html xmlns=”http://www.w3.org/1999/xhtml” xmlns:mml=”http://www.w3.org/1998/Math/MathML” xmlns:epub=”http://www.idpf.org/2007/ops”>
Introduction
Currently there are no US Food and Drug Administration (FDA)-approved therapies for frontotemporal dementia (FTD). Some medications are used off-label for symptomatic management; however, there is minimal evidence for efficacy to support their use. Such therapies rely on modulation of neurotransmitter levels and do not target the underlying pathophysiology of FTD. The limited number of cases of FTD, heterogeneous presentations of FTD, and diverse pathology has historically made it difficult to conduct adequately powered placebo-controlled clinical trials. Most studies of medications have grouped disparate clinical syndromes together and did not attempt to stratify by underlying pathology.
Fortunately there have been remarkable advancements in the understanding of frontotemporal lobar degeneration (FTLD) pathophysiology, genetics, and neuropathology recently. New biomarkers, and new cellular and animal models have been developed. Advances in treatment development for Alzheimer’s disease (AD), which shares some pathogenic molecules with FTD, particularly tau, are likely to inform FTD drug developments, and potentially provide disease-modifying therapies in FTD. Finally, new molecular imaging techniques including tau positron emission tomography (PET) scanning will greatly improve the feasibility of carrying out trials in well-defined FTD syndromes.
This chapter will review the evidence for currently available pharmacologic therapies for FTD and its related disorders. In addition it will discuss potential therapy targets for disease-modifying treatment, pharmacologic therapies in various stages of development, as well as models that may accelerate therapy discovery. The chapter will conclude with suggestions for collaboration with industry partners, clinical trials design, and regulatory considerations.
Neurotransmitter-based symptomatic treatments
Most current medications for FTD were developed for use in psychiatric disorders or AD, and are not indicated for FTD treatment, but are used in desperation to try to manage the behavioral symptoms of FTD. These agents work by modulating the levels or downstream effects of various central nervous system (CNS) neurotransmitters, strategies that are effective in Parkinson’s disease and Alzheimer’s disease. Previous research has implicated alterations in cholinergic, serotonergic, dopaminergic, noradrenergic, and glutamatergic systems in FTD [1]. However, there have been few high-quality clinical studies of therapeutic agents that target these alterations. Most of the available evidence is limited to small case series or open label clinical trials. In addition, there are examples of initially promising therapies for FTD identified in open label studies that have not stood up to the rigors of randomized, double-blind, placebo-controlled trials, underscoring the need to interpret the vast majority of previous clinical therapeutic research in FTD with extreme caution. With these caveats in mind, we review the previous literature on symptomatic therapies for FTD below.
Antidepressants
The serotonergic system has been suggested to mediate behaviors such as impulsivity, aggression, and disinhibition [2]. These behaviors are also commonly seen in behavioral variant (bvFTD) patients. Neuropathologic analyses have shown FTD patients to have a dysfunctional serotonergic system, with reduction of 5-HT1 and 5-HT2A serotonin receptors in orbital frontal, cingulate, medial frontal, and temporal regions [3, 4]. Another study also demonstrated a 40% loss of neurons in the serotonergic raphe nuclei [5]. Selective serotonin reuptake inhibitors (SSRIs) are attractive agents for use in FTD patients as they increase serotonin levels, and have historically been successful in treating clinical symptoms in psychiatric patients that resemble some of the problematic FTD behaviors. As shown in Table 18.1, SSRIs such as citalopram, fluoxetine, paroxetine, sertraline, fluvoxamine, and clomipramine have all been described to be beneficial for managing FTD behaviors in small open label clinical trials or case series [6–14]. Unfortunately, as is often the case, efficacy in small preliminary studies has not been replicated in randomized placebo-controlled clinical trials. Paroxetine showed significant behavioral improvements in two open label trials but failed to demonstrate improvements in a subsequent six-week, double-blind, placebo-controlled crossover trial [15]. The only medication with serotonergic activity with proven efficacy in a small randomized, double-blind, placebo-controlled trial was trazodone, which demonstrated improvements in Neuropsychiatric Inventory (NPI) scores 12 weeks after treatment [16]. Most clinicians believe that antidepressants may help to manage various behavioral symptoms in FTD patients, but with most evidence coming from open label trials or case series, the evidence to support this opinion is mainly anecdotal.
