(1)
Sonno Sleep Centers, El Paso, TX, USA
Keywords
PhylogenySleep disordersModel organisms Drosophila melanogaster Genetics and sleepSleep and longevityUnihemispheric sleepThe “witchery of sleep” has historically been a topic of inquiry for many diverse scientific disciplines as well as a source of personal fascination for most people.
The cry for sleep is ever greater than the cry for bread. Existence depends on both; but we eat to sleep, while we sleep to live. Sleep is of far greater importance than food for the preservation of life. [1, p. 21]
This mysterious brew of an as-yet-undeciphered formula of genetic, electrophysiological, psychological, neurochemical, and molecular events has been implicated in determining mortality and morbidity; in affecting mental and physical health; and in altering memory, cognition , and quality of life. The study of sleep, once confined almost exclusively to the scientific laboratory, has cultivated an entire specialized field of sleep disorders medicine. However, despite dramatic increases in knowledge about basic sleep mechanisms and human sleep disorders, the function and purpose of sleep still continue to remain touched by the diaphanous veil of witchery.
The universality of sleep, in fact, suggests that sleep serves an important biological function. Sleep has now been studied both behaviorally and electrophysiologically in a wide variety of organisms. The majority of sleep studies have been performed on familiar mammals such as mice, rats, cats, dogs, and humans. However, the phylogenetic study of sleep also encompasses nonmammalian organisms with wide variations in habitat, electrophysiology, and behavior. Insects, invertebrates, fish, birds, reptiles, amphibians, and monotremes (platypus and echidna) as well as more unusual mammals such as sloths, elephants, and cetaceans (whales and dolphins) have been studied behaviorally and in several cases electrophysiologically (for recent reviews, see [2–6]). By examining living organisms which have a long history represented in the fossil record, clues to the contribution of sleep in the survival of a species as well as the function of sleep in living species might be determined. Here, we discuss specific findings which have emerged from phylogenetic studies, drawing primarily on nonmammalian or ganisms, and which have significance for unraveling human sleep disorders.
The Definition of Sleep
The behavioral criteria for defining sleep are well known, and the application of these criteria permits the identification of sleep in diverse species. These criteria include: (1) a species-specific posture, (2) behavioral quiescence, (3) elevated arousal thresholds, (4) state reversibility to distinguish sleep from coma or torpor, and (5) a homeostatic response to sleep deprivation [4]. There is well-known electrophysiology which occurs in conjunction with these behavioral criteria. Nonrapid eye movement sleep (NREM) , defined by the presence of high-amplitude slow waves, occurs in cyclic alteration with rapid eye movement (REM) or paradoxical (PS) sleep, defined by the presence of REMs, skeletal muscle atonia, increases in brain temperature, male penile erections, and, in humans, mental activity. Since there is a close relationship between behavior and electrophysiology in mammals, electrophysiology substitutes for visual observations of the sleeping state in virtually all mammalian studies.
The high-amplitude slow waves of mammalian NREM sleep are not a well-defined electrophysiological feature during behavioral quiescence in nonmammalian vertebrates with the exception of birds [7]. This is not surprising given the absence of the thick neocortical layer responsible for generating slow waves in nonmammalian vertebrates. Additionally, REM sleep has not been convincingly demonstrated in nonmammalian organisms with the exception of brief REM sleep bouts, usually lasting less than 10 s, in birds. There have been relatively few electrophysiological recordings, with the exceptions of birds and reptiles, performed during behavioral quiescence in nonmammalian vertebrates. However, in contrast to the reliable expression of REM sleep in mammals, a wide spectrum of electrophysiology during behavioral sleep has been reported in nonmammals. These variations include an absence of electrical activity during behavioral wakefulness and isolated spikes or spike trains during behavioral quiescence in the octopus [8]; spikes during behavioral wakefulness and slower waves of 15–20 Hz in the crayfish [9]; a decline in local field potentials during behavioral quiescence in the fruit fly [10, 11]; high-amplitude spikes during behavioral sleep which disappear during behavioral waking in turtles, tortoises, lizards, and caimans [12–15]; and recently spindle-like activity during behavioral quiescence in the frog [16]. These differences from mammalian sleep electrophysiology have raised the issue of whether nonmammalian organisms exhibit “true” sleep. This debate has been particularly vigorous with respect to reptilian sleep [17, 18] since various investigators have reported the presence of slow waves characteristic of NREM sleep [19], the presence of REM sleep [20], the presence of slow waves during waking [21], and the presence of high-amplitude spikes during behavioral sleep which appear analogous to spikes recorded in the ventral hippocampus of mammals during slow-wave sleep (SWS) [14, 22]. These specific controversies are not reviewed here, but rather we examine those findings from phylogenetic studies which can provide insight into human sleep disorders.
