Fig. 10.1
Sustained increase of oxygenated hemoglobin (oxyHb) signals during treadmill walking in a patients with cerebellar ataxia. While oxyHb signals in the prefrontal cortex gradually decreased during treadmill walking at a steady speed in a healthy subject, a patient with cerebellar ataxia showed sustained increase of oxyHb signals (Adapted from Mihara et al. 2007)
Neural mechanisms for balance control can be assessed by using an event-related fNIRS. A time-line analysis revealed that prefrontal activation was induced to adapt to postural perturbation delivered by combined brisk forward and backward movement on a platform. Activation in the right posterior parietal cortex and supplementary motor area (SMA) was enhanced when auditory warning signals were provided before the delivery of perturbation (Mihara et al. 2008). In a cross-sectional study of balance recovery after hemiplegic stroke, postural perturbation-related cortical activation was observed in the prefrontal cortical areas in both hemispheres, as well as the premotor and parietal cortex in the unaffected hemisphere. There was a significant correlation between balance ability as assessed by Berg Balance Scale, and activation in the SMA and prefrontal cortex (Mihara et al. 2012a). In the subsequent longitudinal observation, postural perturbation-related cortical activation in the SMA of the affected and unaffected hemispheres was significantly increased after intensive rehabilitation (Fujimoto et al. 2014). The increment of the postural-perturbation-related oxygenated hemoglobin signals in the SMA of the unaffected hemisphere, significantly correlated with the gain in balance function as measured by the Berg Balance Scale (Fig. 10.2). These findings support that idea that the SMA plays an important role in postural balance control, and suggest that the SMA is a crucial area for balance recovery after a hemiplegic stroke. Although these refer to mechanisms underlying balance recovery after a hemiplegic stroke, similar observations are made in patients with degenerative cerebellar disease (data unpublished).
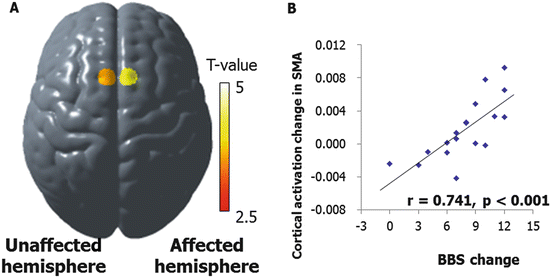
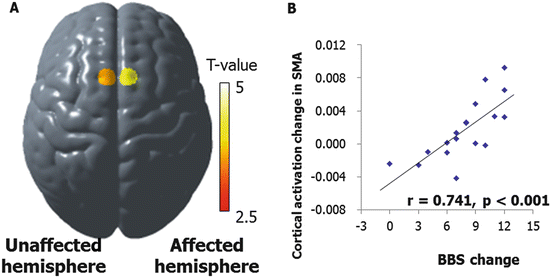
Fig. 10.2
Cortical changes underlying balance recovery in patients with hemiplegic stroke. Increase in SMA activities (a): BA 6, MNI coordinate x/y/z = −9.0/6.6/76. 0in the unaffected hemisphere, 7.8/7.0/75.9 in the affected hemisphere) of the supplementary motor area (SMA) showed significant correlation with improvement of balance score as measured by Berg Balance Scale after intensive rehabilitation for 41 days in average (b). Longitudinal data are from 20 hemiplegic patients with first-ever subcortical stroke (mean ± SD age: 62.2 ± 12.6, 11 males) (Adapted from Fujimoto et al. 2014)
In degenerative cerebellar diseases, it is to be elucidated whether similar mechanisms works or not, since pathological processes are basically progressive. It is possible that certain plastic changes contributing to functional improvement after rehabilitation cannot be taken over in the next phase of the disease. Regarding this issue, previous functional neuroimaging studies have suggested that cerebellar dysfunction might be compensated for by neural networks other than the cerebellum, including frontoparietal cortical areas, especially those involved in the cerebellar-cortical loops. In patients with multiple system atrophy, hand movement induced less bilateral cerebellar activation and greater left superior parietal activation than in healthy subjects. They also had less bilateral cerebellar and greater supplementary motor and left superior parietal activation than patients with Parkinson disease (Payoux et al. 2010). It is also of interest whether such functional modulation leads to structural reorganization. A recent study using voxel-based morphometry (VMA) implied that balance training improved balance performance in patients with degenerative cerebellar diseases, along with gray matter increase in the non-affected cerebral cortex as well as the less affected cerebellar cortex within the cerebellar-cortical loops. The most compelling change in gray matter volume was seen in the dorsal premotor cortex that is shown to project to the primary motor cortex and cerebellar motor areas, including lobules VI and Crus I and VIII (Burciu et al. 2013). It is to be elucidated whether such changes reflect a recovery from degeneration or intact processes of cerebellar plasticity in the remaining healthy tissue. In terms of the impact of rehabilitative intervention on damaged neural networks, further investigations will be required to discover whether enhanced physical activities induce delay pathological process in the affected area. In animal models of spinocerebellar ataxia type 1 (SCA1) caused by expansion of a translated CAG repeat in Ataxin-1, exercise induced up-regulation of epidermal growth factor as well as expanded life span, although the impact on motor function was unclear (Fryer et al. 2011).