Antidepressants in FTD
Drug | Study design | n | Dx | Citation | End point |
---|---|---|---|---|---|
Citalopram | 6 week open label, no placebo | 15 | FTD | 6 | Improved NPI, FBI |
Fluoxetine | 3 month open label, no placebo | 5 | FTD | 7 | Improved disinhibition, depressive symptoms, carbohydrate craving, and compulsions |
Paroxetine | Case series | 11 | FTD/nfvPPA/svPPA | 8 | Improved repetitive behavior |
14 month open label, randomized, no placebo, compared to piracetam | 16 | FTD | 9 | Improved NPI, RSS | |
6 week, double-blind, placebo-controlled, crossover | 10 | FTD | 15 | No significant differences in NPI, CBI. Decrease in some cognitive tasks | |
Sertraline | 3 month open label, no placebo (fluoxetine and paroxetine also used) | 5 | FTD | 7 | Improved disinhibition, depressive symptoms, carbohydrate craving, and compulsions |
6 month open label, no placebo, compared with AD patients | 18 | FTD | 10 | Decrease in stereotypic movements | |
Case report | 1 | FTD–ALS | 11 | Improved in appropriate sexual behavior | |
2 and 4 weeks open label | 4 | svPPA | 12 | Improved NPI | |
Fluvoxamine | 12 week open label, no placebo | 16 | FTD, svPPA | 13 | Improved NPI |
Trazodone | 12 week randomized, placebo- controlled, double-blind crossover | 26 | FTD | 16 | Improved NPI |
Clomipramine | Case series | 3 | bvFTD | 14 | Improved behavior |
NPI = Neuropsychiatric Inventory, FBI = Frontal Behavioral Inventory, nfvPPA = non-fluent variant primary progressive aphasia, svPPA = semantic variant primary progressive aphasia, RSS = Relative Stress Scale, CBI = Cambridge Behavioural Inventory, ALS = amyotrophic lateral sclerosis.
Acetylcholinesterase inhibitors
Acetylcholinesterase inhibitors have long been a mainstay of AD symptomatic treatment, based on the loss of cholinergic neurons from the nucleus basalis of Meynert. Perhaps because of their success in AD, acetylcholinesterase inhibitors have been studied more rigorously than other off-label treatments in FTD (Table 18.2). However, as reviewed by Huey et al. in 2006, in contrast to AD, the cholinergic system in FTD patients is relatively spared from pathology [17]. Clinical trials for the three most commonly used acetylcholinesterase inhibitors have produced disappointing results, although none were large enough or designed to allow for a true efficacy determination. A 12-month open label study of rivastigmine dosed 3–9 mg/day showed some improvements in NPI score but did not prevent cognitive deterioration as measured by the Mini-Mental State Examination(MMSE) [18]. A second study investigated galantamine in 36 bvFTD and primary progressive aphasia (PPA) patients. All patients were treated in an open label fashion for 18 weeks followed by a randomized, double-blind, placebo-controlled withdrawal period for 8 weeks. No significant differences were found in the bvFTD group, while language function remained stable in some of the treated PPA group compared with placebo, who likely had the logopenic form of PPA caused by underlying Alzheimer’s pathology [19]. Donepezil, perhaps the most widely used cholinesterase inhibitor, was studied in a 6-month, open label study in 24 bvFTD patients. The donepezil-treated group had greater worsening on the FTD inventory, and four of the treated patients had worsening behavior. Discontinuation of donepezil led to an abatement of behavioral symptoms, which was replicated in a more recent discontinuation trial of donepezil in FTD patients conducted in Japan [20, 21]. Currently, the evidence suggests cholinesterase inhibitors are not effective treatments in FTD patients and may exacerbate behavioral symptoms. Routine use is not recommended.
Acetylcholinesterase inhibitors in FTD
Drug | Study design | N | Dx | Citation | End point |
---|---|---|---|---|---|
Donepezil | 6 month, non-randomized open label | 24 | bvFTD | 20 | Worsening behavioral symptoms |
2 week discontinuation study | 23 | FTD | 21 | Improved NPI and Zarit Burden Interview | |
Galantamine | 18 week open label followed by 8 week randomized, double-blinded | 36 | bvFTD, PPA | 19 | No signficant changes seen in behavior, non-significant stabilization of language scores for PPA group |
Rivastigmine | 12 month open label, compared with control group | 20 | FTD | 18 | Improved NPI and other behavioral scores, no improvements in cognition |
Antipsychotics and dopaminergic therapies
The dopaminergic system is a potential therapeutic target for FTD but poses an interesting treatment paradox. Patients with frontotemporal dementia with parkinsonism-17 (FTDP-17), a rare form of autosomal dominant FTD usually due to MAPT or GRN mutations, with symptoms of parkinsonism and FTD, have evidence of dopaminergic system dysfunction. Imaging studies such as PET and single-photon emission computed tomography (SPECT) have shown decreased presynaptic dopamine transporter binding in the striatum of FTD patients and decreased postsynaptic dopamine receptor binding in FTDP-17 patients respectively [22, 23]. Dopamine reduction in the striatum of FTD patients has also been demonstrated [24]. However, behavioral symptoms in FTD can be similar to symptoms in schizophrenia, especially in agitation and disinhibition, which are thought to be due to increased dopaminergic activity. Although it should be noted that most dopaminergic abnormalities in schizophrenia are thought to be attributed to the cortical as opposed to striatal dopaminergic system, antipsychotics with dopamine receptor antagonistic properties have successfully treated such symptoms in psychiatric patients. Thus despite the contrary evidence described above, both dopamine blockade and augmentation therapies have been evaluated in FTD patients, as listed in Tables 18.3 and 18.4.
