(1)
Department of Nuclear Medicine and Molecular Imaging, University Medical Center Groningen, University of Groningen, Hanzeplein 1, 9713 GZ Groningen, The Netherlands
31.1 Introduction
31.2 Aetiology
31.3 Neuroanatomy
31.4 Structural Imaging
31.9 Dopaminergic System
31.10 Noradrenergic System
31.11 Serotonergic System
31.13 Conclusions
31.14 Limitations
Abstract
ADHD is a clinically heterogeneous neuropsychiatric disorder with a childhood onset and is often accompanied by comorbidities. Symptoms consisting of inattention with or without hyperactivity or impulsivity may result from disturbances of higher cognitive control, involving multiple regions of the brain which are functionally connected. Although the value of PET and SPECT in establishing diagnosis is limited, it has added to understanding the neurobiological basis of ADHD. Investigations of cerebral perfusion and glucose metabolism during resting conditions and specific tasks have led ADHD to be associated with reduced functionality of the prefrontal cortex and the anterior cingulate cortex, as well as the basal ganglia, cerebellum and the parietal lobe. In addition, there is a growing body of literature assessing the dopamine transporter, endogenous levels of dopamine, response to treatment with methylphenidate and the relationship with genotypes in ADHD.
31.1 Introduction
In this chapter, an overview of the insights gained from PET and SPECT imaging in attention deficit hyperactivity disorder (ADHD) will be provided. Most research has focussed on the detection of functional deficits in resting or fixed conditions, task-related disturbances and alterations in neurotransmitter systems, predominantly the dopaminergic system. More recently, efforts have aimed to relate functional imaging findings to genotypes and specific behavioural characteristics, such as measurements of inattention, hyperimpulsivity and motivation.
ADHD is defined as a developmentally inappropriate disorder characterized by a combination of symptoms of inattention and hyperactivity/impulsivity (American Psychiatric Association 2013). The symptoms must be present for at least 6 months, and individuals must express several symptoms before the age of 12. In addition, the symptoms must be exhibited in at least two settings. ADHD can phenotypically be subdivided into the combined type, a predominantly inattentive type and a predominantly hyperactive/impulsive type. ADHD is the most prevalent as well as the most commonly treated neuropsychiatric condition in school-aged children (Goldman et al. 1998). In the USA, the prevalence is estimated between 5 and 8 % (Dulcan 1997) and is similar to estimates worldwide (Faraone et al. 2003). The syndrome is much more common in boys than in girls (Wolraich et al. 1996). The symptoms persist into adulthood in up to 65 % of cases, although only 16 % of children with ADHD fulfil DSM criteria for ADHD at the age of 20 (Faraone et al. 2006). Typically, symptoms of inattention (40 %) disappear at a lower rate than symptoms of hyperactivity (70 %) and impulsivity (70 %), which tend more to fade from childhood into adulthood (Biederman et al. 2000).
The symptoms of ADHD have often been ascribed to some sort of self-dysregulation. Growing emphasis has been placed on deficits of higher cognitive control, e.g. the ability to suppress inappropriate actions in favour of more appropriate ones (Casey and Durston 2006). These include motivational deficits (Johansen et al. 2009) and disturbances of executive functioning (Walshaw et al. 2010). ADHD patients often do not express symptoms of ADHD alone. Comorbidities are frequently encountered in ADHD patients, who have been reported to be at increased risk of developing delinquency, mood, anxiety and substance use disorders (Biederman et al. 1991). Oppositional defiant disorder, conduct disorder and learning disorders are most prevalent in children, whereas antisocial disorder and alcohol/drug dependency are often observed in adults (Biederman 2005).
31.2 Aetiology
Both environmental and genetic aspects are likely to be involved in the aetiology of ADHD. Strong support for a genetic aetiology has been provided by studies of familial ADHD and studies establishing linkages between genotypes an increased probability of developing ADHD. Based on numerous twin studies, heritability of ADHD is estimated 77 % (Spencer et al. 2007a). Molecular genetic studies have associated polymorphisms at the dopamine transporter (DAT) gene (Cook et al. 1995) and dopamine receptor D4 gene (DRD4) (Faraone et al. 2001) with ADHD, in particular the 10-repeat allele at the DAT1 gene and the 7-repeat allele at the DRD4 gene. However, given the prevalence of these alleles in the Caucasian population (10R DAT 1 allele p ~ 0.75 (Cook et al. 1995), 7R DRD4 allele p ~ 0.12 (LaHoste et al. 1996)), it has to be stressed that the individual contribution of these genes in developing ADHD is modest. Environmental factors contributing to the onset of ADHD symptoms have been identified in the perinatal period, including prenatal exposure to teratogens and low birth weight (Mick et al. 2002a, b). Current models assume an interplay between both genetic and environmental aspects in developing clinical ADHD (Swanson et al. 2007).