Model Systems in the Study of Sleep
Studying sleep in new or unusual nonmammalian organisms is of intrinsic interest for understanding the diversity of behavioral and electrophysiological expressions which sleep can take in the animal world. Unfortunately, most nonmammalian phylogenetic studies have not substantially advanced our understanding of the specific genetic or molecular mechanisms which control the presence or absence of sleep. A recent extensive review by Toth and Bhargava [23] underlines the fact that mammals, including rats, mice, cats, and dogs, have most commonly served as subjects to study sleep. However, nonmammalian organisms, specifically the fruit fly ( Drosophila melanogaster) , the zebrafish ( Danio rerio), and the nematode roundworm ( Caenorhabditis elegans), share a large number of genetic homologues with humans, and they have provided powerful biological models for unraveling the complex molecular and genetic mechanisms which control not only human disease but also sleep mechanisms [24–27].
Drosophila (Fig. 62.1), which has arguably been used more than any other model organism for evaluating the genetics and neurochemistry of sleep , offers several practical advantages [28]. Flies, which have a well-studied genome, are readily available experimental organisms, and large colonies can be maintained in a relatively small space with cost efficiency as compared to most mammals. Furthermore, the life span and reproductive cycle are short, allowing for timely analysis of multiple genetic and molecular interventions, and the resulting large populations which can be generated increase statistical power in evaluating the effects of manipulations. Most major neurotransmitter systems including serotonin, dopamine, and epinephrine, which have been identified as having key roles in human sleep and waking, have also been identified in Drosophila. Zebrafish offers similar advantages as a model organism [29], and a recent detailed, quantified behavioral analysis of the roundworm ( C. elegans) during the quiescent periods accompanying lethargus reinforces the use of this organism as a model for studying sleep [30].
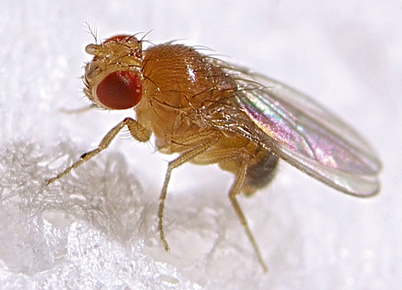
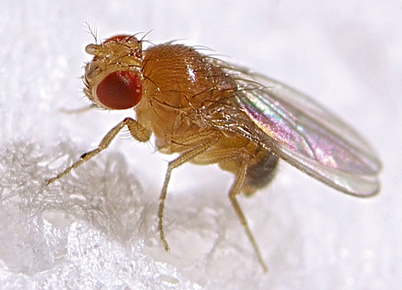
Fig. 62.1
A model organism for the study of sleep, Drosophila melanogaster. (Photo used with permission of Max Westby on Flickr)
Although there may be striking genetic similarities between these organisms and humans, a “model system” is a useful model only if the behavior of interest, sleep, is convincingly demonstrated to exist in these creatures. There are several lines of evidence which strongly suggest that the behavioral quiescence observed in Drosophila is analogous to sleep in humans and other mammals [28, 31]. First, the behavioral criteria for sleep are met in flies including a stereotypic posture during behavioral quiescence and elevated arousal thresholds. Deprivation of the quiescent state resulted in a homeostatic response, i.e., an increase in quiescence following deprivation of quiescence. The presence of sleep is also regulated by circadian clock genes. That this quiescent state following deprivation represents a sleep state different from a permutation in circadian clocks is indicated by a homeostatic response to rest deprivation in flies which were absent in the central clock gene, period. However, mutation of timeless, a second clock gene, did not result in a homeostatic response to deprivation [28, 31]. Further evidence comes from the response to drugs, including caffeine, modafinil, and methamphetamine, all of which produce alerting responses in Drosophila, similar to patterns of wakefulness in humans [32–34]. Antihistamines increase sleep amounts [31]. Finally, patterns of quiescence vary across the life span with age-related changes in sleep [35]. Thus, despite the apparent dissimilarity between Drosophila and humans, the fruit fly appears to be a good candidate for studying molecular and genetic sleep mechanisms potentially impacting sleep disorders.