Drug | Study design | N | Dx | Citation | End point |
---|---|---|---|---|---|
Quetiapine | Case series | 3 | FTD/nfvPPA/svPPA | 8 | Improvement in agitation |
Crossover with dextroamphetamine, double-blind | 8 | bvFTD | 29 | No significant change in NPI | |
Risperidone | Case report | 1 | Pick’s | 25 | Stabilization of cognitive function |
Case series | 3 | FTD/nfvPPA/svPPA | 8 | 1/3 with symptoms of agitation responded | |
Aripiprazole | Case report | 1 | FTD | 26 | Improvement in clinical symptoms |
Case report | 1 | FTD | 27 | Improvement in sexually inappropriate behavior | |
Olanzapine | 24 month open label | 17 | FTD | 28 | Improved NPI, RSS |
Drug | Study design | N | Dx | Citation | End point |
---|---|---|---|---|---|
Selegiline | Case series | 3 | FTD | 31 | Improved behavior |
Bromocriptine | 14 weeks, double-blind, placebo- controlled, crossover | 6 | PPA | 33 | No overall improvements |
Methylphenidate | 2 weeks, double-blind, placebo- controlled, crossover | 8 | FTD | 32 | Decreased risk-taking behavior |
Dextroamphetamine | 8 weeks, randomized, crossover with quetiapine, double-blind | 8 | bvFTD | 29 | Improved NPI |
Evidence for the use of antipsychotics in FTD patients comes mainly from anecdotal case reports and series. Risperidone, quetiapine, and aripiprazole have been suggested to mitigate behavioral disturbances [8, 25–27]. A 24-month open label trial evaluated olanzapine in five groups of patients with various dementia diagnoses. Of the 17 patients with FTD, improvements in delusions, NPI scores, and caregiver stress were observed. Other groups with diagnoses of Lewy body dementia, vascular dementia, and Parkinson’s disease dementia also experienced significant behavioral improvements [28]. In a small, double-blind, crossover study with dextroamphetamine and quetiapine involving eight FTD patients with behavioral symptoms, no difference in NPI was shown between baseline and quetiapine treatment [29]. Currently, the evidence for antipsychotic use in FTD is limited, and use carries the risk of extrapyramidal side effects, to which FTD patients are particularly vulnerable [30]. In addition, the FDA recently determined the use of atypical antipsychotics for behavioral symptoms treatment of dementia to be associated with higher mortality than placebo, related to cardiac or infectious events, and issued a black box warning for all antipsychotics.
With the dopamine deficiencies described above, medications that augment dopamine levels or signal transduction have also been tested in FTD patients. A small series of three FTD patients evaluated the monoamine oxidase (MAO)-B inhibitor selegiline and reported improvements in NPI scores [31]. Similar results were shown in a small, randomized, double-blind crossover study in eight FTD patients using dextroamphetamine along with quetiapine, which is thought to augment dopamine levels by preventing reuptake and inducing release of dopamine [29]. Another double-blind, placebo-controlled, crossover study using methylphenidate, a dopamine and norepinephrine reuptake inhibitor, in eight FTD patients demonstrated improvements in risk-taking behaviour [32]. Six PPA patients of unspecified variant were enrolled in a double-blind, placebo-controlled, crossover trial using bromocriptine, a dopamine agonist. Mild slowing of language deterioration was detected but bromocriptine did not appear to significantly alter disease course [33]. In summary, while a variety of dopamine-modulating therapeutics have shown potentially promising preliminary results in FTD, rigorous placebo-controlled studies should be performed to determine their true safety and efficacy. Safety is a particular concern for these drugs in light of their potential adverse effects such as increased behavioral disturbances, risk-taking behavior, hallucinations; symptoms that have been observed in patients receiving dopamine replacement therapy for Parkinson’s disease.
Anti-epileptics
Anti-epileptic (anticonvulsant) agents have been used as mood stabilizers for manic behaviors, but none have been studied rigorously in the treatment of FTD. Currently there are case reports and case series, as shown in Table 18.5, that report experiences with such medications in FTD. The choice of anti-epileptics has typically been those with mood stabilizing effects such as carbamazepine and valproic acid, with reported improvements in behavior. [8, 34] Topiramate is an anti-epileptic with a migraine treatment indication and mood stabilizing effects and has been incorporated into some weight loss drugs based on a known propensity to cause anorexia. Interestingly, in several case reports it has been shown to reduce hyperorality in FTD patients but no placebo-controlled trials have been done [35–38].
Drug | Study design | N | Dx | Citation | End point |
---|---|---|---|---|---|
Carbamazepine | Case report | 1 | FTD | 34 | Reduced inappropriate sexual behavior |
Topiramate | Case report | 1 | FTD | 35 | Reduced alcohol abuse |
Case report | 1 | FTD | 36 | Improvement in abnormal eating | |
Case report | 1 | bvFTD | 37 | Improvement in abnormal eating | |
Case series | 3 | FTD | 38 | Improvement in compulsive eating | |
Valproic acid | Case series | 3 | FTD/nfvPPA/svPPA | 8 | 3/3 showed improvement in agitation |
Memantine
Memantine is a (N-methyl D-asparate) NMDA receptor antagonist that is indicated for the treatment of moderate to severe AD. Previously, a randomized, double-blind, placebo-controlled trial of 252 patients with moderate to severe AD demonstrated improvements in activities of daily living, cognition, and clinician impression on memantine compared with placebo [39]. Subsequently a randomized, double-blind, placebo-controlled clinical trial of 404 moderate to severe patients already on donepezil showed that addition of memantine resulted in improvements in cognition, activities of daily living, global outcome, and behavior symptoms [40]. Although the underlying pathology and neurotransmitter changes are different in AD compared with FTD, the NMDA receptor is thought to play a role in neurologic dysfunction due to range of different neurologic disorders. Excitotoxicity via overactivation of NMDA receptors by glutamate may be a final common pathway responsible for neuronal death. Whereas one small open label study demonstrated no effects of memantine in bvFTD [41], a small case series and a phase IV open label memantine study suggested improvements in behavioral symptoms as measured by the NPI [42–44]. The efficacy of memantine was further tested in two rigorous randomized, placebo-controlled clinical trials, one 52 weeks and one 26 weeks (Table 18.6) [45, 46]. Although both did not enroll the number of patients originally planned, both failed to demonstrate significant benefits on the NPI or Clinical Global Impression of Change. In addition, memantine treatment was associated with worse performance on a number of cognitive tests [46]. These studies provide fairly strong evidence that memantine is not an effective treatment for FTD. Experience with memantine in FTD underscores the limitations of attempting to extrapolate the efficacy of drugs for FTD based on placebo-controlled trials in AD and other disorders, even when there is a strong scientific rationale for use of the drug in FTD.