31.3 Neuroanatomy
In studies using nuclear imaging techniques, frontal and striatal structures have often been implicated in the brain localization of ADHD-related deficits (Table 31.1). Increasing evidence has come to suggest involvement of the cerebellum, giving rise to a proposed disorder of the cerebello-striato-frontal cortical network. A theoretical framework has been developed in order to facilitate interpretation of functional deficits in ADHD. Nigg et al. conceptualized ADHD as a disorder of cognitive control, in which individuals with ADHD are unable to adjust behaviour appropriately when something unexpected occurs (Casey and Durston 2006; Nigg and Casey 2005). Key brain regions implicated in cognitive control are the frontostriatal circuitry in predicting what will happen in a given context and the frontoneocerebellar circuitry in when the event may occur. Third, the frontoamygdalar circuitry is recruited in affect regulation, motivation and reactive response (Nigg and Casey 2005). The prefrontal cortex has been linked with several aspects thought to be affected in ADHD, including attention, inhibition, planning and working memory (Fassbender and Schweitzer 2006). It is interconnected with neocortical sensory and motor systems as well as subcortical structures, allowing to exert a top-down modulation of a wide variety of brain functions (Miller 2000). The striatum receives input from limbic, associative and motor areas of the prefrontal cortex (Haber et al. 2000). It can be functionally subdivided into the ventral striatum, involved in emotion, motivation and reward-guided behaviours; the associative striatum, related to cognition; and the sensorimotor striatum, modulating motor function (Haber 2003). Impulsivity in ADHD might be a consequence of impaired integration of reinforcing or avoidance stimuli from the ventral striatum and ventral amygdala, respectively, with the current behaviour of the child (Nigg and Casey 2005).
31.4 Structural Imaging
Structural imaging has revealed differences between ADHD patients and healthy controls in multiple regions of the brain, the most consistent finding being smaller sizes of particular brain structures in ADHD patients. Controversy exists as to whether this is more prominent on the left side or on the right side. In a review by Seidman et al., a decrease of 3–5 % in total cerebral volume was reported in 7 of 12 included imaging studies, mostly in the right hemisphere (Seidman et al. 2005). The most commonly reported structural abnormalities include smaller volume of the prefrontal cortex, corpus callosum (particularly posterior regions), caudate nucleus (either right or left) and cerebellum (mostly posterior and inferior lobes) (Seidman et al. 2005). Most studies have been performed in a paediatric population and it is highly questionable whether these findings can be extrapolated to the adult population. One study by Castellanos et al. addressed this issue. In this case-control study, structural differences between ADHD patients and healthy controls in childhood persisted into adolescence, except for the caudate nucleus, in which a normalization effect with age was present (Castellanos et al. 2002).
31.5 Functional Imaging: Cerebral Blood Flow and Glucose Metabolism
Rather than being described in terms of structural abnormalities, ADHD research may benefit more from a functional imaging approach. As clinical evaluation is considered the mainstay for establishing the diagnosis of ADHD, the role of functional imaging for establishing diagnosis may be limited. However, functional imaging may have great value in defining ‘endophenotypes’ in ADHD, correlating behaviour characteristics and genotypes with specific functional deficits. The role of functional imaging is further enhanced by a lack of histopathological studies of ADHD, which renders functional imaging critical to develop insight into the neural bases of ADHD. Methods for assessing functionality in ADHD include fMRI, PET, SPECT, QEEG and MRS. In children, fMRI may be the most suitable method to investigate activational neural circuitries, because of radiation dosimetry issues with nuclear imaging techniques. PET and SPECT studies in ADHD have mostly focussed on adults, except for the majority of studies assessing cerebral perfusion. PET and SPECT imaging has been performed both at resting or fixed conditions and during tasks. Table 31.1 summarizes findings on cerebral perfusion (CBF) and glucose metabolism (CMRglc) in ADHD during resting or fixed conditions. The table serves to highlight brain regions which may be functionally impaired in ADHD as well as to illustrate differences among studies in sample sizes, group characteristics, statistics and radiotracers used. Limitations, particularly of the earlier studies, include prior use of stimulant medication, small sample sizes, mixed or lacking control groups, demographic differences among groups and a region-of-interest (ROI)-based approach.