10.3 Specific Issues in Considering Neurorehabilitation to Cerebellar Degenerative Diseases
The fundamental question regarding the efficacy of neurorehabilitation in cerebellar degenerative diseases is whether it is truly beneficial in terms of both short- and long-term effects. In considering the dose-dependent effect of task-specific training in stroke patients (Kwakkel et al. 1999, 2004), it is assumed that such patients have preserved their motor learning ability and that the disease process is basically non-progressive. Thus two important issues should be considered to answer this question. The first is whether impaired motor learning due to cerebellar dysfunction is compensated for by repeated practice, i.e. intensive task-oriented rehabilitation, since the cerebellum plays a crucial role in motor learning (Ito 2001).
Motor learning is essential to learn to use chopsticks, play a musical instrument, ride a bicycle, and swim. The main component of motor learning is the improved performance realized by repeating a task-oriented practice. In an experimental setting, motor learning includes both adaptation learning and motor sequence learning. Adaptation learning is a form of motor learning based on sensory information, such as skill acquisition for using a computer mouse, controlling a robot arm under a modified force field, and prism adaptation. The transformation process of sensory information to motor command is captured as an internal model. Internal models include both forward and inverse models (Wolpert and Kawato 1998; Wolpert and Ghahramani 2000). Forward internal models can predict subsequent sensory consequences from efference copies of issued motor commands. Inverse internal models calculate the necessary feedforward motor commands from the desired trajectory information (Fig. 10.3, Wolpert and Kawato 1998; Kawato 1999; Kitazawa 2013). In the cerebellum, feedback-error learning contributes to the formation of internal models. Climbing fibers from the inferior olivary nucleus send error signals to the cerebellar cortex, and internal models are then acquired based on synaptic plasticity of the Purkinje cells, i.e. long-term depression (Ito and Kano 1982).
On the other hand, motor sequence learning, the knowledge of the sequence of a series of movements is acquired by repeating those serial movements. This form of motor learning can be regarded as the formation of an internal model for the motor sequence to move smoothly by predicting the next movement. In a rehabilitation setting, the repeated practice of ADL including transfer from bed to wheelchair and toileting, is aimed to complete these behavioral series safely and automatically. Thus in terms of motor learning, the cerebellum play a crucial role for supervised learning based on errors, while the basal ganglia are engaged in reinforcement learning based on reward prediction (Doya 1999; Schultz et al. 1993). Acquired motor skills are consolidated and retained in the cerebellar-cortical loops and striatal-cortical loops. For retention, it is assumed that retention of adaptation learning and motor sequence learning, mainly depend more on the cerebellum and related cortical regions, and the striatum and related cortical regions, respectively (Doyon and Benali 2005; Doyon 2008).