NMDA antagonist in FTD
Drug | Study design | n | Dx | Citation | End point |
---|---|---|---|---|---|
Memantine | 3 month case series | 3 | bvFTD | 43 | Improvement in NPI |
6 month open label, uncontrolled | 16 | bvFTD | 41 | No change in NPI or FBI, overall decline in cognition | |
26 week open label | 43 | bvFTD/nfvPPA/svPPA | 44 | Transient improvement in NPI in FTD group, FTD and svPPA declined on cognitive and behavioral, stable on UPDRS. nfvPPA was stable on ADAS-cog, NPI, and TFLS but declined on UPDRS | |
12 month, randomized, double-blind, controlled | 49 | bvFTD | 45 | No signficant changes except FBI was mildly lower in treatment arm | |
26 weeks randomized, parallel group, double-blind, placebo-controlled | 81 | bvFTD/svPPA | 46 | No effect on NPI, CGIC |
UPDRS = Unified Parkinson’s Disease Rating Scale, ADAS-cog = Alzheimer’s Disease Assessment Scale-Cognitive, TFLS = Texas Functional Living Scale, CGIC = Clinical Global Impression of Change.
Parkinsonism treatment
A substantial portion of FTD patients also present with parkinsonism. FTDP-17 refers to autosomal dominantly inherited FTD associated with mutations in the tau (MAPT) or progranulin (GRN) genes and also frequently associated with parkinsonism. Moreover the disorders such as progressive supranuclear palsy (PSP) and corticobasal degeneration (CBD) which are defined by atypical parkinsonism, typically with substantial rigidity but infrequent tremor or levodopa response, overlap both clinically and neuropathologically with FTD. FTD patients with parkinsonism typically do not respond to dopaminergic therapy such as levodopa/carbidopa, although a few cases of potential benefit have been reported [8]. When significant parkinsomism arises in the setting of FTD, dopaminergic therapy can be attempted, although there is little evidence to suggest a benefit. Moreover, side effects such as nausea, hypotension, psychosis, and livedo reticularis are major issues that often limit the ability to escalate the dose of dopaminergic agents.
Potential disease-modifying treatments
In the past decade, knowledge has rapidly advanced regarding the pathology, genetics, and pathophysi-ology of the various forms of FTLD (see Chapters 13, 14, and 15). In parallel, these insights have been employed to attempt to develop strategies for interventions that would modify the underlying disease processes of FTLD.
Targets
Tau
Tau is a microtubule-associated protein localized to neuronal axons that regulates the stability of microtubules by promoting tubulin polymerization. Tau-related neuronal dysfunction in neurologic disease may occur by two non-mutually exclusive mechanisms, either gain of function or loss of function. Tau gain of function occurs when tau aggregates, which may be toxic to neurons and glia [47, 48]. Tau hyperphosphorylation is also seen in disease states, and may promote aggregation of insoluble tau species and also decrease microtubule binding, leading to loss of tau function [49]. Aggregates of hyperphosphorylated tau are found in AD and approximately half of all FTLD disorders, including Pick’s disease, PSP, CBD, argyrophilic grain disease, neurofibrillary tangle dementia, and FTDP-17. Reducing hyperphosphorylated tau aggregrates and other potentially toxic tau species, altering post-translational modification of tau, such as reducing phosphorylation, or stabilization of microtubules to make up for loss of tau function may all be viable strategies for FTLD-tau therapy. The strong genetic association of tau, via mutations in microtubule-associated protein tau (MAPT), with FTLD provides a rationale for interventions that target tau in FTLD cases with underlying tau pathology. The strong association of the most common PSP clinical syndrome (Richardson’s) with the presence of tau protein aggregates on neuropathologic examination creates a strong rationale for investigating the effects of tau-directed therapies in these patients as well.
Because many were originally intended for use in AD, tau-directed therapies are more advanced than other potential disease-modifying therapies for FTD. An underlying assumption is that tau pathology in AD is sufficiently similar to tau in FTD that the same therapies should be efficacious for both disorders. Therapeutic strategies with tau fall under four general categories: inhibition of aggregation, inhibition of phosphorylation, reduction of tau levels, and microtubule stabilization. The strongest evidence for efficacy from preclinical models of tau-mediated neurodegeneration exists for agents that reduce tau protein levels. Interestingly, a number of transgenic mouse lines that lack tau expression have been found to survive to adulthood with none to few measurable deficits.