There are several areas in which phylogenetic studies have provided new insights into sleep and the effects of sleep in humans.
Mortality/Aging/Insomnia
The connection between character and bedtime which grew up from association when human life was less complex than now has some counterpart in the world of butterflies and insects. The industrious bees go to bed much earlier than the roving wasps. The latter, which have been out stealing fruit and meat, and foraging on their own individual account, “knock in” at all hours til dark, and may sometimes be seen in a state of disgraceful intoxication, hardly able to find the way in at their own front door. The bees are all asleep by then in their communal dormitory. [36, p. 49]
It is well known that there are significant variations in sleep times throughout the animal kingdom [37]. The significance of these variations for determining life span or aging in various species or for promoting the survival of the species, however, is unclear. An important question, the answer to which is universally sought, is whether sleep duration has an impact on life span and mortality and whether life span can be extended by an optimal amount of sleep. In humans, there are now numerous epidemiological studies which suggest extremes of sleep length confer increased risk for mortality (for reviews, see [38–40]). Both short and long sleep times have been associated with increased mortality, and the best survival curves appear to be associated with a sleep duration of about 7 h per night. Somewhat surprisingly, in contrast to common recommendations for many years, 8 h or more of sleep per night has been reported to be associated with increased mortality [41]. Both short and long sleep has also been associated with the development of obesity, diabetes, hypertension , and cardiovascular disease [42]. The specific factors which produce these variations in mortality at both ends of the U-shaped spectrum are unknown. Although a causal relationship between sleep and mortality derived from epidemiological studies is an appealing one, a recent review raises the issue of whether variable methodologies and assumptions preclude any definitive conclusions about the relationship between sleep and mortality [43]. Of note is that very few polysomnographic studies have been performed over a prolonged period of time in a large number of subjects which suggest a relationship between mortality and sleep. In one study in adults followed up for a mean of 12.8 years, sleep latencies of greater than 30 min were associated with a 2.14 times greater mortality risk, and sleep efficiencies of less than 80 % were associated with a 1.93 times greater mortality risk [44]. More recently, polysomnographically determined sleep efficiency in subjective long sleepers with chronic heart failure predicted mortality [45]. To add to this mix on mortality and sleep duration, a dose–response relationship between hypnotics commonly used for insomnia treatment and an increased risk of death as well as an increased cancer risk for the most frequent hypnotic users has been reported [46]. Model systems may be excellent substrates to evaluate the significance of these findings on mortality.
Genetic mutants have been developed in the fruit fly to elucidate the molecular effects of sleep on mortality and aging. It would, of course, be of considerable interest to determine whether measures of sleep duration or sleep quality predict life span. Minisleep ( mns), Hyperkinetic ( Hk), insomnia-like ( ins-l), and Sleepless ( sss) are genetically engineered insomnia mutants which are each associated with marked reductions in total daily sleep time as compared to wild-type flies [47–51]. Even more striking is the finding that life span is reduced in short-sleeping flies, suggesting that sleep duration does, in fact, have a direct impact on longevity . It has been demonstrated that these mutations are mediated by Shaker, a gene which codes for the voltage-dependent potassium channel. In contrast to these findings, another short-sleeping mutant fly strain, Fumin ( fmn), which has alterations in the dopamine transporter gene (DAT), has normal longevity, and fmn mutants do not exhibit a homeostatic response to sleep deprivation, suggesting that dopamine transporter activity is a critical element in sleep homeostasis [52]. Also of interest are the findings that a high-calorie diet in fmn mutants produces even further reductions in already-shortened sleep and markedly reduces longevity, suggesting that accelerated aging and shortened sleep are strongly influenced by elevated caloric intake [53]. Other studies also demonstrate that pharmacological and genetic manipulation of the dopaminergic system in Drosophila affects sleep and arousal [32]. Finally, mutations of the fragile X mental retardation gene ( Fmr1) in Drosophila reveal that overexpression of dFmr1 results in increased sleep amounts, and complete loss of dFmr1 expression results in flies with significantly longer sleep amounts as compared to controls. Relevant to the short and long sleep associated with increased mortality in humans, both short- and long-sleeping flies had shortened life span [54]. Similar to fmn mutants, flies with both dFmr1 overexpression and loss of expression did not exhibit a homeostatic rebound in sleep following 24 h of sleep deprivation.