How well can patients with cerebellar damage learn motor skills? An adaptation learning task consisting of throwing balls at a target wearing a prism, revealed that prism adaptation was impaired or absent in patients with olivo-cerebellar lesions, except lesions in the dentate-thalamic projection (Martin et al. 1996). In an experiment using eye-blink conditioning, patients with degenerative cerebellar diseases showed inappropriately-timed conditioned responses with respect to the conditioned stimulus (Topka et al. 1993). Hatakenaka et al. (2012) investigated the relationship between motor learning and gains in ADL after intensive rehabilitation in patients with infratentorial stroke. Fast motor learning was assessed by the repetition of 30 s pursuit rotor (PR) tasks. Both patients and controls learned the PR skill, although the gains in PR performance were significantly lower in patients. In terms of brain activities, cerebellar patients showed sustained prefrontal activation, while healthy subjects showed reduced prefrontal activation coupled with increased activation in the supplementary motor area after repeated PR tasks. These findings suggest that feedback control of the PR task shifted to feedforward control with task repetitions in healthy subjects, while cerebellar patients continued to rely on feedback information. Furthermore, reduced learning significantly correlated with smaller ADL gains assessed by the Functional Independence Measure (FIM, Keith et al. 1987). Thus impaired motor sequence learning by the PR task was correlated with reduced gains after intensive rehabilitation for ataxic stroke patients. To relearn motor sequences required to perform ADLs, such as transfer, toileting, and dressing independently, preserved motor learning may influence learning efficacy. This indicates that augmented practice may be an optimal strategy to compensate for the impaired motor learning in patients with cerebellar damage. Regarding this issue it is reasonable to test whether intensive rehabilitation induces clinically significant gains in degenerative cerebellar diseases.
The second issue is how long functional gains can be sustained, provided that intensive rehabilitation results in significant gains. To answer this question, longitudinal assessment after focused rehabilitative intervention, including comparison of data for natural decline, will be necessary. The results from a European cohort (Jacobi et al. 2011) indicated that the annual increase of the Scale for the Assessment and Rating of Ataxia (SARA, Schmitz-Hubsch et al. 2006; Yabe et al. 2008) score was greatest in SCA1 (2.18 ± 0.17, mean ± SE) followed by SCA3 (1.61 ± 0.12) and SCA2 (1.40 ± 0.11). SARA progression in SCA6 was slowest and nonlinear (first year: 0.35 ± 0.34, second year: 1.44 ± 0.34). In a Japanese cohort of patients with SCA 6, there was a 3.1 ± 4.3 point change of the International Corporative Ataxia Rating Scale (Trouillas et al. 1997) for 4 years in a retrospective study, and the change of SARA scores was 1.5 ± 2.0 per year in a prospective follow-up (Tsuji et al. 2008; Nakajima et al. 2009). Thus no significant difference in the functional status between assessment at the baseline and 1-year follow-up might be interpreted as an improvement. However, to elucidate if repeated rehabilitative intervention may affect the natural history of degenerative diseases, longitudinal evaluation over further years may be necessary. Individual variables, including gender, age at onset, duration of disease and genetic factors such as length of CAG repeat, also influence long-term outcomes (Klockgether et al. 1998; Jacobi et al. 2011; Schmitz-Hubsch et al. 2008), and these factors need to be adjusted.
10.4 Effect of Intensive Rehabilitation in Degenerative Cerebellar Diseases
There have been two studies testing the effect of intensive rehabilitation on cerebellar ataxia, gait and balance in patients with degenerative cerebellar diseases (Ilg et al. 2009, 2010; Miyai et al. 2012). These studies have shown that the immediate effect of intensive rehabilitation focusing on balance and mobility function is warranted (Table10.1). Ilg et al. (2009) provided 1 h of coordinative training for 3 days per weeks, for 4 weeks, in 10 patients with cerebellar degeneration, 6 with degeneration of afferent pathways. The rehabilitation program included static balance e.g. standing on one leg, dynamic balance e.g. sidesteps, climbing stairs, whole body movements to train the trunk-limb coordination, steps to prevent falling and falling strategies in order to prevent trauma, and movements to treat or prevent contracture especially movements of the shoulders and spine. At the end of the session, patients received an individual written training schedule to enable them to perform the exercises by themselves at home for 1 h each day. Significant improvements in gait performance, including velocity, lateral sway, and intralimb coordination and reduction of ataxia symptoms including regulation of balance in static and dynamic balance tasks, were observed in patients with cerebellar degeneration. These improvement were sustained at a follow-up assessment 8 weeks after the intervention. Of note, these interventions were not effective in patients with afferent ataxia. Subsequent follow-up study revealed that these gains in gait and ataxia were significant at 1 year (Ilg et al. 2010).
