Author
Radiotracer used
Number of patients, type of disorder (responders)
Healthy controls
Timing of second scan after completion of ECT
ECT: number of sessions, type of stimulation
Areas of decreased activity on posttreatment scan
Areas of increased activity on posttreatment scan
Areas of correlation with HDRS changes
Suwa et al. (2012)
FDG
13 MDD, 3 BD (12)
11
12 days
10, bilateral
Frontotemporal neocortex
Right medial temporal, amygdala, pons
None
Reininghaus et al. (2012)
FDG
12 MDD (3)
None
1–7 days
8; bitemporal (9 patients), right unilateral (3 patients)
None
Left temporal lobe (marginal increase)
None
Nobler et al. (2001)
FDG
6UP, 4BP (10)
None
5 days
6–25,bilateral
Bilateral superior frontal lobe, dorsolateral and medial prefrontal cortex, bilateral parietal regions, posterior cingulate gyrus, left inferior temporal lobe
Occipital
–
Yatham et al. (2000)
FDG
6UP (5)
None
7 days
8–12, uni-/bilateral
None
None
None
Henry et al. (2001)
FDG
6UP and BP (3)
None
2–7 days
6–10, bilateral
Frontal lobes, bilateral parietal regions
Right basal ganglia, occipital, brainstem
Decrease in the right parietal, right anterior, left posterior frontal
Volkow et al. (1988)
FDG, 15O-H2O
4UP (3)
None
24 h
6–11, bilateral
Bilateral frontal cortex (not statistically significant)
None
–
Guze et al. (1991)
FDG
4BP (4)
None
1 day: 3 patients, 112 days: 1 patient
6–11, bilateral
None
Day 1: none
–
Day 112: middle frontal gyrus, parahippocampal gyrus
Yuuki et al. (2005)
FDG
4 MDD, 3 BP (7)
10
1 month
6–20, bilateral
Bilateral medial frontal cortex
Left occipital, parietal lobe
–
Sermet et al. (1998)
11C-MET
8 MDD (4 underwent both pre- and post-ECS scan)
5
3 h
Single, bilateral
None
Global bilateral cortical cellular protein metabolism hyperactivation
–
Takano et al. (2007)
15O-H2O
6 MDD (6)
None
(1) Pre-ECT under anesthesia, (2) during ECT, (3) post-ECT 10–30 min
5–12, bilateral
Post-ECT: return of global CBF to the pre-ECT baseline after 10–30 min, increase in thalamus, anterior cingulate, dorsolateral, medial frontal cortex comparing the pre-ECT baseline
During ECT. Basal ganglia, midbrain, pontine tegmentum, thalamus, amygdala, hypothalamus, vermis, inferior frontal, parietal, and temporal cortex (comparing to pre-ECT baseline)
–
Mervaala et al. (2001)
99mTc-ECD, 123I-iomazenil
20 (ECD), 5(iomazenil) MDD (20)
None
1 week
6–11, bilateral
None
ECD: right temporal, bilateral parietal cortices
–
Lomazenil: bilateral frontal, parietal, occipital cortices, right prefrontal cortex
Segawa et al. (2006)
99mTc-ECD
8 MDD, 2 BD (6)
None
3–20 days
8–12, bilateral
Left medial prefrontal area, left limbic regions
None
No positive correlation. Statistically significant negative correlation: left frontopolar gyrus, amygdala, nucleus accumbens, globus pallidus, superior temporal gyrus
Scott et al. (1994)
99mTc-ECD
15 MDD
None
After 45 min
Single, bilateral
Inferior anterior cingulate cortex
None
–
Awata et al. (2002)
99mTc-HMPAO
9 MDD (2 weeks: 9)
9
2, 12 weeks
6–9, bilateral
None
2 weeks: bilateral anterior cingulate, caudal orbitofrontal, right insular, right posterior middle frontal gyrus to the level of controls
–
12 weeks: increased compared to baseline
Kohn et al. (2007)
99mTc-HMPAO
8 MDD (7)
25
1 week
9.9 ± 1.9, bilateral
Responders: parietotemporal, cerebellar cortices
None
–
Nonresponders: none
Elizagarate et al. (2001)
99mTc-HMPAO
10 MDD (10)
None
Intraictal during the third ECT session
6–12, bilateral
Occipital lobe (6 patients), parietal lobe (3 patients)
Temporal lobe, basal ganglia
–
Blumenfeld et al. (2003)
99mTc-HMPAO
10 MDD (10)
None
Ictal (within 30 s of the ECT stimulus) – interictal (2 min prior to ECT stimulus)
Bitemporal (8 patients), bifrontal (2 patients)
None
Bifrontal ECT: prefrontal, anterior cingulate Bitemporal ECT: lateral frontal cortex, anterior temporal lobes
–
Bonne et al. (1996)
99mTc-HMPAO
11 MDD, 9 bipolar (11)
None
5–8 days
7–14, bilateral frontotemporal
None
Responders: anterior, posterior cingulate, basal ganglia, right hemisphere Nonresponders: none