These studies appear to be consistent with much of the data obtained from human studies and provide new insights into the specific genetic mechanisms which are responsible for short sleep. However, the literature has still not answered the specific question of how much sleep is required for a maximal life span. Some of this ambiguity is likely to be the result of technical issues in both human and Drosophila studies. For example, subtle differences in survey questioning presented by different investigators may lead to human over- or underestimation of sleep time [43]. In the case of the Drosophila, there may be significant variations in experimental conditions which produce inconsistent results relative to estimating life span [55, 56]. Seemingly minor factors, including the type of food and social isolation, can interact with genetic background to affect sleep variability in Drosophila [57]. Furthermore, the commonly studied Canton-S strain of flies obtained from different sources exhibits marked differences in total amounts of nighttime sleep depending upon the source of origin, and wild-caught flies from different climates and altitudes also show differences in sleep fragmentation with age [58]. Other nonmammalian studies suggest that various environmental conditions can significantly affect the expression of sleep. For example, differing electrophysiological manifestations of behavioral sleep in Caiman sclerops were found under differing environmental recording conditions, and indeed at least part of the differences in these studies may be directly traced to inadequate environmental adaptation [12, 19]. Social influences and diet can also affect sleep amounts, interact with genetic makeup, and potentially affect longevity in Drosophila [57, 59]. Changes in environmental conditions can improve sleep consolidation in old flies [60]. Finally, one of the most commonly used technologies in determining amounts of Drosophila sleep, the Drosophila Activity Monitoring System (DAMS), substantially overestimates amounts of fly sleep in comparison to more detailed digital video analysis [61].
The genetically and neurochemically engineered sleeplessness of fruit flies and zebrafish raises the issue of whether this sleeplessness is functionally comparable to sleeplessness as it occurs in nature. Recent studies in the Mexican cave fish ( Astyanax mexicanus) shed new light on evolutionarily derived sleeplessness and provide new insights into the effect of habitat on the development of sleep and arousal systems ([62, 63]; Fig. 62.2). Surface- and cave-dwelling populations of these fish differ significantly in daily amounts of sleep with surface fish averaging more than 800 min and three different cave-dwelling populations averaging 110–250 min per day. Blockade of B-adrenergic receptors with propranolol produced a dose-dependent increase in total sleep time, an increase in sleep bout length, and a decrease in sleep bout number in cave fish without any effect at any dose on the sleep of surface-dwelling fish. B-1-, B-2-, and B-3-adrenergic antagonists did not affect sleep in surface dwellers, but cave fish sleep increased significantly in response only to the B-1 antagonist atenolol with a near statistically significant sleep increase in response to the B-3 antagonist SR59203A. Neuroanatomical studies demonstrated that the number of catecholamine neurons was conserved in cave fish as compared to surface-dwelling fish. These findings suggest that evolution has resulted in an increase in the adrenergic arousal system in the cave fish as compared to surface dwellers. Other recent studies in the killifish ( Nothobranchius korthausae) and the three-spot wrasse ( Halichoeres trimaculatus) provide evidence for the improvement in the circadian rhythmicity of aging fish and in the induction of quiescence by melatonin [64, 65].
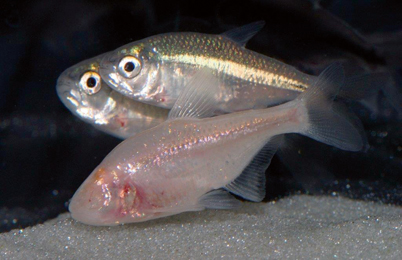
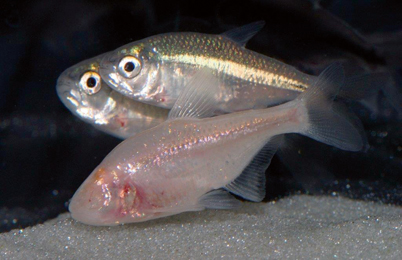
Fig. 62.2
Mexican cave fish ( Astyanax mexicanus) surface dwelling ( top) and cave dwelling ( bottom). (Photo used with permission of Richard L. Borowsky, Ph.D.)
