Study
Imaging technique employed
Target
Number of patients/controls
Patients receiving treatment/total number of patients
Results
Sudo et al. (1998)
PET 11C-MPB
ACh
11/21
0/11
No change
Derry et al. (2006)
PET 18F-MPPF
5HT-1A
14/0
12/14
Inconclusive in absence of control group
Eisensehr et al. (2003b)
SPECT 1PT
Presynaptic DA binding
7/7
0/7
No change
Rinne et al. (2004)
PET 11C-CFT
Presynaptic DA binding
10/15
0/10
No change
Eisensehr et al. (2003b)
SPECT IBZM
Postsynaptic DA (D2) binding
7/7
0/7
Increased striatal DA
Hublin et al. (1994)
SPECT IBZM
Postsynaptic DA (D2) binding
6/8
0/6
No change
Staedt et al. (1996)
SPECT IBZM
Postsynaptic DA (D2) binding
10/10
0/10
No change
Rinne et al. (1995)
PET 11C-raclopride
Postsynaptic DA (D2) binding
7/7
6/7
No change
Khan et al. (1994)
PET 11C-raclopride
Postsynaptic DA (D2) binding
17/32
12/17
No change
McFarlane et al. (1997)
PET 18F-PSP
Postsynaptic DA (D2) binding
6/6
0/6
No change
Joo et al. (2004)
PET 18F-FDG
CMRglu
24/24
0/24
Reduced CMRglu in hypothalami and thalamic nuclei
Dauvilliers et al. (2010)
PET 18F-FDG
CMRglu
21/21
14/21
Increase of CMRglu in limbic cortex
Yeon Joo et al. (2005)
SPECT 99mTc-ECD
rCBF
25/25
0/25
Reduced cerebral perfusion in hypothalami
Hong et al. (2006)
SPECT 99mTc-ECD
rCBF during a cataplectic attack
2/0
0/2
Increased perfusion in limbic areas, basal ganglia, thalami, sensorimotor cortices, and brain stem. Decreased perfusion in prefrontal cortex and occipital lobe
Chabas et al. (2007)
SPECT 99mTc-ECD
rCBF during a cataplectic attack
1/0
0/1
Increased perfusion in cingulate cortex, orbitofrontal cortex, and right putamen
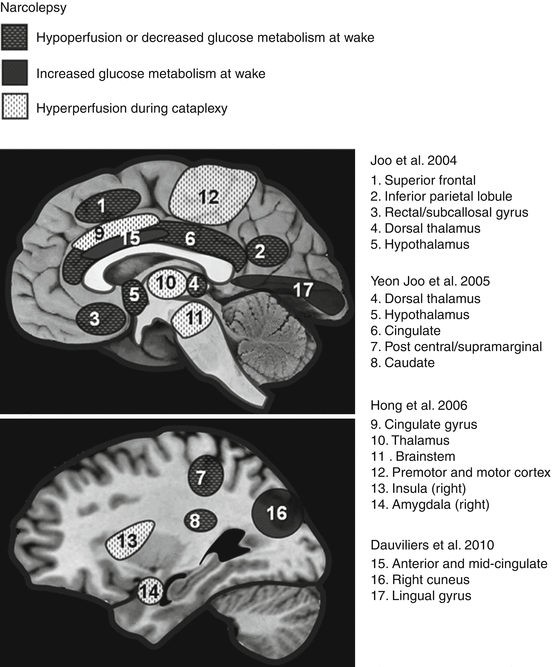
Fig. 34.1
Brain regions showing differences in CMRglu or rCBF during wakefulness in narcolepsy, as well as hyperperfusion (rCBF) during cataplectic attack (Adapted from Desseilles et al. (2008))
34.2.1 Acetylcholine, Serotonin, and Dopamine Functions in Narcolepsy
Sudo et al. (1998) focused on ACh neurotransmission in narcolepsy. They used PET with the radioligand 11C-N-methyl-4-piperidyl-benzilate (11C-MPB) in order to target the muscarinic ACh receptor. When comparing 11 narcoleptics to 21 controls, there was no difference in 11C-MPB binding in the thalamus, pons, striatum, or cerebral cortex.
Derry et al. (2006) evaluated 5-HT neurotransmission in narcolepsy–cataplexy. They used PET with 2′-methoxyphenyl-(N-2′-pyridinyl)-p–18F-fluoro-benzamidoethylpiperazine (18F-MPPF) in order to study 5-HT1A receptors. This study found an increase in 18F-MPPF binding in the anterior cingulate, temporal and mesio-temporal cortices in patients during sleep compared to wakefulness. However, this study is limited by the lack of a control group.