Table 31.1
PET and SPECT studies of glucose metabolism and perfusion in ADHD during resting or fixed conditions
Series | N and clinical diagnosis | Technique and radiotracer | Study design | Findings | Specifications |
---|---|---|---|---|---|
Kim et al. (2010) | 21 ADHD | SPECT and 99mTc-HMPAO | Cross-sectional | Significant hypoperfusion in the right orbitofrontal, right medial gyri, the bilateral putamen and cerebellum and in ADHD patients | All participants were Korean boys |
11 NC | All participants were stimulant naïve | ||||
Voxel-based analysis (p < 0.0005 uncorrected) | |||||
Oner et al. (2005) | 29 ADHD | SPECT and 99mTc-HMPAO | Cross-sectional | Significant reduction of prefrontal asymmetry indices in ADHD with age | All subjects were children between 7 and 14 years old |
12 Epilepsy | ROI analysis (p < 0.05) | ||||
Lee et al. (2005) | 40 ADHD | SPECT and 99mTc-HMPAO | Longitudinal and cross-sectional | Decreased rCBF in the middle prefrontal region, middle temporal region and bilateral posterior cerebellar cortices in ADHD patients. Increased rCBF in the superior parietal region and in occipitoparietal junctions. MPH treatment reduced differences | All participants were children |
17 NC | Patients were stimulant naïve at baseline | ||||
Voxel-based analysis (p < 0.01 uncorrected) | |||||
Schweitzer et al. (2003) | 10 ADHD | PET and [15O]-H2 | Longitudinal | Higher rCBF in the posterior cerebellum on MPH treatment. Off MPH treatment patterns were associated with higher rCBF in the bilateral precentral gyrus and left caudate nucleus | All participants were men |
‘Off’ condition: 8 days without MPH treatment | |||||
Voxel-based analysis (p < 0.001 uncorrected and p < 0.05 corrected) | |||||
Ernst et al. (1998a) | 39 ADHD | PET and [18F]-FDG | Cross-sectional | No difference in rCMRglc between groups. Age was significantly negatively correlated with rCMRglc in women with ADHD | Participants were adults between 18 and 56 years old |
56 NC | Prior stimulant treatment not specified | ||||
ROI-based analysis (p < 0.05 corrected) | |||||
Ernst et al. (1997) | 10 ADHD | PET and [18F]-FDG | Cross-sectional | Higher rCMRglc in the right anterior putamen and in the limbic region in ADHD. Lower rCMRglc in the left anterior putamen and left Sylvian region in ADHD | All participants were girls |
11 NC | 8/10 ADHD patients received prior MPH treatment | ||||
ROI-based analysis | |||||
Sieg et al. (1995) | 10 ADHD | SPECT and [I]-123 IMP | Cross-sectional | Relatively reduced left-to-right ROI count in global, frontal and parietal regions in ADHD | Participants were between 6 and 16 years old |
6 MC | Controls were a mixed psychiatry group | ||||
Control group tended to be older | |||||
Prior stimulant treatment not specified | |||||
ROI-based analysis | |||||
Zametkin et al. (1990) | 25 ADHD | PET and [18F]-FDG | Cross-sectional | Lower global absolute CMRglc in ADHD and in 30 of 60 ROIS | Participants were adults |
50 NC | Patients were stimulant naïve | ||||
ROI-based analysis (p < 0.05 uncorrected) | |||||
Lou et al. (1990) | 9 ADHD | SPET and [Xe]-133 | Cross-sectional | Reduced rCBF in striatal and posterior periventricular regions in ADHD. Increased rCBF in the occipital cortex in ADHD | Patients were between 6 and 15 years old |
15 NC | Prior stimulant use not specified | ||||
ROI-based analysis (p < 0.05 uncorrected) |
Analogous to structural imaging results, functional imaging reports investigating lateralization have yielded inconsistent results. Langleben et al. found an elevated left-to-right perfusion ratio in frontal and temporal regions using voxel-based 99mTc-ECD SPECT in prepubescent boys with ADHD who had most severe symptoms (Langleben et al. 2001). In addition, Oner et al. reported relative right-sided hypoperfusion in the prefrontal lobes during resting conditions in 29 drug-naïve ADHD children (age 7–13), which tended to normalize with age (Oner et al. 2005). However, the opposite (right > left perfusion asymmetry) was observed by Spaletta et al. in the dorsolateral prefrontal cortex (Spalletta et al. 2001).