In summary, human epidemiological studies suggest that sleep duration is associated with life span, and Drosophila studies indicate that this relationship is probably not as straightforward as determining a golden standard for nightly sleep duration, independent of other factors, for the maximization of life span. Genetics, social influences, and environmental factors, largely uncontrolled in human studies, clearly have a powerful influence on sleep amounts and, potentially, longevity. Furthermore, new studies in cave fish may provide a natural model of ecologically distinct sleeping and waking behaviors. Although there are methodological issues which contribute substantially to the findings on mortality and sleep in humans and even with findings from the Drosophila studies, an unambiguous answer to the question of how much sleep is necessary for maximum longevity still remains unanswered.
Pharmacological Development
The genetic correspondence between human disease genes and Drosophila genes offers a unique substrate for studying not only human disease processes but also sleep disorders [26] . Similarly, approximately 70 % of human genes have at least one zebrafish orthologue, and, as a result, zebrafish have been extensively used in developing human disease models [25, 66]. These correspondences between human genes and genes of model systems suggest that model organisms may be of importance in developing in vivo drug treatments at a molecular level for modifying or treating human sleep disorders. Screening of a large number of compounds utilizing behavioral measures of waking and quiescence in larval zebrafish have revealed important insights into mechanisms of drug action including the similar effects on waking and quiescence produced by major neurotransmitter pathways in both zebrafish and mammals, better prediction of the differential effects of poorly understood compounds on waking and quiescence, and identification of additional pathways of drug action on quiescence not previously known [67]. In Drosophila, amylase is a biomarker of increased sleep drive following sleep loss , and an increase in human salivary amylase demonstrates similar properties following sleep deprivation [34]. Furthermore, two Drosophila genes identified in ins-l mutants were modulated in their human homologues following human sleep deprivation [51].
These findings suggest that Drosophila could be effectively used to evaluate the effects of or to be utilized in the development of medications with alerting or sleep-inducing properties. In addition, pharmacological studies in other nonmammalian vertebrates, for example, fish [63–65] and reptiles [68, 69], suggest that these organisms may provide clues about the phylogenetic development of neurochemical mechanisms in the control of sleeping and waking. Although molecular studies in model systems suggest the development of more effective treatments for insomnia, this promise has not been fully realized in the development of safe, effective, widely used, well-tolerated treatments for humans .
Sleep Disorders
Narcolepsy
There has been remarkable progress in understanding the underlying causes of narcolepsy which has resulted directly from animal research (for recent reviews, see [70] and [71]). The discovery of orexin (hypocretin) deficiency resulting from the loss of orexigenic neurons in narcoleptic dogs and mice and the subsequent discovery of the human leukocyte antigen DQB1*0602 and DQA1*0102 in almost all patients with narcolepsy have led to the conclusion that narcolepsy is an autoimmune disease. However, the mechanism or mechanisms by which orexin depletion occurs is still unknown, although upper airway infections and H1N1 flu vaccination in genetically susceptible individuals have been identified as potential environmental triggers in this process [72, 73].
Potentially effective treatments for narcolepsy arising out of animal studies appear to be within the closest reach. Gene replacement therapy in orexin-deficient mice restores sleep consolidation and the timing of REM sleep, but does not improve cataplexy [74], whereas gene transfer into the zona incerta in orexin -deficient mice improved cataplexy, but did not improve sleep fragmentation [75]. In humans, an orexin antagonist has recently been demonstrated to improve sleep in insomniac humans [76]. Gene therapy clinical trials are now being performed worldwide for a number of human diseases including, for example, cancer, cardiovascular diseases , Parkinson’s disease, and cystic fibrosis [77]. Unlike current narcolepsy drug treatments which target symptom improvement in excessive daytime sleepiness, the results of gene transfer therapy in animal models suggest that this approach has the potential to produce a treatment which targets the molecular and cellular deficits in human narcolepsy.