Inverse correlation between severity of depression and HMPAO uptake, positive correlation between improvement and increase in tracer uptake
Milo et al. (2001)
99mTc-HMPAO
15 MDD (5)
11
4 days
6–12, bilateral
5 excellent responders: global normalization of baseline hyperperfused regions towards normal
5 excellent responders: normalization in frontal regions Nonresponders: none
–
Nonresponders: none
Navarro et al. (2004a)
99mTc-HMPAO
14 MDD (14)
28
12 months
8–14
None
Normalization of bilateral frontal hypoperfusion, no significant difference between ECT remitters and healthy controls at long-term scan
–
Takano et al. (2006)
99mTc-HMPAO
8 MDD (8)
12
5 days (Post 1) and 1 month (Post 2)
5–10, bifrontotemporal
Decrease in right cuneus at Post 1
Post 1: right hippocampal gyrus
–
Post 2: right medial frontal
Vangu et al. (2003)
99mTc-HMPAO
13 MDD (7)
3–11 (mean 5.3 ± 2.3) days
4–14, bilateral frontotemporal
None
Responders: left frontal, anterior cingulate gyrus
–
Nonresponders: none
Bajc et al. (1989)
99mTc-HMPAO
8 MDD, 3 schizoaffective disorder
Imaging during acute ECT
Parietal, occipital cortex
Frontal, temporal cortex, basal ganglia
–
A decrease in rCBF or CMRGlu after ECT has been most commonly reported in frontal regions, the parietal regions, the left inferior and the left medial temporal lobes, and the posterior cingulate gyrus (Henry et al. 2001; Nobler et al. 1994, 2001; Suwa et al. 2012; Volkow et al. 1988; Yatham et al. 2000). Decrease of activity in the ventrolateral prefrontal and orbitofrontal cortex was also observed in responders to antidepressants (Brody et al. 1999; Nobler et al. 2001), which further implicates the involvement of the prefrontal cortex in the neuroanatomical pathway of depression.
On the contrary, several studies also reported increase in rCBF or glucose metabolism in these cortical regions (Awata et al. 2002; Blumenfeld et al. 2003; Mervaala et al. 2001; Milo et al. 2001; Ota et al. 2003; Suwa et al. 2012; Takano et al. 2006; Vangu et al. 2003). Increase in rCBF or glucose metabolism in the basal ganglia, the occipital regions, the parietal regions, and the brainstem was also observed (Elizagarate et al. 2001; Henry et al. 2001; Nobler et al. 2001; Suwa et al. 2012; Takano et al. 2007; Yuuki et al. 2005). No statistical difference in glucose metabolism was reported by Yatham et al. (2000) and Reininghaus et al. (2012) on post-ECT scans in comparison to baseline despite clinical response.
Heterogeneous results could be due to small sample size of all studies, concomitant medicaments, different therapeutic protocols, and different indications for the treatment. Further studies with larger sample sizes are needed to clarify the antidepressant mechanism of action of ECT.
35.3.4.2 Long-Term Effect of ECT
The safety and long-term effects of ECT were evaluated by several authors (Anghelescu et al. 2001; Navarro et al. 2004a, b; reviewed in Petrides et al. 2011). Navaro and colleagues found no differences in both studies in rCBF comparing healthy controls and patients 12 months after ECT treatment. Anghelescu presented a single MDD patient who received more than 60 ECT sessions in 5 years and later presented normal CMRGlu in comparison to the normal database. No clinical signs of progressive cognitive deterioration were reported in several patients, treated with ECT over many years, including a 74-year-old patient, who received more than 400 ECT treatments (reviewed in Petrides et al. 2011).
Navarro et al. (2004b) compared the long-term effect of ECT or pharmacological treatment in MDD patients. After a 12-month follow-up period of euthymia, both treatments subgroups were associated with normalization of baseline hypoperfusion in frontal regions, and no significant differences were found in frontal brain perfusion either between patient subgroups and healthy controls or between ECT remitters and antidepressant drug remitters. Long-term ECT treatment is thus not associated with structural brain lesions.