Tau gain-of-function therapies
Methylene blue (reformulated as LMTx), an inhibitor of tau and possibly transactive response DNA-binding protein 43 (TDP-43) aggregation, completed a phase II clinical trial in AD in 2009, and is now being investigated in two phase III clinical trials for AD and bvFTD. Pathologic tau species are frequently hyperphosphorylated. Protein kinase inhibition may prevent phosphorylation, and glycogen synthase kinase 3 (GSK3) has been an early target of therapies meant to block tau phosphorylation. Clinical trials using lithium chloride for PSP and CBD and tideglusib for PSP, both thought to be GSK3 inhibitors, were not successful because of toxicity (lithium) and lack of efficacy (tideglusib). Lithium chloride used in AD patients did not change cerebrospinal fluid (CSF) phosphorylated tau levels or GSK3 activity in serum [50]. Preliminary reports also suggest that the specific GSK3b inhibitor, tideglusib, also did not demonstrate any effects on cognitive end points in a phase IIb AD trial or a phase II trial in PSP [51, 52]. Modulating phosphatase activity to remove phosphate groups or more specific kinase inhibitors remain a possible intervention to ameliorate tau pathology and remain an active area of study. In addition, modulating other post-translational modifications of tau such as acetylation and glycation may be other promising therapeutic strategies [53]. Decreasing total tau protein levels via antisense oligonucleotide-mediated reduction of mRNA levels is actively being investigated in a number of academic laboratories and pharmaceutical companies [54].
The most advanced strategies for reducing endogenous tau levels involve immunologic approaches targeting a variety of different tau epitopes. Recent studies demonstrating that pathogenic tau species are transmitted trans-synaptically provide support for reducing tau levels in the extracellular space. Immunotherapeutic approaches to tau can be characterized as active or passive immunization. Active immunization involves introduction of foreign antigen into the subject, inducing a T-cell response and antibody generation. Active immunization raises safety concerns as previous amyloid active immunotherapy trials were associated with encephalitis. The use of smaller tau peptides may decrease the risk of inflammation while retaining the ability to generate antibodies that clear tau pathology in the cortex [55]. Immunized transgenic mouse models with human tangle pathology have been shown to perform better on sensorimotor tests with decreased cognitive impairment [56, 57]. At least one anti-phosphorylated tau vaccine is currently in clinical development [58].
Passive immunization has the advantage of potentially fewer autoimmune side effects, and several approaches have been described in tau transgenic mice. Antibodies directed against various tau epitopes have been administered to mouse models prior to the onset of pathology, demonstrating reduction in tau tangles, pathologic tau, and improved motor tasks [59, 60]. Recently, an anti-tau monoclonal antibody that targets a pathologic form of tau that is able to seed other pathologic conformations was shown to block seeding activity and improve cognitive deficits in tau transgenic mice [61]. As this antibody is not thought to enter neurons, its mechanism of activity likely involves peripheral clearance, promotion of microglia tau uptake, or blocking trans-synaptic spread of tau species with seeding activity. In short, proof of concept has been demonstrated in animal models using both active and passive immunization approaches, with improvements in clearance of tau as well as memory and motor tasks, but human studies are still needed. It remains to be seen whether certain tau conformations are better targets for immune clearance and whether different tau conformations are associated with different tauopathies.
Tau loss-of-function therapies
Loss of tau binding to microtubules is thought to impair their function in transporting cellular constituents via a loss in MT stability [62]. Stabilization of microtubules has been proposed as a way to make up for loss of tau function. Taxanes, a class of cancer drugs derived from taxol that stabilize tubulin and a related class of compounds called epothilones, with fewer toxic side effects and better blood–brain barrier penetration have been of interest. A recent study suggests paclitaxel may help restore synaptic function in mutant human tau neurons [63]. The leading compound in this class, epothilone D, was found in transgenic tau mouse models to improve axonal microtubule density and decreased axonal dystrophy. In addition, spatial learning deficits improved in treated mice, at a dose 1/30th to 1/10th of the dose utilized in human cancer trials [64, 65]. Epothilone D was briefly investigated in a phase I clinical trial for AD, but was abandoned for further development. Recently a peptide derived from the growth factor activity-dependent neurotrophic protein called davunetide, which may also promote microtubule stability, failed to show efficacy in a pivotal clinical trial in PSP patients (unpublished data). Despite these disappointing results, microtubule-stabilizing agents continue to be explored as potential therapies, and various similar compounds are in phase I or preclinical testing as of this writing. TPI-287, a taxane-based microtubule-stabilizing agent has entered phase I clinical trial for Alzheimer’s disease [66].