31.6 Task-Related Functional Imaging
It can be seen from Table 31.1 that studies performed under resting or fixed conditions tend to show disparate results with multiple brain regions affected across studies. Although these findings could be indicative of more widespread area in the brain with altered functionality in ADHD, it might also be related to individual differences which are not ADHD specific. Therefore, it can be hypothesized that functional imaging would benefit from the integration of tasks, during which functional deficits related to ADHD become apparent. During task execution, ADHD patients may demonstrate both regions of hypoactivity and hyperactivity in comparison to controls. The former can be viewed of as a reduced capability to recruit a certain brain region which is necessarily activated in the healthy subject, whereas the latter can be seen as inefficient, requiring extra energy to perform a task. Alternatively, activation of regions which are not normally activated could reflect usage of compensatory mechanism. Discriminative tasks across (paediatric) ADHD literature typically allow assessment of components of executive functioning, such as inhibition, working memory, planning, set shifting and fluency (Walshaw et al. 2010). The studies referred to in the following paragraph make use of a specific task to identify dysfunctions related to ADHD.
Amen et al. applied perfusion SPECT both during resting conditions and a concentration task to assess functional differences between adults with ADHD (n = 27) and healthy controls (n = 20). The most significant differences were observed in the prefrontal orbits and poles. Reduced activation of these regions was associated with a post hoc sensitivity and specificity of above 74 % for discriminating ADHD from controls (Amen et al. 2008a). Ernst et al., using [15O]-H2 PET and a decision task, found that normal controls (n = 12) were more likely to activate the right anterior cingulate cortex and the left hippocampus than subjects with ADHD (n = 10) (Ernst et al. 2003). It can be hypothesized that impaired functioning of the prefrontal cortex and anterior cingulate cortex may lead to the compensatory usage of different brain regions. In correspondence, Schweitzer et al. reported increases in cerebral perfusion predominantly in occipital areas in men with ADHD during the Paced Auditory Serial Addition Task, which is used to assess working memory, whereas non-ADHD men showed increased perfusion in the anterior cingulate and frontal regions. Interestingly, the ADHD patients confirmed to have used visual strategies, consistent with activation of the occipital cortex. This finding might thus be reflective of compensatory use of visuospatial skills in ADHD (Schweitzer et al. 2000).
In order to increase statistical power, some researchers have aimed to combine results from studies to create ADHD versus control contrasts. An activation-likelihood estimation (ALE) meta-analysis performed by Dickstein et al., combining data from 16 neuroimaging studies (13 fMRI and 3 PET), revealed that healthy controls were more likely than ADHD subjects to activate the left ventral prefrontal cortex, dorsolateral prefrontal cortex, anterior cingulate cortex, bilateral parietal lobe, right thalamus, left middle occipital cortex and right claustrum, extending from insula to striatum. ADHD patients tended to activate the insula within the frontal lobe, and portions of the middle frontal cortex, left thalamus and the right paracentral lobule more (Dickstein et al. 2006). Another very recent review combining 55 task-based fMRI studies showed specific patterns of altered activity in children and in adults with ADHD. In children with ADHD, significant hypoactivity was reported in frontal regions and putamen bilaterally, as well as the right parietal and right temporal regions, whereas hyperactivity was seen in the right angular gyrus, middle occipital cortex, posterior cingulate cortex and midcingulate cortex. Adults with ADHD displayed a less widespread pattern of altered activity in relation to controls. Hypoactivity relative to controls was observed in the right central sulcus, precentral sulcus and middle frontal gyrus in subjects with ADHD. Relative hyperactivity was seen in a region with a peak in the right angular and middle occipital gyri (Cortese et al. 2012). Thus, the results from task-based functional imaging implicate that ADHD pathophysiology may involve different functionally interconnected neuronal networks (Yeo et al. 2011), e.g. reduced recruitment of the ventral and somatomotor networks in children and the frontoparietal system in adults, which may be compensated for by other neuronal systems.