35.3.4.3 Serotonin and Dopamine Changes in ECT
The mechanisms underlying the therapeutic effect of ECT are not completely known (reviewed in Kupfer et al. 2012), and potential targets considered are brain serotonin-2 (5-HT2) and serotonin-1A (5-HT1a) receptors, as well as dopamine D2 receptors. While various antidepressant medications cause downregulation of brain 5-HT2 (Eison et al. 1991; Meyer et al. 2001; Yatham et al. 1999), it has been shown that ECT upregulates 5-HT2 receptors in rodents (Kellar et al. 1981).
The effect of ECT on human brain 5-HT2 was assessed by Yatham et al. (2010) using 18F-setoperone, an agonist with a high affinity and specificity for serotonin 5-HT2receptors (Petit-Taboué et al. 1996). Widespread reduction in brain 5-HT2 receptors was observed in patients with depression after ECT, with peak changes in the parahippocampal gyrus and the medial prefrontal cortex. The ability of ECT to further downregulate brain 5-HT2 receptors in antidepressant nonresponsive individuals may explain its efficacy in patients with antidepressant refractory depression. Downregulation of brain 5-HT2 receptors after ECT was also observed by Strome et al. (2005), who studied the effect of ECT to binding of 18F-setoperone on brain 5-HT2 receptors in nonhuman primates. The discrepancy between the effects of ECT on the brain 5-HT2 receptors in studies on humans, primates, and rodents might be due to species differences in brain 5-HT2 receptors regulation. Likewise, a widespread reduction of binding of a radioligand 11C-WAY100635, a highly selective and potent c5-HT1A antagonist to postsynaptic brain 5-HT1A receptors (Ito et al. 1999), was also demonstrated in cortical and subcortical regions, except the occipital cortex and the cerebellum (Lanzenberger et al. 2012).
In contrast, no effect of ECT on 5-HT1A receptor binding was found by Saijo et al. (2010a) using the same radioligand in nine depressed patients. The difference in the results could be due to the significantly higher number of ECT sessions, used by Lanzenberger in comparison to Saijo or the interaction between ECT and various concomitant pharmacological treatments, that might affect 5-HT1A receptor binding.
The effect of ECT on dopamine D2 receptors in human brain was studied by Saijo et al. (2010b), using dopamine D2/D3 agonist radioligand 11C FLB457. The comparison was made between D2 receptor binding in pre- and post-ECT scans in seven MDD patients and 11 healthy controls. No significant differences were seen in D2 receptor binding between patients with MDD and healthy controls. Posttreatment scans revealed significant reduction in D2 receptor binding in the right rostral anterior cingulate, suggesting that one of the mechanisms of ECT could be related to dopaminergic alteration in that area. The results were consistent with findings of Kuroda et al. (2006) in their rTMS study using 11C-raclopride PET.
35.4 Cognitive Behavior Therapy
The investigation of mechanisms of psychotherapy action has up to the last decade been only possible at the cognitive and behavioral level. The advance in neuroimaging has only recently enabled investigation of biological consequences of psychotherapy. These new findings will allow a selection of a proper psychotherapeutic treatment and follow its effects.
35.4.1 Imaging of Cognitive Behavior Therapy in Obsessive–Compulsive Disorder
Several studies identified changes of CMRGlu and rCBF after cognitive behavior therapy (CBT) in OCD patients. Elevated baseline rCBF and CMRGlu was observed in OCD patients in the orbitofrontal cortex, anterior cingulate cortex, basal ganglia, and thalami (Baxter et al. 1987; Perani et al. 1995; Nakatani et al. 2003; Nordahl et al. 1989; Yamanishi et al. 2009). The decrease of metabolism in connection to response to psychotherapy was observed in the prefrontal cortex (Brody et al. 2001; Kennedy et al. 2007; Yamanishi et al. 2009), the bilateral caudate (Schwartz et al. 1996), the head of the right caudate (Baxter et al. 1992; Nakatani et al. 2003), and the bilateral thalamus (Saxena et al. 2009), replicating the results of several studies of OCD treatment using neurosurgery (Biver et al. 1995; Sachdev et al. 2001) and pharmacotherapy (Baxter et al. 1992; Saxena et al. 2002). Good response to psychotherapy was associated with greater decrease in the right caudate metabolism compared to nonresponders (Schwartz et al. 1996; Nakatani et al. 2003). In contrast, Apostolova et al. (2010) reported increase in the right caudate nucleus in both CBT and paroxetine group of OCD patients. This was attributed to the effect of therapy on concomitant affective and anxiety disorders and the large proportion of early-onset OCD in the sample.