Table 10.1
Results of intensive rehabilitation in patients with degenerative cerebellar diseases
Miyai et al. (2012) | ||
---|---|---|
Number of Pt | 16 | 42 |
Type of disease | SCA6(2),SCA2(1),ADCA(1), IDCA(6)/FA(3),SANDO(2),SN(1) | SCA 6(20), ADCA(6),IDCA (16) |
Age ± SD (Range) | 61.4 ± 11.2 (44 ~ 79) | 62.5 ± 8.0 (40 ~ 82) |
Gender | 8 M, 8 F | 22 M, 20 F |
Duration of disease | 12.9 ± 7.8 (3 ~ 25 years) | 9.8 ± 6.2(7 month ~ 30 years) |
Baseline SARA | 15.8 ± 4.3 (11 ~ 24) | 11.3 ± 3.8 (5 ~ 21.5) |
Control | No | Crossover for short-term effect |
Intervention | 1 h × 3 per week × 4 weeks | 2 h × 5 + 1 h × 2 per week × 4 weeks |
Post training | Home training protocols | Unmonitored self-training |
Outcome measures | SARA, gait speed, balance, BBS, GAS | SARA, FIM, gait speed, cadence, FAC, falls |
Assessment point | 4 weeks pre, baseline, post, 8 weeks, 1 year | Baseline, post 0, 4, 12, 24 weeks |
Main results | SARA & gait improved 8 weeks & 1 year post rehabilitation only in patients with cerebellar ataxia not afferent ataxia | SARA & gait improved 12 weeks & 24 weeks, respectively |
In a study by Miyai et al. (2012), patients with degenerative cerebellar disease received 4-week inpatient rehabilitation, including 1-h physical therapy and 1-h occupational therapy per day on weekdays, and 1 h of either physical or occupational therapy on weekends (Cerebellar Ataxia Rehabilitation: CAR trial). Forty-two patients with spinocerebellar degeneration were randomly assigned to the immediate group or the delayed-entry control group. The short-term outcomes of the immediate and control group were then compared. Long-term evaluation of both groups took place at 4, 12, and 24 weeks after the intervention. Each therapy focused on the improvement of ataxia and ADL. Physical therapy emphasized improving postural balance and gait, and the program included general conditioning, range-of-motion exercise for trunk and limbs, muscle strengthening, static and dynamic balance exercises with standing, kneeling, sitting, and quadruped standing, mobilizing the spine in both prone and supine positions, walking indoors and outdoors, and climbing up and down stairs. The occupational therapy program emphasized improving ADL and the program comprised relaxation, hygiene, dressing, writing, eating, toileting, bathing, balance exercises, reaching, coordinative tasks of the upper limbs and trunk, and dual motor tasks such as handling objects while standing and walking. After discharge, patients were instructed to keep to a similar level of activity in their daily lives as they did before. Patients receiving home-based rehabilitation at a baseline level, usually 20–40 min of physical therapy per week, were advised to continue the program after discharge at the same frequency as they did before admission. The immediate group showed significantly greater functional gains in ataxia, gait speed and ADL than the control group. The improvement of truncal ataxia was more prominent than that of limb ataxia. These gains in ataxia and gait were sustained at 12 weeks and 24 weeks, respectively (Figs. 10.4 and 10.5). At least one measure was better than at the baseline, at 24 weeks in 22 patients. Patients with sustained improvement had less severe ataxia (lower SARA score) than those without sustained improvement (8.9 ± 0.7 vs. 12.7 ± 0.9, p < 0.05). The receiver operator characteristic curve (ROC) analysis showed that the baseline SARA score was moderately predictive of the sustained improvement at 24 weeks.