TDP-43
Insoluble deposits of TDP-43 in neurons and glia are found in approximately half of FTLD cases, termed FTLD-TDP [67]. Of the FTD clinical syndromes, FTLD-TDP is strongly associated with FTD-ALS (amyotrophic lateral sclerosis) and svPPA. Two autosomal dominant forms of FTLD, FTLD due to progranulin (GRN) and chromosome 9 open reading frame 72 (C9orf72) mutations, display FTLD-TDP pathology, although TDP-43’s role in the pathogenesis of these syndromes is unclear. Mutations in the TDP-43 gene (TARBP) itself are generally associated with an ALS phenotype; rarely these are also associated with FTD. In FTLD and ALS, aggregates of TDP-43 are hyperphosphorylated, ubiquitinated, and cleaved in the C-terminal fragments. TDP-43 is a RNA-binding protein involved in transcription, splicing, and transport of a variety of heterogeneous RNA targets, and is essential for neural development and axon guidance. In addition, TDP-43 is able to autoregulate its expression level through a negative-feedback loop [68]. Whether TDP-43 aggregates or loss of TDP-43 function leads to neurodegeneration is under investigation. A challenge has been that numerous mouse transgenic models overexpressing mutant TDP-43 have failed to demonstrate TDP-43 aggregates despite ALS-like phenotype development [69, 70]. Yet disease progression was halted when the transgene was turned off, suggesting function may be more relevant to pathogenesis [71]. Eventual elucidation of TDP-43 function may lead to targeted therapies directed at this pathologic finding. Of note TDP-43, C9ORF72, and the fused in sarcoma protein (FUS), which is also associated with a rare but severe form of FTD (and ALS), are all involved in RNA processing, and thus general strategies to modulate RNA processing and transport may be promising therapeutic approaches to FTLD-TDP. Development of human biomarkers that can quantify abnormal TDP-43 or its associated proteins may also help FTLD-TDP patients by allowing accurate therapeutic intervention. Recently, the ratio of phosphorylated tau to total tau was shown to help identify human cases of FTLD-TDP [72]. It is hoped that tau PET imaging may also be able to differentiate FTLD-TDP cases during life; however, this has yet to be formally demonstrated [73].
Progranulin
Mutations in the progranulin gene (GRN) discovered in 2006 account for up to 5–10% of FTD cases with European ancestry [74]. GRN mutations result in haploinsufficiency of GRN mRNA expression, leading to a readily measurable decrease in serum and CSF levels of progranulin (PGRN) protein that may serve as a useful biomarker not only for diagnosis but also treatment-response therapies in FTLD patients [75–77]. Endogenous molecules such as transmembrane proteins, microRNAs, and allelic variants of GRN that affect PGRN levels are suggested to affect the risk of developing FTLD-TDP [78]. In the CNS, PGRN is produced by both neurons and glia, and has neurotrophic and synaptic effects, as well as regulating the function of microglia. The physiologic role of PGRN in the CNS is an area of continued investigation, but in the periphery PGRN serves as a neurotrophic factor, and plays a role in development, wound repair, tumorigenesis, and inflammation. PGRN has a potent role in regulating systemic inflammation, where it appears to antagonize the pro-inflammatory effects of tumor necrosis factor-α (TNF-α) [79]. FTLD-TDP patients have elevated peripheral TNF-α levels, suggesting that CNS immune dysregulation may play a role in the development of FTLD [80]. Moreover, anti-PGRN antibodies are sometimes found in patients with other forms of systemic autoinflammatory disease [81].
Despite the lack of clear understanding of PGRN function, it is a promising target for FTD treatment. The measurable decreases in human blood and CSF PGRN levels provide strong evidence that decreased PGRN signaling is a cause of FTD. This implies that raising or restoring PGRN levels into the normal range might be an effective therapy for FTLD. Suberoylanilide hydroxamic acid (SAHA), a FDA-approved histone deacetylase inhibitor (HDACi), was shown to be an enhancer of PGRN expression [82]. A recent in vitro study using small molecules to decrease sortilin (SORT1) function, a neuronal receptor for PGRN endocytosis, demonstrated elevated PGRN levels in human cells [83]. Alkalizing drugs such as chloroquine, bepridil, and amiodarone that affect endosomal sorting similarly stimulate PGRN production, through a post-translational mechanism [84]. However, a recent pilot phase II study using amiodarone in five FTD patients with GRN mutation failed to demonstrate elevation of peripheral GRN levels or a change in disease course when retrospectively compared with 13 FTD patients who were followed in previous years. A caveat of this study is the lack of dose-ranging studies, and final utilized dose was based on cardiologic settings [85]. A phase I clinical trial of the CNS-penetrant calcium channel blocker nimodipine is under way to determine the maximum tolerated dose and its effects on serum and CSF PGRN levels [86]. In addition, PGRN’s role in inflammation may also provide novel intervention strategies as described below.
Immunogenicity
There has long been a suspicion that there is an inflammatory contribution to neurodegenerative pathogenesis. Elevations in CSF cytokines, notably TNF-α, have previously been demonstrated in FTD but without clear differentiation which pathologic subtypes were associated [87]. PGRN mutation with TDP-43 pathology has been of particular interest as it has been demonstrated that PGRN knockout mice develop inflammatory arthritis and PGRN demonstrated antagonistic effects to TNF-α signaling [79]. In addition, in one study, around 40% of rheumatoid arthritis and systemic lupus erythematosus patients had anti-PGRN antibodies that significantly lowered PGRN levels, similar to the haploinsufficiency state of PGRN mutation carriers [81]. A high prevalence of systemic autoimmune conditions and elevated levels of TNF-α in svPPA and GRN mutation carriers compared with Alzheimer’s controls, normal controls, and general population further support the role of inflammation in FTLD-TDP. Specific autoimmune conditions found to be significantly elevated were inflammatory arthritides, such as rheumatoid arthritis, systemic lupus erythematosus, and psoriasis; cutaneous disorders; and gastrointestinal conditions [80].