31.7 Response to Pharmacological Treatment
In addition to functional imaging during resting or fixed conditions or tasks, investigating the response to pharmacological treatment may offer further insights into the neurobiological basis of ADHD. Although treatment with stimulant therapy such as methylphenidate (MPH) has been shown to improve symptoms in a majority of ADHD patients, at least on the short term, it is not yet fully clear how it affects neural mechanisms. Functional MRI studies have yielded inconsistent results, with one report mentioning upregulatory effects of MPH on frontostriatal regions (Rubia et al. 2011), whereas another study described decreased perfusion resulting from MPH treatment in these regions (O’Gorman et al. 2008). These studies were however performed in different groups (ADHD boys vs. ADHD men). One of the proposed mechanisms of MPH is that it alters more widespread patterns of activation in ADHD patients to more localized patterns similar to those activated in attention processes in normal subjects. In fact, in healthy adults, MPH has been shown to reduce the required increase in global brain metabolism to accomplish a cognitive performance task, indicating that MPH improves efficiency and allows to ‘focus’ more. Also, MPH does not seem to increase global brain metabolism when no cognitive task is performed (Volkow et al. 2008). Early PET and SPECT studies have failed to report significant influences of stimulant treatment on glucose metabolism or perfusion in subjects with ADHD, probably because a cognitive task was missing (Matochik et al. 1994; Ernst et al. 1994). Schweitzer et al. failed to observe normalization of brain perfusion after 3 weeks of MPH treatment in ADHD men (n = 10), despite normalization of behaviour during a test of executive functioning (PASAT). Instead, it was reported that MPH reduced perfusion in the prefrontal cortex (Schweitzer et al. 2004). Langleben et al. reported areas of increased perfusion of the motor, premotor and anterior cingulate cortex 36 h after MPH cessation in prepubescent boys with ADHD (n = 20) during a ‘go/no go task’. This finding suggests that discontinuing MPH treatment may disrupt the normalization effect on cerebral perfusion, probably resulting from the absence of inhibitory striatal signals (Langleben et al. 2002). Alternatively, this finding might be reflective of a withdrawal effect (Castellanos 2002). Differences in assessing the effect of MPH might be related to differences in groups, type of task (e.g. working memory requirements) or imaging techniques used.
Functional imaging has revealed several characteristics that separate responders from nonresponders to MPH treatment, offering another possibility to elucidate the effects of MPH on neuronal systems. Cho et al. reported that nonresponders showed higher rCBF at baseline in the left anterior cingulate cortex, the left claustrum, the right anterior cingulate cortex and the right putamen in comparison to responders. Only one area of decreased perfusion in the right superior parietal lobe was seen in nonresponders (Cho et al. 2007). These observations indicate that MPH treatment may be beneficial only when a perfusion deficit is present in areas on which MPH acts to increase perfusion, such as in the anterior cingulate and the prefrontal cortex. This hypothesis is supported by the findings from a study by Kim et al., who showed increased perfusion in the bilateral prefrontal cortex, caudate nucleus and thalamus in responders (20/32), regions typically involved in ADHD pathophysiology, after 8 weeks of MPH treatment (Kim et al. 2001). Amen et al. reported a superior response to MPH in ADHD patients who showed relatively small increases in rCBF in the left and right prefrontal poles during a concentration task at baseline as opposed to those who had larger increases in these regions (Amen et al. 2008b). In this case, the larger increases in prefrontal perfusion at baseline during a concentration task might indicate an already sufficient reaction of the brain to the task, without the potential of MPH to further enhance it. Rather than describing a direct effect on perfusion of MPH, these findings might reflect resolution of a hypodopaminergic state with MPH treatment, given the correlation between increased dopamine transporter (DAT) density and cerebral blood flow in the subcortical and cortical attention network (da Silva et al. 2011). In the following paragraphs, this, among other issues, will be further addressed.
31.8 Neurotransmitter Systems
Frontal and striatal structures receive large projections from the dopaminergic, noradrenergic and serotonergic system. Dopaminergic neurons are predominant in the prefrontal cortex and the striatum. The parietal lobe, part of the posterior attention centre, is predominantly modulated by noradrenaline (Levy and Farrow 2001). Treatment with drugs targeting both dopaminergic and noradrenergic systems have been demonstrated to improve symptoms of inattentiveness, executive function and working memory, whereas serotonergic treatment is generally considered to have poor effectiveness (Biederman and Spencer 1999).