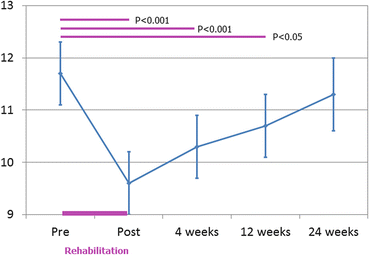
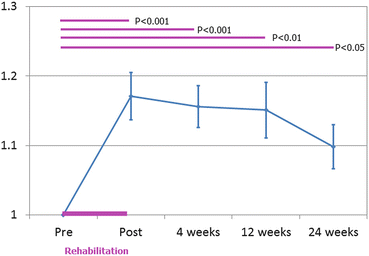
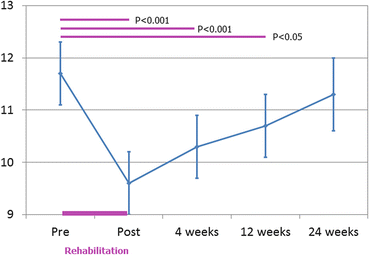
Fig. 10.4
Longitudinal changes of SARA after intensive rehabilitation (n = 41). Although functional status tended to decline to the baseline level within 24 weeks, gain in SARA score was significant up to 12 weeks (Wilcoxon signed-rank test)
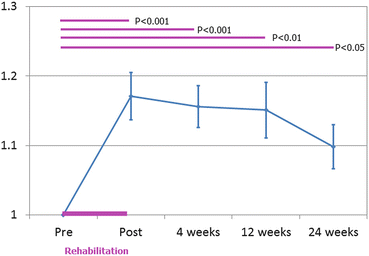
Fig. 10.5
Longitudinal changes of normalized gait speed after intensive rehabilitation (n = 40). Gait speed was the most sensitive to changes. There was a 17 % increase in gait speed immediately after the intervention and still 10 % increase at 24 weeks after the intervention
Although these studies show the significant effect of focused rehabilitation in patients with degenerative cerebellar disease (Table 10.1), several unresolved questions remain, including dose and duration of intensive rehabilitation, identification of the specific components of intervention that work, strategies that maintain functional gains after intensive rehabilitation, strategies for patients with severe ataxia, strategies for patients presenting with additional pyramidal/extrapyramidal signs, i.e. multiple system atrophy, and strategies for enhancing outcome by the combination of rehabilitation and pharmacological neuro-modulation/brain stimulation (Ilg et al. 2014). In terms of the minimum dose required to obtain meaningful gains, it seems to be at least 3 h per week for 4 weeks. It is unclear whether greater amounts of intervention will result in further improvement since the functional outcome of balance and gait after intervention of 14 h per week for 4 weeks seems to be comparable with the outcome after 3 h per week for 4 weeks. This then raises the possibility that home-based intensive rehabilitation could be a feasible intervention for patients with degenerative cerebellar diseases. Other issues will be discussed in the following sections.
10.5 Specific Components of Intervention
As described in the previous section, the rehabilitative approach to degenerative cerebellar diseases is comprehensive, and includes practice for improving balance, gait and ADL. This raises the question of whether there is a specific intervention that can improve cerebellar ataxia. A similar problem emerged with strategies of motor rehabilitation in stroke patients. Several meta-analyses from small randomized controlled trials, have shown that strategies focused on high-intensity and repetitive task-specific practice, are likely to promote motor recovery after stroke (Langhorne et al. 2009). During the last decade, there has been an accumulation of several well-designed large-scale randomized control trials. In particular, the EXCITE (Extremity Constraint Induced Therapy Evaluation) trial (Wolf et al. 2006) is the first large multi-center randomized controlled trial for the efficacy of constraint movement induced therapy (CIMT), designed according to CONSORT (Consolidated Standards of Reporting Trials) (Altman et al. 2001; Schulz et al. 2010). In this study, 222 participants who had suffered a stroke within the previous 3–9 months, were assigned to receive either CIMT) or usual and customary care (ranging from no treatment after concluding formal rehabilitation, to pharmacologic or physiotherapeutic interventions). The CIMT group, where patients wore a restraining mitt on the less-affected hand, while engaging in repetitive task practice and behavioral shaping with the hemiplegic hand, showed greater gains than the usual care group in both timed tasks of the affected upper extremity, and in the measure of daily use of the affected upper extremity. These improvements in arm motor function persisted for at least 1 year.
