Fig. 10.1
The regeneration process occurring when a peripheral nerve gap has been bridged with a hollow nerve guidance channel can be divided into two main phases (a, b). (a) The molecular and cellular phase: When the nerve guide is initially filled with plasma exudate, extracellular matrix molecules and neurotrophic factors accumulate within its lumen (also referred to as the fluid subphase [10]). This is followed by the formation of a loose fibrin bridge between the separated nerve ends (also referred to as the matrix subphase [10]). The regenerative matrix is then populated by migrating and proliferating Schwann cells, perineurial cells (mainly fibroblasts), and endothelial cells (also referred to as the cellular subphase [10]) and, under optimized conditions, by pro-healing macrophages of the M2a and M2c phenotype [41]. (b) The axonal and myelination phase: Axonal sprouts travel along the regenerative matrix and the basal laminae provided by the Schwann cells (also referred to as the axonal subphase [10]). Once axonal sprouts have reached their appropriate targets distal to the nerve lesion, they mature and gain in diameter, which induces their myelination by neighboring Schwann cells (also referred to as the myelination subphase [10])
10.3 The Concept of Functionalization of Bioartificial Nerve Guides
The functionalization of nerve guides with regeneration-promoting substances, mainly neurotrophins or neurotrophic factors [23, 30], has been proven to increase the axonal regeneration [11, 22]. On the other hand, the optimal mixture of these proteins, as well as their optimal temporal-spatial distribution along a nerve guide, is still subject of experimental work [33]. Furthermore, it has been repeatedly discussed that neurotrophins and other neurotrophic proteins have a limited stability (short half-life time in vivo) and that the high dosages, which need to be delivered, can negatively interfere with the regeneration process [10]. Timely and accurate reinnervation of the target tissue could especially be impaired by exaggerating axonal sprouting or entrapment of regenerating axons at sites of high protein concentration [35]. Furthermore, support of the regeneration process may be achieved by supplementing an appropriate cytokine mixture [40] and/or using a biomaterial for nerve guide fabrication with immunomodulatory properties to induce invasion of pro-healing macrophages [36]. Recently, it has been postulated that the microenvironment created after peripheral nerve lesion is uniquely affecting macrophages plasticity [53]. Future studies on the interaction of Schwann cells and invading macrophages, besides those needed for myelin removal, will probably elucidate new aspects to be considered in peripheral nerve tissue engineering approaches.
Over the years, diverse fabrication techniques for preparing structured nerve implants were evaluated including computer-assisted manufacturing, laser-based tissue blotting, and advanced electrospinning or self-assembly of engineered polymers [22]. Furthermore, various surface modifications such as uniform or graduated coating or change of topography ranging from creating an uneven surface up to formation of longitudinal grooves have been evaluated [10, 46].
At first, structural modifications should provide an optimal surface for migrating host cells (so far Schwann cells are mainly considered) and support the remodeling of the bands of Büngner for an optimized axon guidance [11, 27, 39]. This type of modification with physical or topographical guidance cues is also considered to increase the speed and quality of peripheral nerve regeneration [22, 35]. In order to at best resemble the native nerve graft architecture with its axon guiding, elongated, fascicular bands of Büngner, researchers have included fibers (of micro- or nanoscale), filaments, (hydro-)gels, or sponges into the lumen of nerve guides [21, 22, 35].
Secondly, certain modifications should also increase the surface-volume relationship and as such the concentration of regeneration-promoting proteins released from the separated nerve ends and the surrounding into the graft [10]. Other types of modifications can also change the type of nerve implants from being a more or less complex guidance structure or surface for Schwann cell migration into a guiding drug delivery system with pharmacotherapeutic activity [14, 22]. The latter would be of special interest for delayed reconstruction approaches when the initial and physiological upregulation of the repair program and the reprogrammed phenotype of repair Schwann cells [29] have disappeared over time [55]. Pharmacotherapeutic attempts should aim into the incorporation and timely and spatially balanced liberation of neurotrophic molecules, cytokines, or other substances that could modify the phenotype of Schwann cells toward a prolonged support of the regeneration program. It has been demonstrated, for example, that the pharmaceutical substance FTY720P (fingolimod) promotes the phenotype of the repair Schwann cells in vitro [26], and it needs future studies to identify if this could be an appropriate pharmacological tool to be incorporated into drug delivering NGCs.
