinal levels needed to be fused, and the need of osteotomy [12].
Advanced imaging studies, including computed tomography (CT), CT myelograms, and/or magnetic resonance imaging (MRI), are typically required to evaluate the bony anatomy, spinal canal, neural elements, vascular anatomy, and discs. Preoperative MRI and/or CT myelogram can be particularly important in patients with neurological symptoms or deficits. CT myelogram can be particularly useful in the setting of previous instrumentation, since it is often less affected by imaging artifact compared with MRI.
Dual energy X-ray absorptiometry (DEXA) scans provide valuable information regarding bone density, and patients should be treated for osteoporosis prior to surgery if feasible. Patients with kyphoscoliosis with increased SVA but low PT (inability to compensate) should be evaluated for hip or lower extremity pathology, such as hip deformity or hip flexion contracture before considering surgical correction, as failure to diagnose these pathologies can lead to poor postoperative outcome. These patients are usually referred for preoperative physical therapy for better spinopelvic alignment prior to surgical fusion. The Thomas test is helpful in the assessment of hip flexure contracture [15].
8.5 Preoperative Surgical Planning
8.5.1 Surgical Approach and Goal
Osteotomy used to correct fixed sagittal plane deformity depends on the severity and flexibility of the deformity, and whether the kyphosis is long, sweeping, and gradual, versus short and angular. The PSO has the advantage of producing substantial correction of about 30° at a single level, without lengthening the anterior column, does not require the disc space to be mobile, and provides a large area of three columns of bone contact for successful bony fusion. The goal of surgery is to achieve complete or near complete correction of sagittal spinopelvic malalignment while minimizing perioperative and postoperative complications. It has been shown in multiple studies that correction of both SVA and PT has better postoperative clinical outcome than does correction of SVA alone [14, 15]. Schwab et al. have proposed that the ideal goals of surgical correction should be SVA <50 mm, T1 SPI <0°, PT <20°, and LL within 9° of PI [20].
Preoperative planning includes determining the extent of osteotomy required at the vertebral level to achieve the predicted level of correction and determining the ideal level for the PSO. Smith et al. performed a comparative analysis using a multicenter PSO database and the available mathematical formulas used to predict optimal postoperative SVA (<5 cm) [45]. They concluded that mathematical formulas that do not include pelvic parameters and changes in the unfused spinal segment (reciprocal changes) are less reliable predictors of postoperative alignment and outcome. Ondra et al. [46–48] used a trigonometric method to predict the angle of correction required from a PSO to achieve optimal sagittal alignment, but the formula did not incorporate the pelvic parameters in the planning and failed to predict the extra correction needed in patients with increased PT. Kim et al. proposed another formula based on sagittal Cobb angle, recommending that the difference between LL and TK be a minimum of 20° (i.e., LL ≥ TK + 20°) [49]. They also did not include pelvic parameters and reciprocal changes in the unfused segments. Rose et al. modified this formula, adding PI, and concluded that PI and TK can predict the LL necessary to correct sagittal alignment with the formula PI + TK + LL ≤ 45° [50]. The least amount of LL needed to regain sagittal alignment is LL ≤ 45 − TK − PI, but this formula did not integrate the amount of PT needed to maintain upright posture; hence, patients with higher PT were at risk of being undercorrected based on this formula. Schwab et al. proposed the formula LL = PI ± 9° for patients with decreased LL but otherwise reasonable spinal contour [20, 51]. This formula was also limited by the exclusion of PT. By predicting both postoperative PT and SVA with their formula, Lafage et al. have tried to overcome these shortcomings [52, 53]. The expected PT is dependent on LL and PI and is calculated by: PT = 1.14 + 0.71 × PI − 0.52 × (Maximal lumbar lordosis) − 0.19 × (Maximal thoracic kyphosis), whereas SVA is calculated by another formula: SVA = −52.87 + 5.90 × PI − 5.13 × (Maximal lumbar lordosis) − 4.45 × PT − 2.09 × (Maximal thoracic kyphosis) + 0.566 × (Age). Smith et al. in their analysis of five formulas found that the formulas by Lafage et al. were able to predict both poor and good SVA correction with good accuracy and had the best total prediction accuracy [45]. The preoperative plan should be altered if poor outcome is predicted [52, 53]. The inclusion of spinopelvic parameters and the integration of patient age into the calculation were credited for the improved accuracy of their formula.