Posttreatment changes of activity in the dorsal anterior cingulate cortex are somewhat dubious: increase of the activity in this area was reported by Saxena et al. (2002), while decrease was observed by Brody et al. (2001) and some other pharmacotherapy studies (Perani et al. 1995; Baxter et al. 1992; Saxena et al. 2001). No changes after CBT were seen in the study by Baxter et al. (1992). Decrease in cingulate activity may not be a necessary mechanism of action for the improvement of OCD symptoms, but may still be associated with treatment response. Increase was also reported in the temporal lobes (Brody et al. 2001; Kennedy et al. 2007).
Response to CBT and antidepressant pharmacotherapy in patients with panic disorder resulted in decrease of right frontal and temporal regions in both treatment groups (Prasko et al. 2004).
Pretreatment metabolic predictors of response to CBT and pharmacotherapy were studied and the results are dubious: positive correlation was found between treatment response in the CBT-treated group and pretreatment left orbitofrontal cortex metabolism (Brody et al. 1998), the left and right lateral orbitofrontal cortex, the left dorsomedial prefrontal cortex, and thalamus (Kennedy et al. 2007). A tendency to a negative correlation exists between the relative response to treatment and the pretreatment glucose metabolism in the left orbitofrontal cortex (Apostolova et al. 2010) and in the right inferior occipital cortex and left inferior temporal cortex (Kennedy et al. 2007). Yamanishi et al. reported significant correlation of the baseline rCBF in the bilateral orbitofrontal cortex and the change in the severity score among responders (Yamanishi et al. 2009). Schwartz et al. (1996) reported significant bilateral decrease in caudate glucose metabolic rates in the CBT responders group in comparison to nonresponders.
35.4.2 Imaging of Cognitive Behavior Therapy in Depression
Brody et al. (2001) compared DMM patients treated with either CBT or selective serotonin reuptake inhibitors (SSRI) and healthy controls. Regional brain metabolic abnormalities seen at baseline tended to normalize with both treatments. Decrease in prefrontal cortex and left anterior cingulated gyrus and increase in left temporal lobe were seen. Martin et al. (2001) compared changes in rCBF after venlafaxine, an antidepressant of the serotonin–norepinephrine reuptake inhibitor class and CBT. Increase in the right basal ganglia was seen in both types of therapy, and increase in the right posterior cingulate followed CBT. Goldapple et al. (2004) compared CMRGlu changes after CBT and SSRI therapy. Widespread decrease of activity in frontal regions was associated with both types of therapy, and increase in hippocampus, parahippocampus, and dorsal cingulate was seen in CBT group.
Several treatment-dependent differences in limbic and cortical activity may reflect treatment modalities’ specific mechanisms, which are yet to be explained in future studies.
35.4.3 Dopamine and Serotonin Changes After Cognitive Behavior Therapy
Several authors explored the dopamine/serotonin system changes after treatment with CBT: psychodynamic psychotherapy in MDD patients had no effect on dopamine D2 receptor binding in the striatum in 11C-raclopride PET study (Hirvonen et al. 2011), but midbrain serotonin transporter density, marked by 5-HT1a receptor binding of 11CWAY-100635, significantly increased (Karlsson et al. 2010). Lehto et al. (2008) observed midbrain serotonin (SERT) and striatum dopamine transporter (DAT) densities after 12 months of psychodynamic psychotherapy in depressive patients. Midbrain SERT density increased significantly in atypical but not in nonatypical depression patients, while there were no changes in the levels of DAT.
These findings are consistent with previous studies that provided evidence of decrease in density of serotonin receptors in depression (Drevets et al. 1999; Hirvonen et al. 2008). Antidepressive effect of enhanced serotonergic activity is widely used in therapy with SSRI. According to similar clinical improvement in both types of therapy, we can assume that CBT enhances serotonergic activity through increased levels of SERT.
Cervenka et al. (2012) showed a direct relationship between symptom change after CBT and extrastriatal binding of 11C FLB457, a high-affinity dopaminergic D2/D3 antagonist in nine patients with social anxiety disorder (SAD). Negative correlation between change in D2 receptor binding potential and the anxiety symptoms change was found for the medial prefrontal cortex and hippocampus.