A few studies investigated presynaptic DA binding in narcolepsy using 123I-(N)-(3-iodopropene-2-yl)-2b-carbomethoxy-3b-(4-chlorophenyl) tropane (123I-IPT) SPECT (Eisensehr et al. 2003b) and 11C-2b-carbomethoxy-3b-(4-fluorophenyl) tropane (11C-CFT) PET (Rinne et al. 2004). However, there was no significant difference when comparing narcoleptics and controls. When looking at postsynaptic D2 receptor binding, a study found a difference between narcoleptic patients and controls using SPECT and 123I-(S)-2-hydroxy-3-iodo-6-methoxy- ([1-ethyl-2-pyrrolidinyl] methyl) benzamide (123I-IBZM). They found increased D2 binding in the striatum in seven narcoleptics. There was also a positive correlation between IBZM binding to the striatum and the incidence of sleep attacks and cataplexy (Eisensehr et al. 2003b). However, other studies using SPECT scans with IBZM were not able to replicate these findings (Hublin et al. 1994; Staedt et al. 1996). Khan et al. (1994) and Rinne et al. (1995) examined the relationship between dopamine and narcolepsy using a PET study with 11C-raclopride, but their results were inconclusive. MacFarlane et al. (1997) conducted a study using PET with l8F-fluoropropyl-spiperone (18F-PSP) ligand and were not able to find a difference in the striatal binding of D2.
34.2.2 Brain Glucose Metabolism and Perfusion in Narcoleptic Individuals
Another important aspect that several neuroimaging studies examined is the difference in narcoleptic brain activity during the day. Two studies concentrated on the assessment of CMRglu during resting wakefulness. One study in particular assessed the CMRglu of 24 narcoleptic patients and 24 normal individuals using PET with 18F-fluorodeoxyglucose (18F-FDG). They found that narcoleptics had reduced CMRglu in the bilateral posterior hypothalami and mediodorsal thalamic nuclei (Joo et al. 2004). However, this study did not include EEG measurements, for vigilance monitoring. Another study used SPECT with 99mTc-ECD and found that there was hypoperfusion in the bilateral anterior hypothalami. This study also found decreased rCBF in the caudate, superior/middle frontal gyri, postcentral gyrus, parahippocampal gyrus, and cingulate cortex (Yeon Joo et al. 2005). Both studies concluded that altered hypothalamic activity could reflect hypocretin deficiency in patients with narcolepsy–cataplexy, while the other neuroimaging patterns could be related to dysfunctions in emotional and cognitive processes. In contrast, a study conducted by Dauvilliers et al. (2010) used PET with 18F-FDG and found an increase in CMRglu in the limbic cortex (more precisely in the anterior and mid-cingulate cortex), as well as in the right cuneus and lingual gyrus. However, this last study included patients treated with psychostimulants and did not use an objective assessment of vigilance with EEG.
34.2.3 Neural Correlates of Cataplexy
Given the inherent difficulty in “catching” a narcoleptic patient in the scanner during a cataplectic episode, few studies have examined brain activity during cataplexy (loss of muscle tone). A study was conducted using technetium-99m ethylcysteinate dimer (99mTc-ECD) SPECT on two individuals suffering from narcolepsy with cataplexy. Scans obtained during a cataplexy episode were compared to those recorded during wakefulness and REM sleep. Cataplexy was associated with increased perfusion in limbic areas (amygdala, cingulate gyrus), basal ganglia, thalami, sensorimotor cortices, and the brainstem. Conversely, perfusion decreased in the prefrontal cortex and the occipital lobe (Hong et al. 2006). Increased activity in the cingulate cortex and amygdala may underlie abnormalities in the neural processing of emotions (which typically trigger cataplectic episodes), but the small sample limits the interpretation of findings. A case study using SPECT with 99mTc-ECD found an increased perfusion in the cingulate cortex and basal ganglia during an episode of cataplexy, in agreement with the previous report (Chabas et al. 2007). Dauvilliers et al. (2010) finally scanned two narcoleptic patients using PET with 18F-FDG during a cataplectic attack, but did not find any significant difference when cataplexy scans were compared to the corresponding baseline wakefulness scans of the same patients.
34.2.4 Pharmacological Treatment of Narcolepsy
Since the main symptom of narcolepsy is excessive sleepiness, medications that promote vigilance are vital in narcolepsy treatment. Psychostimulants are known to induce enhanced wakefulness as well as improvements in physical functioning; hence, this class of drugs has seen much use in treating narcolepsy. Studies involving functional neuroimaging techniques such as SPECT and PET have investigated the neural effects of these drugs in narcoleptic patients.
34.2.4.1 Methylphenidate
Methylphenidate, an amphetamine derivative, is commonly used for treating narcolepsy. One SPECT study used 133Xe inhalation to examine rCBF in narcoleptic individuals before and after treatment with methylphenidate for about 2 weeks. Administration of the drug increased rCBF during the awake state in the brainstem and cerebellar region (Meyer et al. 1980). The specificity of this finding to narcolepsy cannot be assessed, because controls were omitted in this study.