The decision regarding where to place the PSO depends on the site of the deformity. A patient with loss of lumbar lordosis, without thoracolumbar kyphosis, is treated with PSO below the level of conus medullaris to reduce the risk of spinal cord injury from manipulation, retraction, or wedge closure. It is usually performed at L2 or L3, and occasionally at L4, as the apex of lumbar lordosis in a sagittally aligned spine is usually at the L3–L4 disc space. If there is coexisting flexible thoracolumbar kyphosis, a PSO can be performed in the lumbar region and the fusion extended proximally in the thoracic spine with or without multilevel SPO. If there is coexisting rigid thoracolumbar kyphosis, then the PSO is usually performed at the apex of kyphosis. A PSO can be performed in the thoracic or cervical spine if indicated, but with potential increased risk of neural injury.
Lafage et al. in a multicenter clinical study found that changes in the regional spinal curvatures (thoracic kyphosis and lumbar lordosis) correlated with PSO degree of resection but not with the level of PSO [54]. The multicenter data indicated a consistent range of correction afforded through one level PSO in the lumbar region, with mean correction of 24° at L1 and L2, 25° at L3, and 22° at L4, which is less correction in comparison to other reports from a single center [1, 24, 30, 54]. This finding demonstrates that greater degrees of realignment may not be possible universally in all cases and that during surgical planning, a single-level PSO with multilevel SPO or an extended PSO should be considered for greater correction. Lafage et al. also reported in their multicenter study, that parameters associated with spinal global alignment (SVA and T1/T9 SPI) did not correlate with vertebral level of PSO, which contradicts previous reports that suggested that the vertebral level of PSO has significant impact on the degree of correction of SVA. However, previous reports did report correlation between PSO level and PT. These findings support the clinical application of their predictive formulas mentioned earlier, for planning realignment surgery. The lack of correlation between SVA and PSO level could be related to the pelvis acting as a principal regulator for subtle changes in the lumbar alignment (PSO at L1 versus L4) and needs to be further investigated [54].
Several studies have shown the importance of pelvic parameters in the assessment of sagittal spinal alignment and correction of both SVA and PT for better outcomes. Schwab et al. in their multicenter retrospective consecutive PSO case series of 99 patients evaluated the causes of failed spinopelvic alignment following PSO and reported a 23 % realignment failure rate [55]. Patients with failed PSO had significantly larger preoperative spinopelvic deformity, including larger preoperative SVA, larger PT, larger PI, and greater PI-LL mismatch. Deformities associated with larger spinopelvic malalignment must be recognized during surgical planning, as these patients should receive larger osteotomies or additional corrective procedures to avoid under correction [50, 55].
Another important factor to be considered during surgical planning for correction of sagittal malalignment is reciprocal changes. Reciprocal changes are compensatory changes that occur in the unfused spine segments and are more prone to develop when major corrections of spinal deformity are performed over fewer spinal segments [56]. Lafage et al. have reported negative impact of increased reciprocal thoracic kyphosis on postoperative SVA after lumbar PSO [57]. In 34 patients who underwent lumbar PSO with upper instrumented vertebra below T10, the mean PSO resection was 26°, lumbar lordosis increased from 20° to 49°, SVA improved from 14 to 4 cm, and PT improved from 33° to 25°. The mean increase in thoracic kyphosis was 13° within the unfused thoracic segment, but was unchanged in 11 patients, while 5 patients had favorable reciprocal changes and 18 patients had unfavorable changes. In 6 of the 18 patients with unfavorable changes, this was due to junctional failure (defined as a 10° or more increase of kyphosis over 2 vertebrae above the fusion). They found that unfavorable reciprocal changes were associated with greater preoperative sagittal malalignment, higher PI, higher preoperative PT, and greater age. Furthermore, it has also been shown that there are reciprocal changes in LL after correction of TK [56, 58]. Another important regional parameter that is often overlooked is cervical lordosis and cervical sagittal alignment. Smith et al. reported that patients with sagittal plane deformity may have increased cervical lordosis to maintain horizontal gaze and surgical correction of the sagittal alignment, results in reciprocal change with relaxation of cervical hyperlordosis [59].