The findings above suggest there may be an inflammatory role to the pathogenesis of FTD, especially in TDP-43 pathology. Decreased PGRN expression leading to increased TNF-α signaling or primary increased TNF-α signaling may contribute to FTLD-TDP. It may also be that systemic inflammation shares underlying pathogenic mechanisms with those at work in FTLD-TDP. A mounting body of evidence suggests that PGRN also plays a role in the development of AD, and cases of both FTLD-TDP and AD pathology have been described [88]. Modulation of TNF-α levels using FDA-approved anti-TNF-α agents commonly used to treat systemic autoimmune disease, such as infliximab, adalimumab, etc., may be of interest. Engineered PGRN fragments have also been developed and shown to reduce arthritis in mouse models, offering another potential approach to FTLD-PGRN therapy [79]. An exciting possibility is that an efficacious treatment for FTLD-PGRN may predict efficacy in AD and/or systemic autoimmune diseases given the overlap in pathogenic mechanisms.
C9ORF72
FTD and ALS are both neurodegenerative processes. ALS affects both upper and lower motor neurons, presenting with weakness, atrophy, and rapid progression to death; up to 22% of ALS patients meet FTD diagnostic criteria, and 48% manifest cognitive or behavioral abnormalities without meeting the full criteria. Conversely, up to 15% of FTD patients display signs of motor neuron disease or ALS [89]. Both diseases have a 10% prevalence of autosomal dominant pattern as well, suggesting some shared pathophysiology (see Chapter 6).
In 2011, a hexanucleotide repeat expansion in a 5′ non-coding region of the C9orf72 gene was found to be the cause of FTD and ALS in a strongly chromosome 9p-linked family [90–92]. Subsequent analysis revealed this mutation to be the cause of 11.7% of familial FTD and 23% of familial FTD–ALS in one large series. Clinical phenotypes most frequently associated with this mutation are FTD, ALS, and FTD–ALS, but corticobasal syndrome, nfvPPA, and svPPA with and without motor neuron disease have been described. The pathology associated with C9orf72 mutation carriers have been overwhelmingly FTLD-TDP, specifically subtypes A and B, but also with unique p62-positive, TDP-43-negative inclusions, especially in the cerebellum and hippocampus [93].
Although the precise function of C9ORF72 remains unknown, a bioinformatics approach has recently shown that it is structurally similar to differentially expressed in normal and neoplastic cells (DENN) proteins, a class of GDP/GTP exchange factors important for Rab-GTPases, suggesting a role in vesicular trafficking [94]. Expanded repeats may result in untranslated regions causing loss of function. In addition, similar to myotonic dystrophy, expanded RNA transcripts may result in toxic nuclear inclusions that sequester other RNAs or RNA-binding proteins, a class of proteins in which both FUS and TDP-43 belong. The GGGGCC repeat RNA structure also allows for an unconventional means of translation, termed repeat-associated non-ATG (RAN) translation. Recently, antibodies generated against these RAN translation products have identified unique accumulations of poly-(glycine-proline) peptides [95]. Similar polypeptides have also been observed in myotonic dystrophy type 1 and spinal cerebellar ataxia 8. Whether this polypeptide is simply an accumulation of translated products via RAN translation or causes toxic effects remain to be seen, but may serve as a potential sensitive, specific, and early biomarker for C9ORF72 causes of FTD and ALS.
As expanded RNA transcripts may be a possible culprit of neurodegeneration, antisense oligonucleotides (ASO) may serve as a possible therapeutic strategy for FTLD due to C9orf72 hexanucleotide repeat expansions. ASO are synthetic nucleic acids that inactivate the mRNA of a target gene by direct binding or inducing RNase H-mediated cleavage via DNA/RNA heteroduplex. For C9orf72 mutation carriers, this strategy is attractive as it might lower both expanded RNA transcripts and limit RAN translation. Preliminary steps for ASO treatment have been studied in ALS caused by superoxide dismutase (SOD1) and were well tolerated in animal models and a recent phase I clinical trial in human SOD1 mutation carriers [96]. ASO directed against C9orf72 sequences have been shown to reduce C9orf72 RNA expansion-dependent pathology in cultured neurons derived from human C9ORF72-induced pluripotent stem cells (iPS) [97]. Furthermore, injection of ASO directed against C9orf72 sequences into mice did not demonstrate behavioral or neuropathologic abnormalities, suggesting that broad reduction of C9orf72 translation is likely to be safe [98].