A highly active research field in the last decades has been the potential use of cell therapy in peripheral nerve regeneration. Primary autologous Schwann cells have been representing the first choice of a cellular supplement to tissue-engineered nerve grafts. But although multiple protocols have been developed (e.g., [7, 25, 31, 48]), the harvest of autologous Schwann cells still has almost the same limitation as the harvest of a complete autologous nerve graft. Therefore, the use of autologous Schwann cells as cellular substitute in a clinical setting is still only an option for the most devastating cases [34]. This is the reason why in the recent years, different types of mesenchymal stem cells (derived from bone marrow, Wharton’s jelly, adipose tissue, or skin) have been explored as potential substitutes for Schwann cells within tissue-engineered nerve grafts [15, 32, 45, 47]. In this context, the term “tissue engineering” is not limited to the combination of innovative biomaterials with regeneration-promoting cells, extracellular matrix components, or neurotrophic proteins but refers also to the supplementation of these additives to acellular nerve guides to improve also the performance of those in long-distance repair [54]. Figure 10.2 summarizes the main concepts for NGC modification toward the development of an optimized substitute for autologous nerve grafts.


Fig. 10.2
The remodeling of the peripheral nerve regeneration-supporting properties provided by an autologous nerve graft includes three main concepts: (a) Providing a three-dimensional guidance structure within the lumen of hollow nerve guidance channels, e.g., by placing a scaffold providing a channel like porosity or another longitudinal guidance structure. This could be achieved, for example, by the use of hydrogels, micro- or nanotubules (left), and micro- or nanofibers (center) or by the introduction of longitudinal films or grooves (right). (b) The integration of neurotrophic molecules, cytokines, or pharmaceutical substances that are either bound to the biomaterial or encapsulated for coordinated release should result in the induction of an optimized and prolonged support of the regeneration process. (c) The incorporation of support cells aims to provide additional guidance for regrowing axons and to deliver a regeneration-promoting milieu
10.4 The Limitations for Translation of Experimental Work into Clinical Application
Although so many innovations arose and so diverse material science approaches have been undertaken, only a very limited number of new products have been translated “from bench to bedside” into a clinical use in the last years. The reasons for this may be as manifold as the engineering ideas.
10.4.1 The Perniciousness of Multidisciplinary Approaches
At first, material scientists may not always be appropriately introduced by their collaboration partners to the specific needs of the biological system they are asked to develop biomaterials for. This can lead to discrepancies, and although the most modern fabrication techniques are used, the developed material compositions may become inappropriate. One example we have experienced in our own group is the properties of an electrospun polycaprolactone nerve guide that, although the qualification of this synthetic polymer has been proven before [23], did in our hands induce a massive and deleterious foreign body reaction upon suture between separated nerve ends [12]. Another example is the natural polysaccharide chitosan, which was already in the 1990s used as biomaterial for nerve guide fabrication but demonstrated insufficient stability to be further processed into a clinical product [16]. Anyhow chitosan was further investigated for its use as a biomaterial for neural repair [20], and finally, about 20 years later, an optimized nerve guide was developed [24] and registered for clinical use (nerve gap repair <3 cm) in Europe and the USA (see Chap. 5). A third example, again from our own laboratory, is the use of a hyaluronic acid-based laminin containing hydrogel, originally developed to support regeneration of vascular and neural tissue and with favorable properties for organotypic in vitro cultures of sensory dorsal root ganglia [43, 58]. Surprisingly, this specific hydrogel did, instead of supporting the regeneration process, impair peripheral nerve regeneration [38]. In general, hydrogels are used to provide a matrix in which invading or transplanted cells feel as confident as in their physiological destinations. In the context of peripheral nerve regeneration, the hydrogel should support the migration of Schwann cells and other support cells, like, e.g., the pro-regenerative macrophages mentioned before. But when it comes to the questions of how cell-friendly the hydrogel filler and how porous the wall of the NGC should be, specific interactions have to be considered. A recent study demonstrated that when the porous properties of the NGC wall allow uncontrolled infiltration of the cell-friendly hydrogel filler with fibroblasts, this will negatively interfere with the nerve regeneration process [13]. Interestingly, a much simpler modification of a hollow single lumen chitosan nerve guide, namely, the longitudinal introduction of a central chitosan film, was much more successful in increasing the regeneration outcome across a critical defect length of 15 mm in the rat sciatic nerve after immediate [37] and after delayed nerve reconstruction [52]. It can be expected that translation of this two-chambered chitosan nerve guide design into a medical product will be done in a reasonable period. As another example for fruitful collaboration between material scientists and clinical scientists, a more complex, microstructured collagen nerve guide has been developed recently. This specific nerve implant allowed successful nerve repair across a 20 mm sciatic nerve gap in the rat [4] and can also be filled with mesenchymal stem cells for cellular regeneration support [3]. For the latter nerve guide, first results from a clinical study are expected in not later than 1–2 years’ time.