34.2.4.2 Modafinil
Modafinil is another psychostimulant drug used to promote wakefulness in patients with sleep disorders. In one experiment, 99mTc-ECD SPECT was performed when narcoleptic patients were in the awake state, both before and after a 4-week treatment with either modafinil or placebo (Joo et al. 2008). Modafinil caused a significant reduction in subjective daytime sleepiness, while the placebo did not, and patients in the on-modafinil condition showed an increase in rCBF in the bilateral prefrontal cortices (Joo et al. 2008). Thirty-two narcolepsy patients took part in this experiment, but in the absence of controls, the findings cannot be specifically applied to narcolepsy. Another experiment employed 18F-FDG PET to measure CMRglu in narcoleptic patients (Dauvilliers et al. 2010). Some of the patients were given modafinil and/or antidepressants (for treating cataplexy). Narcoleptics who received the treatment had a higher CMRglu in the cerebellum and the primary sensorimotor cortex compared to untreated patients, which contrasts with the SPECT study by Joo et al. (2008), in which modafinil was associated with a decrease in rCBF in the cerebellum. Researchers conducted another study using 18F-FDG PET to assess changes in CMRglu after the administration of modafinil (Kim et al. 2007). Seven narcoleptics patients completed the experiment. After 2 weeks of treatment with modafinil, the left hippocampus of narcoleptics exhibited an increase in CMRglu compared to pretreatment scans. Given that similar neuroimaging pattern was found with modafinil treatment in healthy volunteers (Joo et al. 2008), the specificity of this finding to narcolepsy might be questioned.
34.2.5 Summary
Generally, SPECT and PET studies did not demonstrate a consistent difference in ACh, DA, or 5-HT neurotransmission in narcolepsy. Patients had reduced activity in the bilateral, hypothalamic, and thalamic nuclei, in agreement with a dysfunction of the hypocretinergic system and an impairment of vigilance. Importantly, alterations of limbic structures were found and are in agreement with abnormalities in emotional processing. Furthermore, these imaging data are in agreement with neuropsychological studies finding symptoms of narcolepsy in patients with hypothalamic lesions (Dempsey et al. 2003; Muller 2010).
Although studies showed functional brain changes in narcoleptic patients posttreatment with the drugs discussed above, the meaning and significance of these differences still remain unclear, especially given the general lack of control and/or placebo groups. Further studies are thus needed to provide information on the specificity of these drug effects to narcoleptic patients.
34.3 Restless Legs Syndrome and Periodic Limb Movements
Restless legs syndrome (RLS) and periodic limb movements (PLM) are distinct yet overlapping sensorimotor disorders. RLS is characterized by an overwhelming urge to move the legs (and less often, the arms), especially when at rest and in the evening or at night. The compulsion is associated with persistent feelings of discomfort from deep inside the limbs (AASM 2005; Allen et al. 2003). PLM is distinguished by intermittent episodes of repeated and highly stereotyped limb movements when at rest, typically during NREM sleep (PLMS), but also occurring during wakefulness (PLMW). The same patient can exhibit both PLMS and PLMW. The movement typically consists of an extension of the big toe and partial flexion of the ankle, knee, and, less often, hip. While these movements disturb sleep and can result in arousal or awakening, patients are mostly unaware of the movements or even that their sleep has been disturbed. Diagnosis requires a polysomnographic recording in combination with a complaint such as “unrefreshing” sleep (AASM 2005; Pennestri et al. 2006).
Epidemiological studies estimate a 5–20 % prevalence of RLS (Allen et al. 2003) and a 3.9 % prevalence of PLMS in the general population (Ohayon and Roth 2002). RLS-related symptoms are responsible for sleep-onset insomnia and nocturnal awakenings in 94 % of patients (Montplaisir et al. 1997). RLS can occur in an isolated form (idiopathic) or can be secondary to (or associated with) other medical conditions, such as iron deficiency anemia, neuropathy, and Parkinson’s disease (PD) (AASM 2005; Allen et al. 2003; Pedroso et al. 2013). Depression and anxiety-related psychiatric illnesses are more prevalent in RLS and PLM patients than in healthy individuals (Pennestri et al. 2006; Picchietti 2006).
RLS and PLM frequently co-occur. However, PLM is nonspecific, occurring in isolation in healthy individuals or comorbid with other sleep disorders such as narcolepsy, RBD, and sleep apnea (Pennestri et al. 2006). Since both disorders are so closely associated, few neuroimaging studies have examined PLM alone, and instead RLS and PLM are most often considered in concert. The following section will first describe neuroimaging studies centered on RLS and will end by covering the few studies of PLM alone.