31.9 Dopaminergic System
The dopamine system has been extensively investigated in ADHD. Most compelling evidence for its involvement in ADHD is the clinical benefit a substantial part of ADHD patients experiences during methylphenidate (MPH) treatment, although MPH mediates its effects though the noradrenergic system as well (Arnsten 2006). Oral MPH is the most commonly used pharmacologic treatment of ADHD. It acts as an inhibitor of the DAT, which functions to reuptake dopamine from the synaptic cleft. This has been demonstrated in vivo using [99mTC]-TRODAT SPECT (Krause et al. 2000; Dresel et al. 2000). At therapeutical levels of 0.3–0.6 mg/kg, oral MPH is likely to occupy more than 50 % of DAT (Volkow et al. 1998). As a consequence, dopamine uptake by the presynaptic neuron is blocked and the release of dopamine in the extraneuronal space is increased (Elia et al. 1990). Correspondingly, PET imaging with [11C]-raclopride has shown that MPH treatment results in increased levels of endogenous dopamine in the striatum, both in ADHD patients and healthy controls (Volkow et al. 2007a; Rosa-Neto et al. 2005). This is reflected by a decrease in binding potential (B max/K d) of [11C]-raclopride, resulting from increased competition from endogenous dopamine (Laruelle 2000). Measurements of DAT availability, D2/D3 (postsynaptic) receptor availability and [11C]-raclopride binding potential have been correlated with symptoms of inattentiveness and hyperactivity (Table 31.2), suggesting involvement of the dopamine reward pathway in the pathophysiology of ADHD (Volkow et al. 2009).
One of the most widely accepted theories concerning ADHD is the dopamine deficit theory (Levy 1991). According to this theory, there is a reduced level of striatal extrasynaptic dopamine in ADHD. This, in turn could be either due to high DAT activity or low dopamine release. Indeed, early studies assessing the DAT with SPECT have shown significant increased striatal DAT density in ADHD patients when compared to controls (Krause et al. 2000; Dresel et al. 2000; Dougherty et al. 1999; Cheon et al. 2003; Larisch et al. 2006; Spencer et al. 2007b). Increased DAT binding was also observed in the right caudate nucleus using [11C]-altropane PET, a radiotracer with considerably higher specific DAT binding than [99mTc]-TRODAT-1 (Spencer et al. 2007b). Although increased striatal DAT density has supposedly been one of the characteristic ADHD-related neural dopaminergic abnormalities, there have been several reports finding no increased DAT density (van Dyck et al. 2002) or reduced striatal DAT density in ADHD patients (Volkow et al. 2007b, 2009; Hesse et al. 2009). A recent meta-analysis, combining results from nine studies, has shown an overall increase of 14 % in DAT binding among ADHD patients (Fusar-Poli et al. 2012). However, the authors conclude that a confounding factor was present in the finding that drug-naïve ADHD patients expressed significantly lower DAT values than those who were previously treated. Accordingly, Feron et al. found that previous MPH treatment may result in higher than pretreatment DAT binding (Feron et al. 2005). Another report demonstrated, contrastingly, a lower DAT binding effect of previous MPH treatment (Vles et al. 2003). It has been hypothesized that DAT density may vary over time, functioning to maintain homeostatic tonic levels of synaptic or extrasynaptic dopamine, e.g. low levels of dopamine require low levels of DAT, whereas high levels of dopamine require high levels of DAT (Swanson et al. 2007). From this point of view, it can be seen that low levels of DAT, such as in drug-naïve ADHD patients, might indicate the presence of a dopamine deficit. Other factors besides prior use of stimulant medication that that might influence DAT binding include age (7 % decline per decade (Larisch et al. 2006)) and smoking (decrease of DAT binding (Krause et al. 2002)).