10.4.2 The Perils of Preclinical Work
Another obstacle for the translation of all the recent innovations into a promising new medical product, likely, is the fact that many attempts are not adequately evaluated. Simple biocompatibility studies may convince material scientists in the first step, but the novel biomaterials may reveal non-appropriate properties when tested in a more comprehensive way in challenging preclinical models. The highest potential for a propagation of a novel nerve guide into a medical product for clinical use have those approaches that have proven their regeneration-supporting properties in comprehensive in vitro and in vivo studies. Reports that conclude only from biocompatibility studies with glial cell lines, for example, that a biomaterial is very promising for the fabrication of a NGC, are of minimal value for clinical scientists. In vitro studies utilizing cell lines can indeed reveal important information, but the cell lines need to be appropriate for the specific field of neural regeneration addressed. And for a more substantial indication of the biomaterial properties, those studies need regularly to be followed by evaluation of the behavior of primary nerve cells [19]. Also from an ethical point of view, in vitro studies are warranted to reduce the number of animals devoted to in vivo experiments, but a final comprehensive preclinical in vivo evaluation, probably also in different animal models, is unavoidable [19]. A meaningful result from in vivo studies, again, will only be obtainable when the different levels of peripheral nerve regeneration that range from proven regeneration of axons across a substantial distance over evidenced reinnervation of distal targets (including indicated specificity of this reinnervation) to, most important, functional recovery [6, 44] are appropriately evaluated. Qualified preclinical animal models should recapitulate the specific nerve regeneration processes, which also take place during peripheral nerve regeneration in human patients. The rat sciatic nerve transection and reconstruction model is the far most used in vivo system [1, 18]. Therefore, studies using this model have a higher value with regard to comparability among each other. The rat sciatic nerve model allows evaluation of the reconstruction of defect lengths up to 15–20 mm in a large variety of functional assessments [6, 44]. Although already accepted as a critical defect length model, the critical defect length in human patients is considerably longer (> 10 cm), but the rat sciatic nerve model is still the most appropriate and at best standardized model [1]. The rat median nerve model is considered to be appropriate for extrapolation toward human upper limb or digital nerve injuries and provides from an ethical point of view the high advantage of only minimal impairment for the animal well-being [28]. Rat models have further been developed to additionally address different health conditions and their impact on peripheral nerve regeneration. Studies performed in the Goto-Kakizaki rat that presents with moderately increased and clinically relevant blood glucose levels, thus resembling diabetes type 2 conditions [50, 51], deliver information important for an increasing amount of patients. The mean life expectancy of the laboratory rat additionally allows consideration of delayed nerve reconstruction approaches, a condition that is also highly relevant for a significant number of patients in which primary nerve reconstruction cannot be performed due to a seriously affected general health condition [9]. Finally, after comprehensive preclinical investigation, first inhuman experiences are eventually achievable by repairing the sural nerve after its harvest as an autologous nerve graft with the novel nerve guide that is about to be established for clinical use [5].
For the clinical scientists and clinicians interested into the latest developments and novel medical products that may become available in the nearer future, it is of outmost importance to know about the predictability of the results published from experimental work. Only with this knowledge, the curious reader will be able to conclude that an experimental approach to develop a novel nerve guide is indeed promising and deserves the enterprise to be processed toward a clinical product.
In vitro studies demonstrating the biocompatibility with primary peripheral nerve cells, primary neurons, or organotypic cultures from dorsal root ganglia or spinal cord preparations and also providing evidence for a stimulated neurite outgrowth from primary sensory or motor neurons by the tested biomaterial provide a legitimization for in vivo studies [19]. For this legitimation, it is not obligatory to demonstrate optimal biomaterial properties by complex peripheral nerve regeneration in vitro models, which evaluate neurite outgrowth from spinal cord slices to peripheral nerve segments or nerve guide material [17, 56]. The latter in vitro systems are the most refined and sophisticated ones, but on the opposite, they are difficult to establish and to propagate.