34.3.1 Restless Legs Syndrome
There are few functional neuroimaging studies of RLS. A PET study by Trenkwalder et al. (1999) involving six RLS patients and six age-matched controls measured CMRglu with 18F-FDG and found no significant differences. It is noteworthy that the patients were scanned outside of the symptomatic period.
Most PET and SPECT studies of RLS have looked for neurotransmission abnormalities using radioligands for DA and opioids. It has been shown that DA antagonists exacerbate RLS symptoms, whereas DA agonists and opioids are the major form of therapy for RLS (Stiasny-Kolster et al. 2005; Trenkwalder et al. 2008).
DA studies focused mainly on the striatum, examining both presynaptic DA transporter (DAT) and postsynaptic D2-receptor binding. Striatal DAT can be taken as an indicator of DA neuron density in the substantia nigra (SN). Some PET studies showed decreased presynaptic DA function in the striatum of RLS patients versus controls, using either 18F-dopa (Ruottinen et al. 2000; Turjanski et al. 1999) or 11C-methylphenidate (Earley et al. 2011). However, an early PET study using 18F-dopa found no such difference, albeit with a limited sample of patients (Trenkwalder et al. 1999). Furthermore, a number of SPECT studies found no difference in DAT in RLS versus controls, using 123I-2beta-carbomethoxy-3beta-(4-iodophenyl) tropane (123I-β-CIT) (Michaud et al. 2002; Mrowka et al. 2005) or 123I-IPT(Eisensehr et al. 2001; Linke et al. 2004). The discrepancy in these findings may be attributable to particular pharmacokinetic properties of radioligands used in PET and SPECT. Earley and colleagues (2011), in the aforementioned study, scanned their patients in the morning (n = 20) and evening (n = 16) and found no difference in DA according to time of day. Hence, time of day does not seem to modulate DAT binding. There was also no significant correlation between severity of RLS symptoms and DAT. Kim et al. (2012) employed SPECT with 123I-β-CIT and 123I-IBZM and, in contrast with all previous presynaptic DA studies, found an increase in DAT density in the striatum, as well as the caudate and posterior putamen.
Postsynaptic D2-receptor binding studies are also rather equivocal. A few SPECT studies used 123I-IBZM. Most found no difference (Eisensehr et al. 2001; Tribl et al. 2002, 2004), while one found a slight decrease in striatal D2-receptor binding in RLS patients versus controls (Michaud et al. 2002). Two PET studies using 11C-raclopride found divergent results: Turjanski et al. (1999) found a decrease and Cervenka et al. (2006) an increase in striatal D2-receptor binding. This discrepancy may be explained by the inclusion of a sample of RLS patients previously exposed to DA drugs in the study by Turjanski and colleagues (1999), whereas patients in the other study were drug naïve (Cervenka et al. 2006). It has in fact been shown that D2 receptors can be downregulated by chronic drug treatment, hence decreasing ligand binding (Stanwood et al. 2000). Cervenka and colleagues (2006) measured D2-receptor binding in extrastriatal structures by scanning 16 RLS patients with 11C-FLB457 and found increased binding potential in the striatum as well as in the insula, thalamus, and anterior cingulate cortex. The areas showing increased D2-receptor binding are part of the medial nociceptive system, which regulates the affective component of pain. If this system were to undergo endogenous DA depletion, one could expect upregulation of D2 receptors, just as the study showed. The authors also took measurements in the morning and the evening and found no diurnal changes in D2 binding potential. Furthermore, no significant correlation was found between RLS symptom rating and D2 binding potential. Hence, diurnal changes in RLS symptom severity cannot be accounted for by presynaptic DA transmission (Earley et al. 2011) or postsynaptic D2 binding (Cervenka et al. 2006). In a later PET study using 11C-raclopride, Earley et al. (2013) found that RLS patients had lower D2-receptor binding potential in the putamen, as well as the caudate but not ventral striatum. Interestingly, in light of the divergent results of previous PET and SPECT studies, the authors of the study deemed D2-receptor binding potential of questionable value to RLS research.
Since RLS seems to be a disorder of the nociceptive system, it follows that the opioid system, which modulates pain, may play a role in RLS. Indeed, opioid receptor agonists have been shown to improve RLS symptoms (Walters 2002). This effect may however be mediated by DA and may not necessarily reflect a deficiency in endogenous opioids (Barriere et al. 2005). In support of this, one PET study has examined opioids in RLS, using 11C-diprenorphine (a nonselective opioid receptor ligand), and found no differences between patients and controls, although the authors did find some correlations between RLS severity or pain scores and opioid binding in several brain areas (von Spiczak et al. 2005).
In addition to nigrostriatal abnormalities in DA neurotransmission, descending dopaminergic projections to the lower brainstem and spinal cord, as well as opioid receptors in the spinal cord, are also thought to play an important role in RLS pathophysiology. In addition, spinal cord lesions and peripheral neuropathies are associated with RLS (Trenkwalder and Paulus 2010). However, limitations in the resolution of PET and SPECT in these areas preclude further investigation using these imaging techniques.