35.5 Lesioning Procedures
The origins of psychosurgery can be traced to antiquity through the practice of trephination, the procedure of craniotomy using the cylindrical saw termed the “trephine.” A trephined skull that dates to approximately 5100 BC has been identified in France, and the literature on trephination for the relief of neuropsychiatric symptoms can be dated to 1500 BC.
Swiss psychiatrist Gottlieb Burckhardt performed the first psychosurgical procedure of the modern era in 1888 by excising multiple foci in frontal, parietal, and temporal cortices in six patients. In the early twentieth century, the Estonian neurosurgeon Lodovicus Puusepp performed sections of frontal and parietal lobes, while John Farquhar Fulton and Carlyle Jacobsen presented data on calming behavioral changes associated with the resection of the anterior frontal association cortex. Portuguese neurologist Egas Moniz suggested the ablation of the frontal cortex in humans with psychiatric disease. Together with his colleague Almeida Lima, he performed the first successful psychosurgery by injecting alcohol into the white matter of the frontal lobe of a patient with paranoid delusions and anxiety. After performing over 100 of such operations, Moniz was awarded with the Nobel Prize in Medicine or Physiology in 1949 despite little follow-up or objective results.
Widespread use of lobotomy was seen in the first half of the twentieth century, popularized with the introduction of transorbital frontal lobotomy by Americans Walter Freeman and James Watts. The decline of frontal lobotomy started in the 1950s after the introduction of chlorpromazine, the first effective pharmacological therapy for psychosis, and the spreading awareness about dubious efficacy and severe side effects of the procedure (reviewed in Mashour et al. 2005; Robison et al. 2012).
Currently there are four commonly employed neurosurgical ablative stereotactic procedures: anterior cingulotomy, subcaudate tractotomy, limbic leucotomy, and anterior capsulotomy. They are typically used for affective and anxiety disorders rather than cognitive disorders in patients who are refractory to pharmacological, psychotherapeutic, or electroconvulsive therapies (reviewed in Mashour et al. 2005).
35.5.1 Anterior Cingulotomy
Anterior cingulotomy is currently the most common neurosurgical procedure for treatment-refractory psychiatric syndromes. In this procedure, anterior portion of the cingulate gyrus is stereotactically lesioned, interrupting tracts between the cingulate gyrus and the frontal lobes. This eliminates the efferent projections of the anterior cingulate cortex to the orbitofrontal cortex and to the limbic system, resulting in a 30–68 % response rate in OCD and depression (Ballantine et al. 1987; Dougherty et al. 2002; Jenike et al. 1991; Jung et al. 2006).
Two studies involving FDG PET identified cerebral metabolic correlates as potential predictors of treatment response to anterior cingulotomy.
Rauch et al. (2001) identified one locus of significant correlation of higher preoperative metabolism and better treatment response for OCD within right posterior cingulate cortex, approximate Brodmann area 31, while Dougherty et al. (2003) identified two loci, associated with a greater postoperative improvement of major depression – the left subgenual prefrontal cortex and left thalamus.
Greenspan et al. (2008) reported a case report on H2 15O PET findings of pain-evoked blood flow response in which perception of pain and temperature was assessed before and after cingulotomy for OCD. The preoperative pain-evoked activation of the bilateral middle cingulate cortex diminished postoperatively, but the activation was seen in the ipsilateral parasylvanian cortex. The study gives evidence to the functional connectivity of the hierarchical pain network.
35.5.2 Subcaudate Tractotomy
Subcaudate tractotomy is primarily used for the treatment of refractory depression. It targets a region of white matter localized beneath the head of the caudate known as the substantia innominata, interrupting the loop between the cortex and thalamus via the striatum (Shah et al. 2008).
A bifrontal stereotactic tractotomy, a procedure that destroys bifrontal pathways located beneath and in front of the head of the caudate nucleus, was used by Biver et al. (1995) to treat a 37-year-old patient with refractory OCD. Hypometabolism was seen on a posttreatment FDG PET scan in comparison to the baseline scan in areas known to be associated with depression: the medial part of the orbital cortex, thalamus, caudate nucleus, and subgenual cingulate. Surgical intervention resulted in partial clinical improvement.
35.5.3 Limbic Leucotomy
Limbic leukotomy is the combination of stereotactic lesions created in the anterior cingulotomy and subcaudate tractotomy. A response of 36–50 % was reported in 21 patients who underwent limbic leukotomy for OCD or depression (Montoya et al. 2002).