The discovery of C9ORF72 may reveal important mechanistic information about the molecular pathophysiology of FTD and ALS and may provide novel treatment strategies. If loss of function is implicated, strategies to increase levels of C9ORF72 or augment C9ORF72 function may be needed. If expanded RNA transcripts are responsible, ongoing clinical trials for non-coding repeat expansion disease such as myotonic dystrophy type 1 (DM1) and fragile X-associated tremor/ataxia syndrome (FXTAS) may provide starting points for decreasing RNA transcription or altering RNA structure and aggregation. ASOs may be a unique, targeted therapy for patients carrying the C9orf72 expansion. The discovery of poly-(glycine-proline) peptides may provide a useful early, sensitive and specific biomarker for C9ORF72 causes of FTD, both diagnostically and as a pharmacodynamic tool, allowing for accurate targeted intervention.
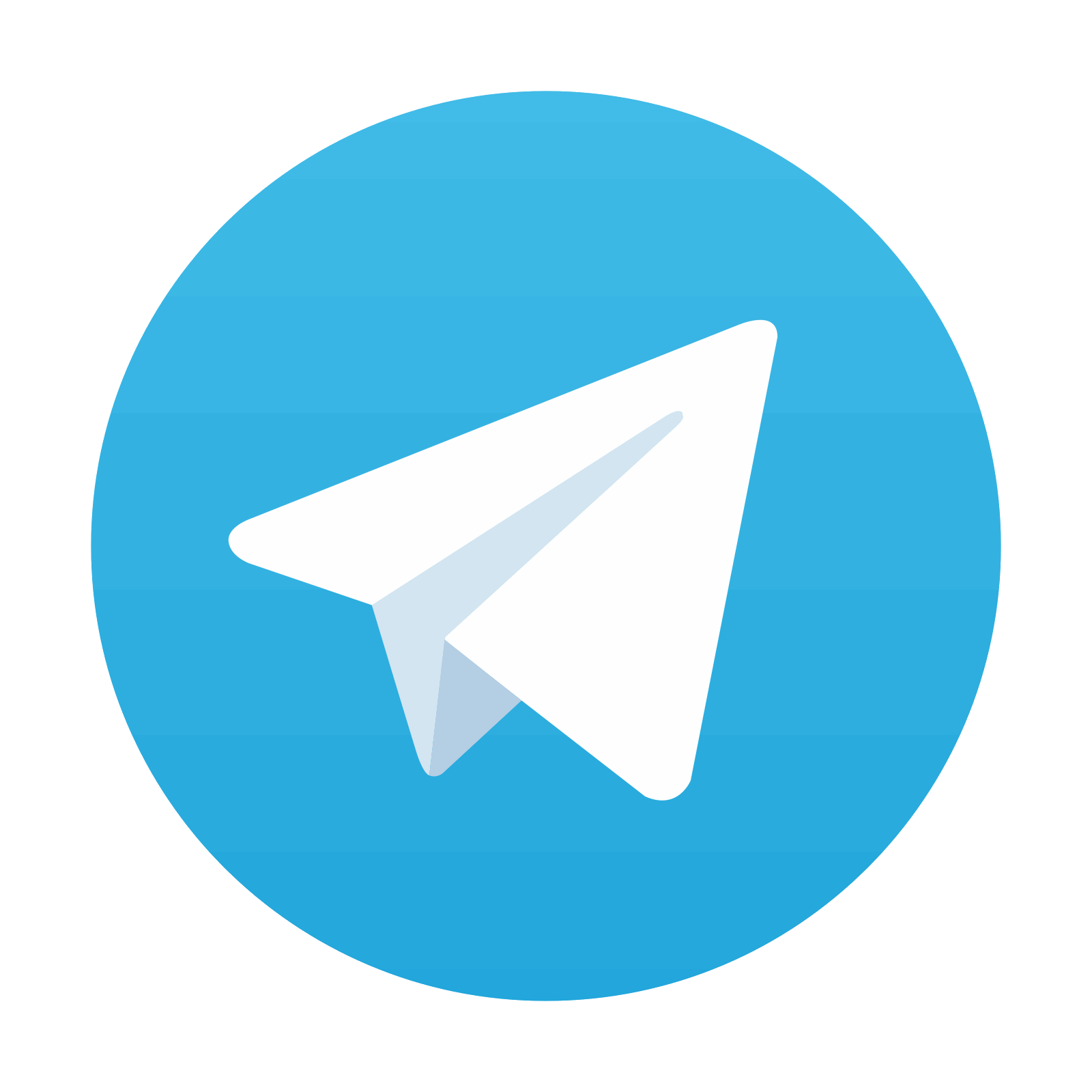
Stay updated, free articles. Join our Telegram channel

Full access? Get Clinical Tree
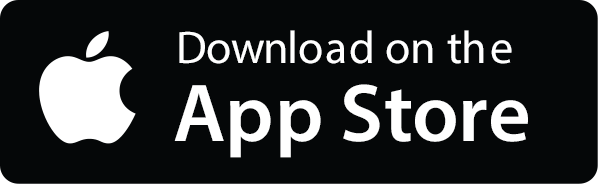
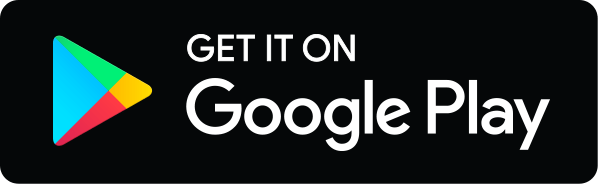