Whether or not fusion is performed across the lumbosacral junction is an important decision for the overall success of the surgery. The status of the lower lumbar spine discs, the level of curve, or lumbosacral fractional curve determines whether instrumentation and fusion is warranted across the lumbosacral curve. However, patients with lumbar degenerative disorders in whom the lumbosacral junction is not included are at higher risk of breakdown in the region and worsening of sagittal malalignment. If the osteotomy is at L4 or below or if the patient is osteoporotic with poor sacral fixation, then extension of instrumentation to the pelvis using iliac screws is recommended [3].
8.5.2 Patient Positioning
Prone positioning for posterior spinal reconstructive surgery needs appropriate padding of all bony prominences, as patients are often required to be prone for an extended period of time. We typically use a radiolucent, open-frame Jackson spine table, which allows the abdomen to float freely, thereby decreasing venous pressure, and accentuates lumbar lordosis with hip extension [60, 61]. The chest pad and the hip/thigh pad should be far enough apart to facilitate lordosis correction with closure of the PSO. The arms should be properly positioned and padded to prevent plexus and other nerve injuries. Care should be taken to ensure that the face and eyes are free of pressure throughout the surgery. The patient should be placed in a slight reverse Trendelenberg position to help prevent ischemic optic neuropathy. A severely kyphotic patient, when positioned prone may not fit properly on the table and additional padding for the chest and pelvis may be required to properly accommodate the patient on the table. Some of these patients may warrant placement in Gardner-Wells tongs with gentle axial traction (10–15 lb) in order to minimize pressure on the face and eyes during the surgery. Countertraction with femoral pins may be considered in patients with significant pelvic obliquity including neuromuscular deformities. However, since femoral countertraction causes spinal distraction, it should be avoided in patients undergoing VCR and patients with angular deformity, as it may increase the risk spinal cord injury. Intraoperative fluoroscopy can be used to localize the levels after positioning and after subperiosteal exposure, as well as to evaluate for change in spinal alignment after positioning [62]. Notably some patients may have significant improvement in lumbar lordosis after positioning, which in some cases may alter the surgical plan.
8.5.3 Neurophysiological Monitoring
New neurologic deficits, including nerve root injury, cauda equina injury, and spinal cord injury, are inherent risks and among the most feared complication of spine surgery for correction of deformity [30, 63–66]. There have been multiple studies that have demonstrated that neurophysiologic monitoring can assist in early detection and possible prevention of new neurologic deficit [6–8, 67, 68]. We routinely use somatosensory-evoked potentials (SSEP), transcranial motor-evoked potentials (tcMEPs), and free-running electromyography (EMG). The combined monitoring of SSEP and tcMEPs helps to decrease false-negative rates. Transcranial MEPs are very sensitive to any changes in spinal cord blood flow, either due to hypotension or a vascular insult [9]. Also changes in tcMEPs are detected earlier than changes in SSEP, hence facilitating more rapid identification of impending spinal cord injury [9, 10]. Neurophysiological monitoring of the upper extremities helps to monitor for brachial plexus compromise secondary to positioning and also serves as a control for comparison with lower extremity monitoring.