It does not seem likely that nonmammalian models of narcolepsy are on the horizon, in large part because, with the exception of birds which have very brief bouts of REM sleep, REM sleep has not been convincingly demonstrated. Although the zebrafish does not exhibit behavioral characteristics of narcolepsy per se, the overexpression of hypocretin/orexin as well as null mutations for hypocretin/orexin produce increases in locomotor activity, decreases in arousal thresholds, and shortened, fragmented sleep in the dark [78, 79]. Also of interest is that Yokogawa and colleagues [79], during the course of evaluating hypocretin receptor mutants, demonstrated that normal adult zebrafish maintained under constant light conditions exhibit an almost complete suppression of sleep. There was no homeostatic rebound in response to this sleep suppression, but a progressive return to sleep occurred after 1–2 weeks. Similarly, following sleep deprivation with electrical stimulation, no sleep rebound occurred upon release into light, but release into darkness resulted in a homeostatic sleep rebound. Exposure to light alone during the last 6 h of the biological night resulted in a marked suppression of sleep without a subsequent rebound upon release into darkness. These findings raise the issue of whether these anomalies in sleep homeostasis and sleep suppression in response to light are factors which may decrease the usefulness of the zebrafish as a model organism for the study of human disorders such as narcolepsy . Additionally, both the absence of behaviors such as cataplexy in the zebrafish and the absence of REM sleep suggest that this model system is unlikely to offer significant advantages beyond the current mammalian models in exploring narcolepsy.
Sleep Apnea
Insomnia is the most common sleep complaint, typically occurring in about one third of the population, but sleep apnea has more visibly alarming and recognizable symptoms including loud snoring, periods of observable breath holding, vigorous movements, and excessive daytime sleepiness. Often, these symptoms are not recognized as having direct links to cardiovascular disease , metabolic disorders, and cognitive deficits, thus increasing the potential medical complications related to sleep apnea. A familial, and possibly a genetic, component to sleep apnea has been recognized for several years [80–82]. A naturally occurring model of sleep apnea has been described in the English bulldog which has crowded upper airway anatomy similar to human sleep apnea patients [83], and sleep apnea has been reported in obese miniature pigs [84]. However, sleep apnea has not been described in nonmammalian organisms.
Through artificial occlusion of the airway and exposure to repetitive hypoxemia, induced sleep apnea in dog, rat, and mice preparations has been used to demonstrate the physiologic effects of sleep apnea [85–88]. These mammalian studies have shed new light on, for example, the cardiovascular and neurochemical consequences of sleep apnea [89, 90], but model organisms have not been forthcoming in determining the genetic basis for the mechanisms of this disorder. Given the myriad of factors which impact the expression of sleep apnea including age, obesity, gender, and factors such as alcohol consumption in addition to influences on cardiovascular, metabolic, and respiratory systems, it seems likely that a different animal model will be required. No genome-wide association studies (GWAS) which involve scanning the DNA of a large population to determine specific genetic markers of a particular disease, in this case sleep apnea, have been performed. The gene encoding the allele APOE e4, which plays a critical role in cholesterol metabolism and transport, has been associated with obstructive sleep apnea in both adults and children, but a meta-analysis of studies examining APOE and sleep apnea concluded that the evidence for this association is weak [91]. In an additional meta-analysis of sleep apnea, genetic association studies revealed only one association, TNFA rs1800629, which was significantly associated with obstructive sleep apnea [92]. Thus, the molecular basis of sleep apnea remains murky in humans, and animal studies or model systems have not, to date, added significant clarification to the genetic or neurochemical basis of this disorder.
Restless Legs Syndrome
There have been substantial advances in understanding the genetic underpinnings of restless legs syndrome in humans, largely as the result of large-scale GWAS . Genetic loci have been identified at MEIS1, BTBD9, and MAP2K5/SKOR1 which are associated with increased risk for restless legs syndrome [93–95]. Naturally occurring restless legs or periodic limb movements have not been described in either the mammalian or nonmammalian animal literature. However, the identification of these human genetic loci has stimulated the development of a Drosophila model of restless legs syndrome [96].