34.3.2 Periodic Limb Movements
Dopaminergic transmission has been studied in relation to PLM. At the presynaptic level, Happe and colleagues (2003) measured DA transmission in 11 patients with Parkinson’s disease (PD) using SPECT with 123I-β-CIT. Patients with PD showed a stark reduction in striatal binding compared to controls, as expected. By also measuring PLMS by polysomnography, the authors detected a negative correlation between the number of PLMS and striatal DA binding values. This suggests a possible role of presynaptic DA deficiency in PD-induced PLMS. Staedt and colleagues examined postsynaptic D2-receptor binding in the striatum of PLMS patients in a few studies using SPECT and 123I-IBZM (Staedt et al. 1993, 1995a, b) and found decreased D2-receptor occupancy (Staedt et al. 1993, 1995a). DA replacement therapy can reverse this pattern and restore sleep quality (Staedt et al. 1995b).
34.3.3 Summary
PET and SPECT studies on RLS and PLM seem to indicate a hypoactivity of DA neurotransmission underlying these disorders, both at the presynaptic and postsynaptic levels. DA deficiency, in concert with CNS iron depletion, may unbalance the sensorimotor control of pain. Further research into RLS and PLM brain activation during sleep is needed to confirm these findings and shed further light on these little explored disorders.
34.4 Parasomnias
Parasomnias are characterized by undesirable physical events and experiences occurring during entry into sleep, within sleep, or during arousals from sleep (AASM 2005). They are divided into two categories: REM and NREM parasomnias. Although some forms are benign, others may result in injury and sleep disruption, severely affecting one’s life. PET and SPECT bring important contributions to the pathophysiology of parasomnias.
34.4.1 Sleepwalking
One common type of NREM parasomnia is sleepwalking, formally known as somnambulism. It “consists of a series of complex behaviors that are usually initiated during arousals and slow wave sleep (SWS) and culminate in walking around with an altered state of consciousness and impaired judgment” (AASM 2005). To date, there is only a single case studying sleepwalking with neuroimaging. Bassetti et al. (2000) hypothesized that sleepwalking is a dissociated state, consisting of both mental and motor arousal. Using SPECT, recordings were taken from a 16-year-old man in two conditions: one recording during SWS, the other 24 s after the occurrence of a sleepwalking episode arising from SWS. In both conditions, the patient was injected with 99mTc-ECD. Compared to undisturbed SWS, there was an increase in rCBF post-sleepwalking, particularly in the posterior cingulate cortex and the anterior cerebellum (Bassetti et al. 2000). Interestingly, these areas showed a decrease in activity in healthy volunteers during SWS compared to wakefulness (Maquet et al. 2000). Furthermore, Bassetti et al. compared their data to those of control subjects and observed that the patient demonstrated a decrease in perfusion in the frontoparietal associative cortices during the sleepwalking episode compared to wakefulness in controls. This hypoperfusion was interpreted as reflecting a lack of self-related awareness and the inability to recall the events of the sleepwalking episode. In contrast, the hyperperfusion of the posterior cingulate and cerebellum was thought to reflect persistent arousal patterns, which is in line with the hypothesis of a dissociated state. Further studies should confirm these findings using a larger sample size.
34.4.2 REM Sleep Behavior Disorder
Within REM parasomnias, RBD is accompanied by a loss of skeletal muscle atonia usually present during REM sleep and involves complex motor activity occurring specifically in association with dream mentation. The disorder is characterized by unpleasant dreams and dream enactment, which could be disturbing to the patient or the bed partner (AASM 2005). RBD can exist with or without a medical condition, respectively known as secondary RBD or idiopathic RBD. Parkinson’s disease, dementia with Lewy bodies (DLB), and multiple system atrophy tend to develop in patients with RBD several years later (Postuma et al. 2009). SPECT and PET have played a significant role in highlighting the brain regions involved in RBD pathophysiology and clinical evolution (Fig. 34.2).