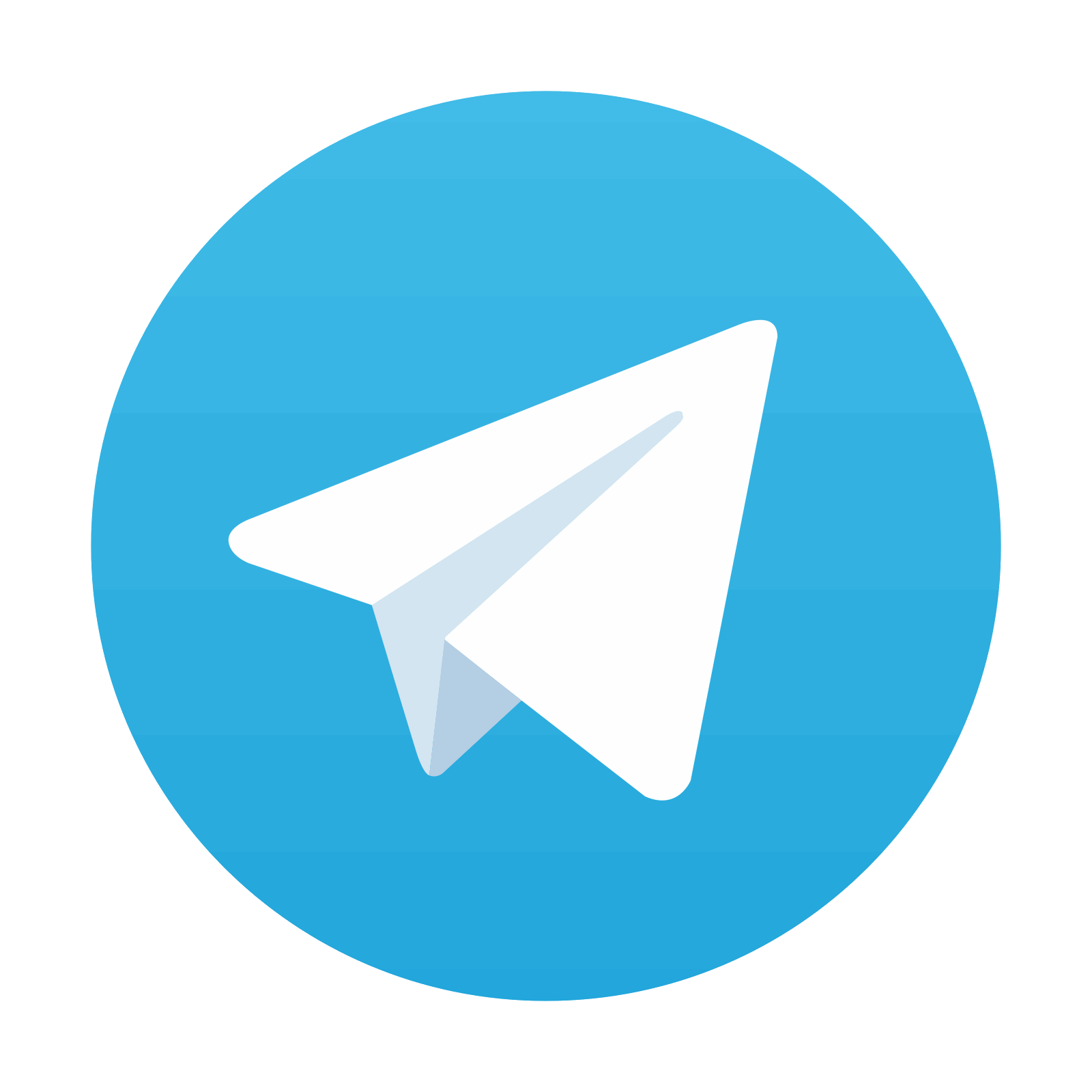
Stay updated, free articles. Join our Telegram channel

Full access? Get Clinical Tree
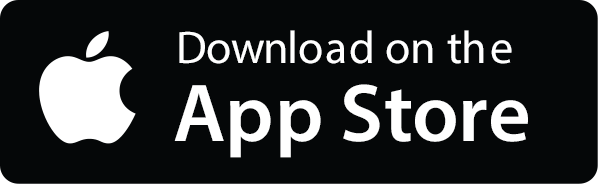
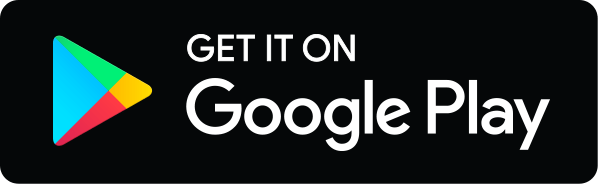