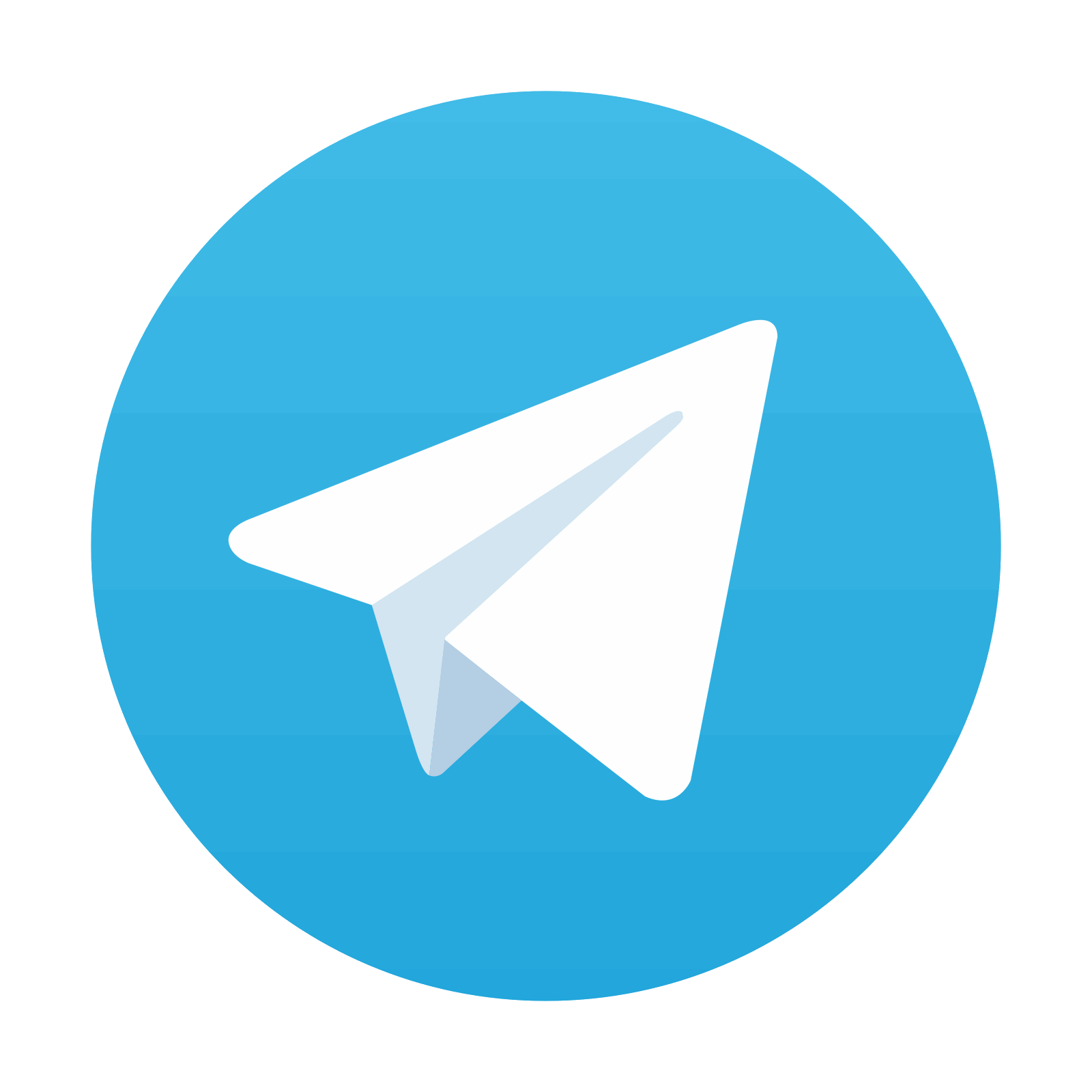
Stay updated, free articles. Join our Telegram channel

Full access? Get Clinical Tree
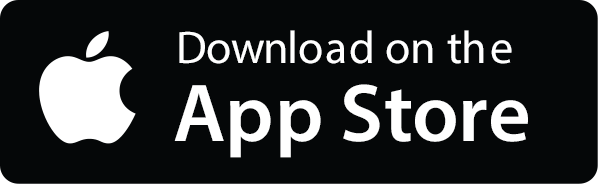
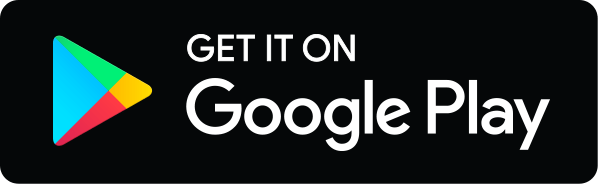