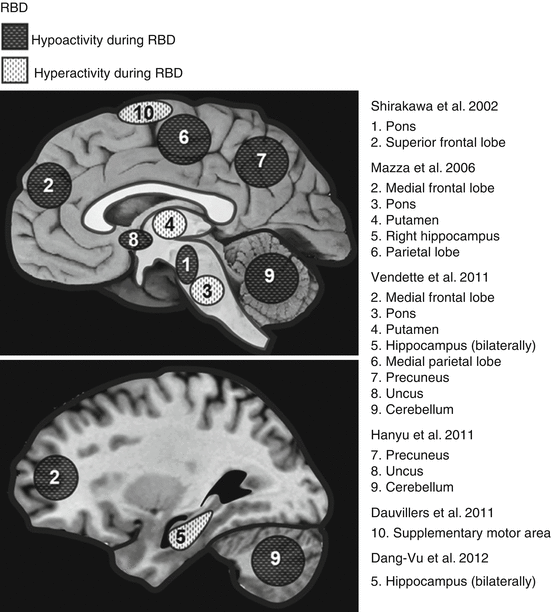
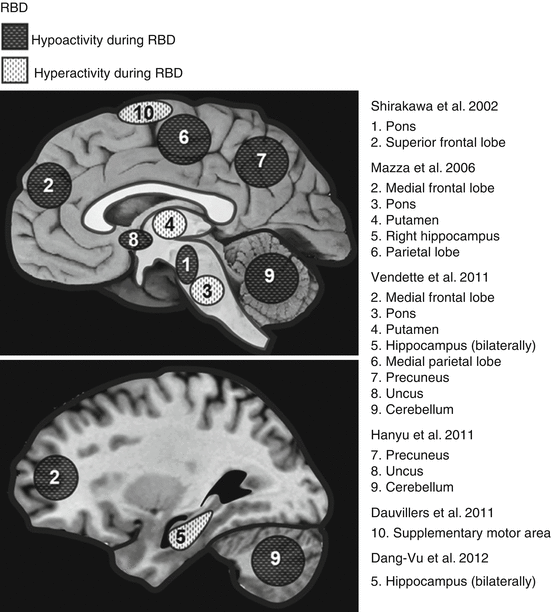
Fig. 34.2
Brain regions showing hyperperfusion or hypoperfusion in rCBF during wakefulness (except for Dauvilliers et al. (2011), which was conducted during a RBD episode in REM sleep) in RBD patients. Many of these changes in rCBF mirror those observed in the early stages of Parkinson’s disease (Adapted from Desseilles et al. (2008))
A study performed by Shirakawa et al. (2002) compared 20 male idiopathic RBD patients to 7 healthy male subjects using N-isopropyl-p-123I-iodoamphetamine (123I-IMP) SPECT. Compared to the control group, a statistically significant decrease of rCBF was found in the right and left upper portion of the frontal lobe and in the pons. The scans were performed at night, although it was not clear which state of vigilance they were experiencing.
Mazza et al. (2006) conducted a study using 99mTc-ECD SPECT, which included eight idiopathic RBD patients and nine healthy control subjects. In contrast to Shirakawa et al. (2002), significant hyperperfusions were found in the pons, as well as in the putamen and the right hippocampus. Interestingly, increased rCBF is also present in the latter two regions during the early stages of Parkinson’s disease (Imon et al. 1999). In addition, decreased perfusion was found in the frontal lobe, particularly in the motor cortices and in the temporo-parietal cortices. A larger study of 20 idiopathic RBD patients and 20 control subjects exhibited similar results (Vendette et al. 2011). Once again using 99mTc-ECD SPECT, hyperperfusion was displayed in pons, putamen, and bilaterally in the hippocampus and hypoperfusion in frontal and medial parietal areas.
Hanyu et al. (2011) monitored rCBF using 123I-IMP SPECT in 24 patients with IRBD. In contrast with previous studies, they did not find significant differences between patients and controls in the brainstem and frontal areas. Results did however display hypoperfusion in RBD patients, in the precuneus, cerebellum, and uncus, regions also identified by Vendette and colleagues (2011).
Two studies led by Caselli et al. (2006) and Fujishiro et al. (2010) assessed CMRglu with 18F-FDG PET in subjects with dream-enactment behavior. These subjects displayed decreased CMRglu in multiple cortical areas, such as occipital, frontal, parietal, temporal, and cingulate. No polysomnographic recording was performed to confirm a diagnosis of RBD; rather, patients were selected based on questionnaires and interviews only, hence diminishing the validity of the study.
SPECT with 99mTc-ECD was recently used to predict the onset of PD and DLB in 20 idiopathic RBD patients (Dang-Vu et al. 2012). The average follow-up of 3 years revealed that PD or DLB emerged in ten of the patients; interestingly, these ten patients showed an increase in hippocampal rCBF at baseline. It can thus be proposed that the progression of idiopathic RBD into PD or DLB can be predicted via abnormal perfusion in the hippocampus.
While the studies above described functional neuroimaging acquired in RBD patients mainly during wakefulness, only one study reported brain activations associated with RBD behavioral manifestations. This study was conducted on a single patient, with multiple system atrophy and RBD, and compared to two healthy control subjects (Dauvilliers et al. 2011). After injecting 99mTc-ECD during a RBD episode, compared to wakefulness, the patient showed increased perfusion in the supplementary motor area, suggesting this area’s involvement in the onset of dream-enactment behaviors. The effect was not present in controls when contrasting REM sleep versus wakefulness. No SPECT data was obtained during REM sleep outside the behavioral episode in RBD patients.