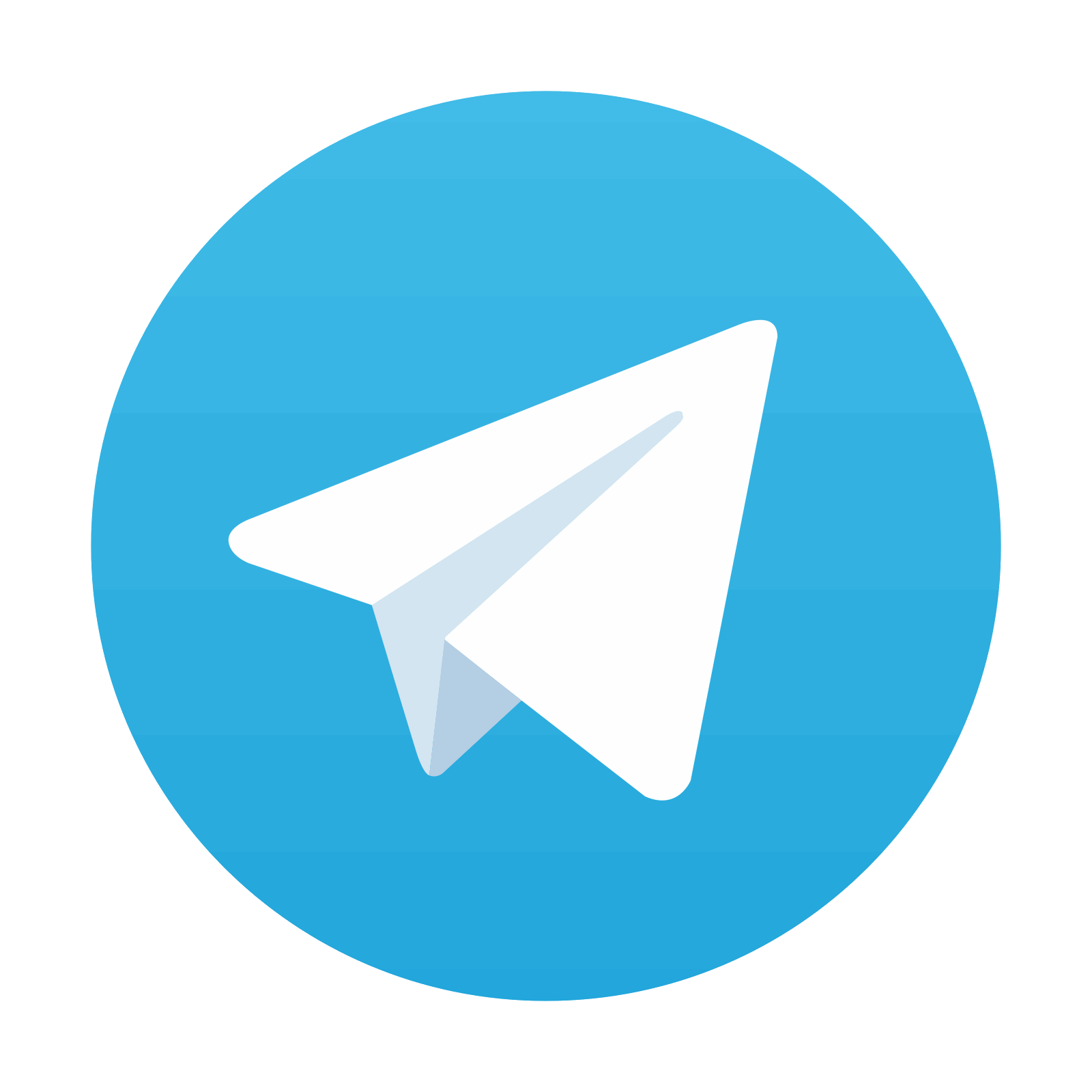
Stay updated, free articles. Join our Telegram channel

Full access? Get Clinical Tree
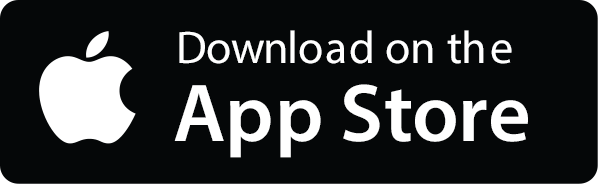
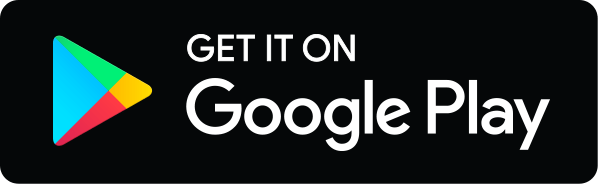