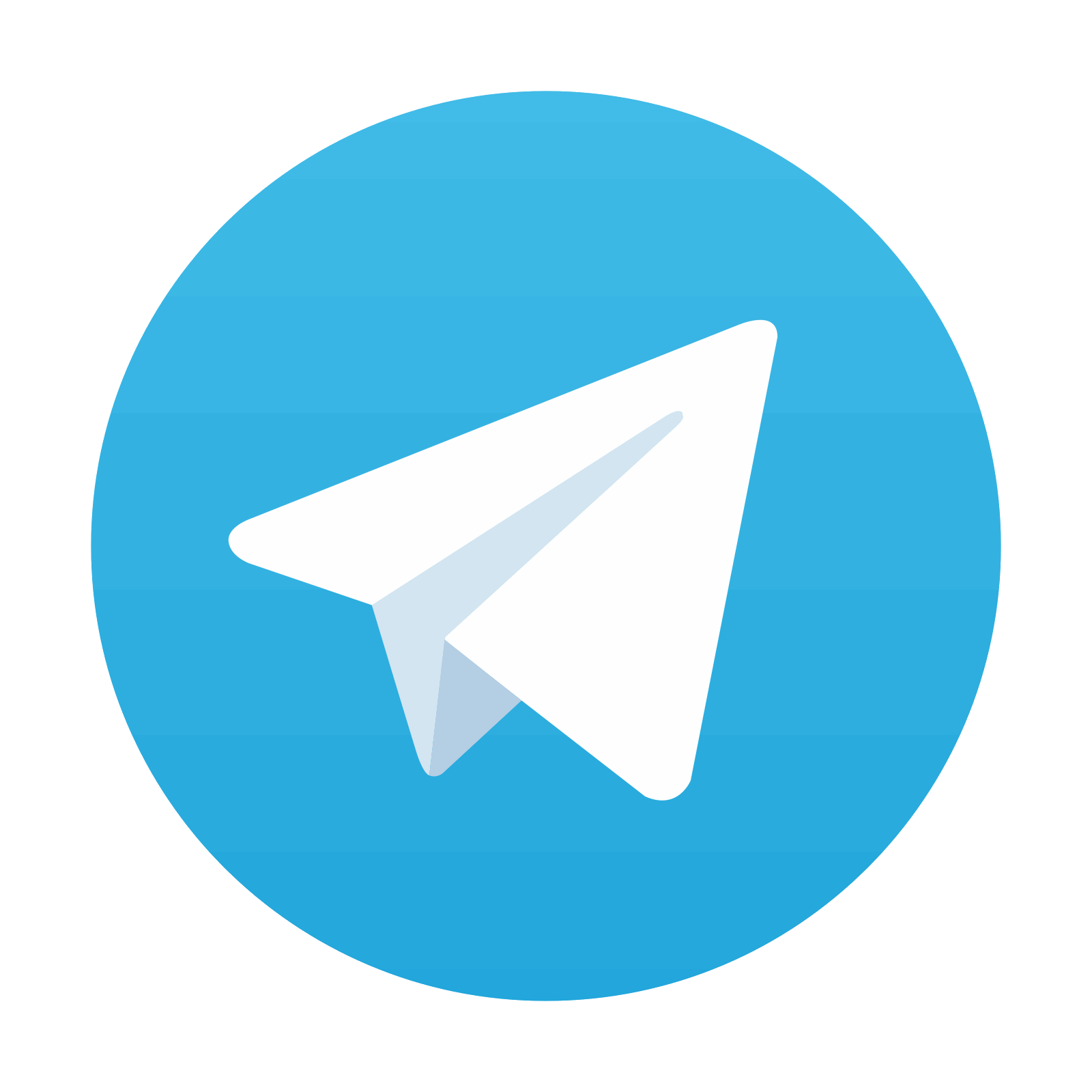
Stay updated, free articles. Join our Telegram channel

Full access? Get Clinical Tree
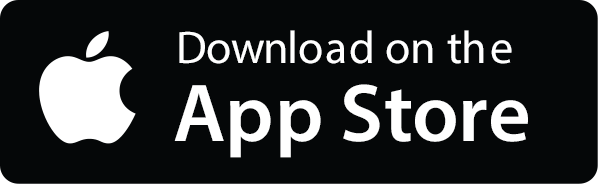
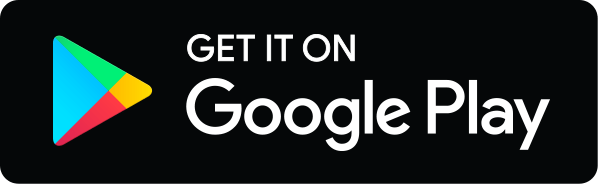