Due to the relationship between RBD and PD, multiple system atrophy, and other conditions associated with DA dysfunction (Gagnon et al. 2009), there have been numerous SPECT and PET ligand studies in the last decade analyzing the nigrostriatal DA system in RBD patients. A group performed two SPECT studies with 123I-IPT demonstrating a decrease in DAT at the presynaptic site of the striatum in idiopathic RBD patients compared to age- and sex-matched controls (Eisensehr et al. 2000, 2003a). Additionally, these two studies also included an assessment of postsynaptic D2-receptor binding using 123I-IBZM SPECT and found no significant change in RBD compared to controls and PD. This suggests that DA dysfunction in the striatum is restricted to the presynaptic level in RBD patients, in line with a loss of DA midbrain neurons, and similarly to findings in PD (Tatsch et al. 1997).
The same conclusion was reached in a PET study using 11C-dihydrotetrabenazine (11C-DTBZ) in a study comparing 6 idiopathic RBD patients to 19 controls (Albin et al. 2000). In agreement with the studies conducted by Eisensehr and colleagues (2000, 2003a), the density of striatal DA was measured, and a decrease in presynaptic binding was found, most prominently in the posterior putamen.
Similarly, another PET study was performed using 11C-DTBZ to measure presynaptic striatal binding. The 13 patients who had RBD and probable multiple system atrophy showed a decrease in binding, which was negatively correlated with the severity of REM atonia (Gilman et al. 2003). These results suggest that a presynaptic DA deficit might contribute to the frequent occurrence of RBD in patients with multiple system atrophy.
Four studies examined DAT in RBD patients using SPECT with 123I-2β-carbomethoxy-3β-(4-iodophenyl)-N-(3-fluoropropyl)-nortropane (123I-FP-CIT). Two studies in particular concluded that an insignificant number of RBD patients demonstrated a decrease of striatal DAT (Stiasny-Kolster et al. 2005; Unger et al. 2008). Another report compared 14 idiopathic RBD patients, 14 early-stage Parkinson’s disease, and 12 controls (Kim et al. 2010). Further, confirming the studies performed by Eisensehr and colleagues (2000, 2003a), the RBD patients showed lower binding in the striatum compared to control subjects, more specifically in the putamen. This binding was however higher compared to Parkinson’s disease patients, suggesting a progressive DA impairment from RBD to Parkinson’s disease. In a more recent 123I-FP-CIT SPECT study, 43 idiopathic RBD and 18 controls were examined longitudinally for striatal DAT (Iranzo et al. 2010). It was found that there was reduced binding in 40 % of the RBD patients. This study included a follow-up demonstrating that a neurodegenerative disorder developed in eight of the IRBD patients within 2.5 years after the imaging took place. Interestingly, 6 of these 8 patients had reduced DAT at baseline, highlighting the significance of lowered DAT in the prediction of disease evolution.
A case study involving a 73-year-old man used 11C-CFT to assess changes of nigrostriatal presynaptic DA 1 and 3.5 years after the onset of RBD (Miyamoto et al. 2010). Compared to controls, the first year’s results displayed only a minor decrease in the posterior putamen, yet after 3.5 years there was a more pronounced decrease of 4–6 % per year. Similarly, a recent 3-year study used 123I-FP-CIT SPECT on 20 IRBD patients (Iranzo et al. 2011). Complementary to the case report, there was a reduction in binding over time (compared to controls) in all striatal regions with the exception of the right caudate nucleus, further demonstrating a progressive nigrostriatal dopaminergic dysfunction.
34.4.3 Summary
Several SPECT and PET neuroimaging studies are available for RBD. However, to date, only one study has been devoted to sleepwalking. Sleepwalking demonstrates brain patterns reminiscent of both SWS and wakefulness states, therefore appearing as a dissociated state. Additional studies are needed to further qualify the role of SWS alterations in somnambulism.
In RBD patients, SPECT and PET have shown that there exists a presynaptic dysfunction of DA nigrostriatal pathways, further indicating that RBD represents the early stages of PD, DLB, and multiple system atrophy. Moreover, the risk of progression from RBD to other neurodegenerative disorders can be estimated using SPECT. Hypoperfusions found in the pons agree with human studies involving pontine lesions in RBD pathophysiology (Culebras and Moore 1989; Gomez-Choco et al. 2007; Kimura et al. 2000; Limousin et al. 2009; Plazzi and Montagna 2002; Provini et al. 2004; Schenck and Mahowald 2002; Tippmann-Peikert et al. 2006; Xi and Luning 2009; Zambelis et al. 2002). The role of structures such as the hippocampus and cognitive aspects of RBD should be further investigated. Finally, brain activity patterns during behavioral episodes and during sleep should be examined to shed further light on the pathophysiology of RBD.