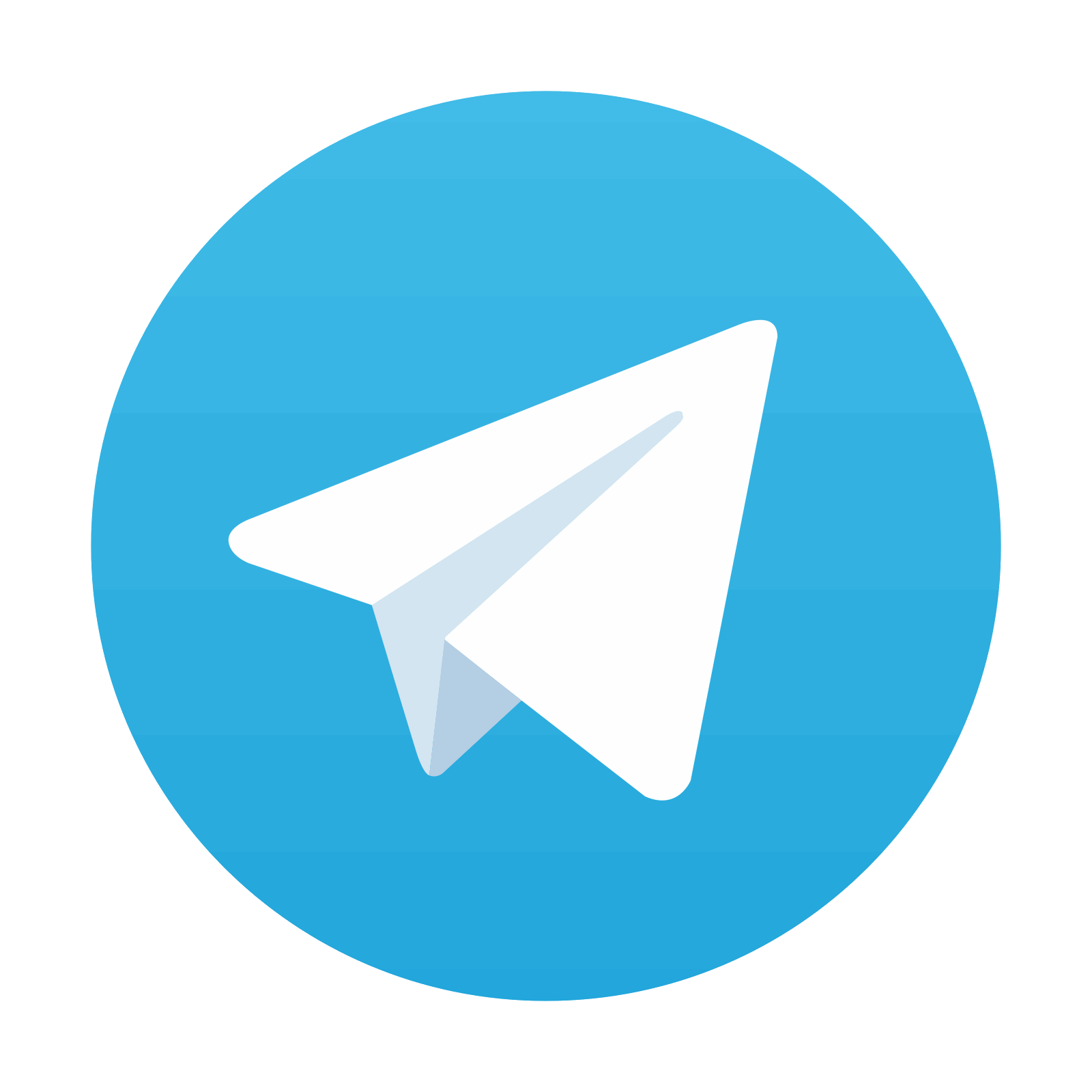
Stay updated, free articles. Join our Telegram channel

Full access? Get Clinical Tree
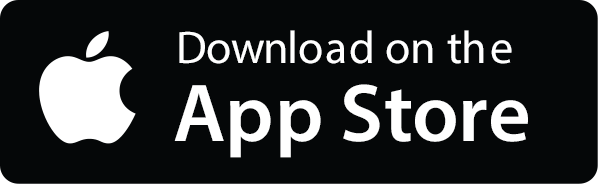
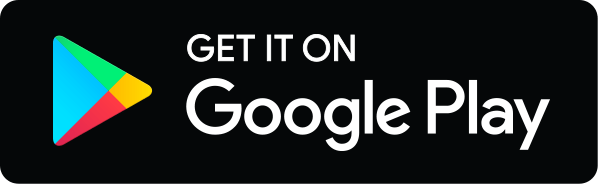