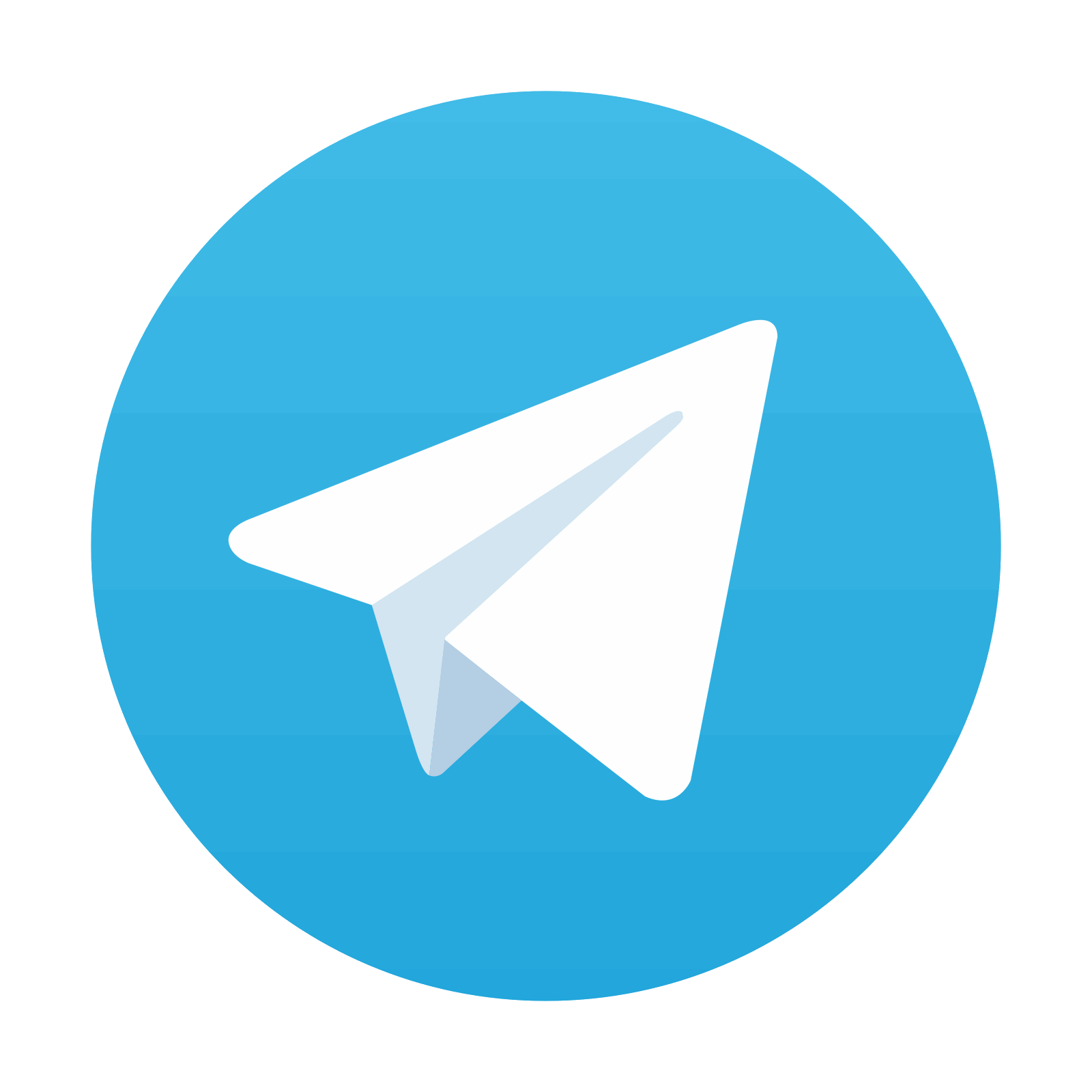
Stay updated, free articles. Join our Telegram channel

Full access? Get Clinical Tree
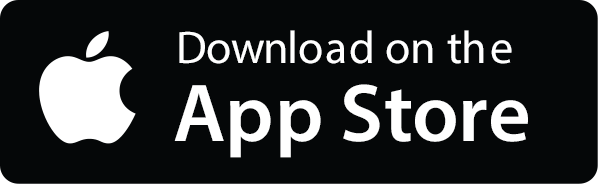
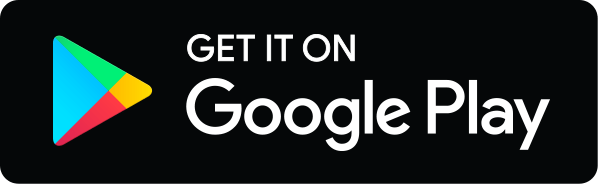