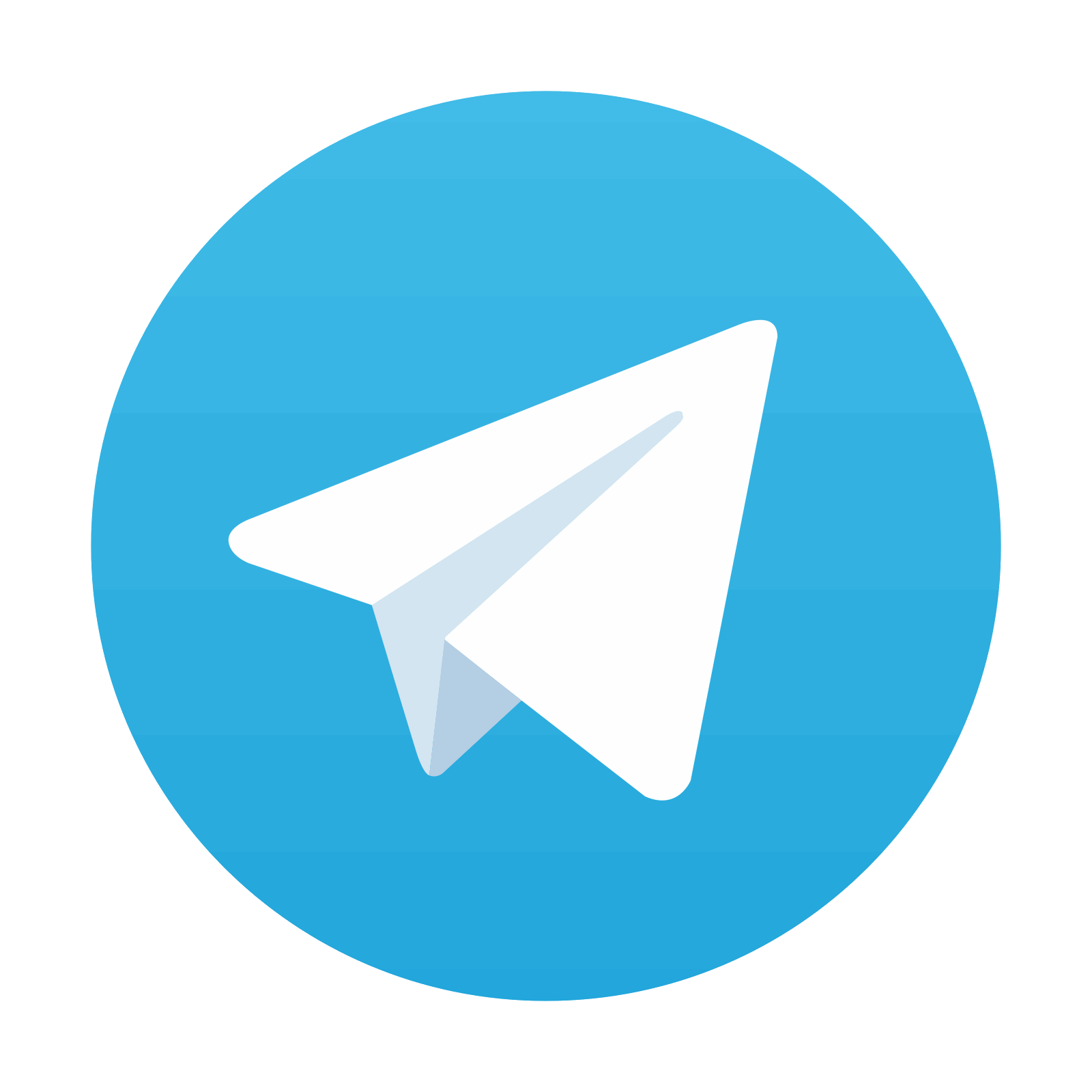
Stay updated, free articles. Join our Telegram channel

Full access? Get Clinical Tree
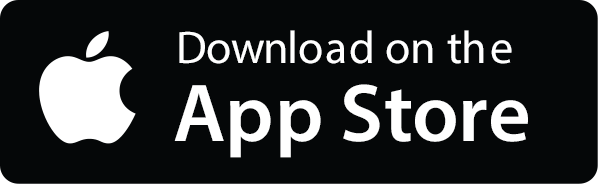
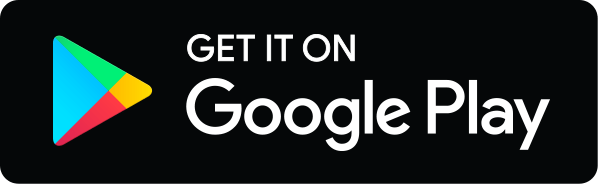