Ion
Intracellular (mM)
Extracellular (mM)
Equilibrium potential (mV)
Potassium (K*)
140–150
5
–90
Sodium (Na*)
5–15
145
+60
Chloride (Cl)
4–30
110–125
–70
Synaptic transmission is the major mode of transmission of information from one neuron to another. Each synapse has a presynaptic terminal containing neurotransmitters in vesicles and a postsynaptic terminal containing receptors for the neurotransmitters. Neurotransmitters are released from the presynaptic terminal when an action potential causes sufficient change in the voltage (depolarization) at the presynaptic terminal to activate voltage-gated calcium channels allowing calcium to enter into the presynaptic terminal. This triggers a cascade of events leading to the fusion of presynaptic vesicles with the membrane of the presynaptic terminal, thereby releasing neurotransmitter molecules into the synaptic cleft. Binding of neurotransmitters to the receptors on the postsynaptic terminal activates ion channels associated with them, allowing the passage of ions leading to local changes in membrane potential (Fig. 1.1). Such local changes in membrane potential are known as postsynaptic potentials (PSPs) which are nonpropagated small-amplitude potentials lasting 10–100 ms. PSPs can be excitatory (excitatory postsynaptic potential (EPSP)) or inhibitory (inhibitory postsynaptic potential (IPSP)) depending on the type of ion channel activated and the electrochemical gradient for ions that can pass through the channel. EPSPs generally result from an inward flow of positive ions such as sodium or calcium and cause depolarization (excitation), thus decreasing the threshold for triggering an action potential in the postsynaptic terminal. On the other hand, IPSPs result from an inward flow of negative ions (e.g., chloride) or outward flow of positive ions (e.g., potassium) and cause hyperpolarization (inhibition), thus increasing the threshold for triggering an action potential in the postsynaptic terminal.


Fig. 1.1
Membrane potential (MP) changes and current flows during synaptic activation. a The MP of the postsynaptic neuron and the MP of the presynaptic fibers are recorded by means of intracellular microelectrodes. Action potentials in the excitatory and inhibitory presynaptic fibers lead to excitatory postsynaptic potential (EPSP) and inhibitory postsynaptic potential (IPSP), respectively, in the postsynaptic neuron. Two EPSPs sum up to a superthreshold potential, triggering an action potential in the postsynaptic neuron. b During EPSP and IPSP, ionic current flows occur through as well as along the neuronal membrane, as shown by arrows. The density of + and – signs indicates the polarization of the subsynaptic (dark area) as well as that of the postsynaptic membrane during synaptic activation. Adapted from [1]
A single EPSP or IPSP is not sufficient enough to move the membrane potential of the postsynaptic terminal to or away from the threshold for triggering of action potential. Summation of several PSPs is necessary for that purpose. Such summation can be spatial (summation of several PSPs in the vicinity) or temporal (summation of several PSPs occurring in quick succession). Neurons generally have extensive dendritic arborization, and hundreds to thousands of axons can form synapses on a neuron’s dendrites. Each neuron receives synaptic inputs from multiple sources at the same time—spatial and temporal summation of these inputs determines whether or not the neuron fires an action potential.
EEG Generator
A large number of EPSPs and IPSPs generated in a complex network of neurons alter the overall excitability of the neurons in the network. Such PSPs generate an extracellular field potential (Fig. 1.2) that changes over time which is believed to be the basis of potentials recorded on EEG. The extracellular field potential is a secondary phenomenon resulting from the development of potential gradients between areas of localized membrane potential change and the remaining areas of the neuronal membrane. A ‘sink’ is generated at the site of an EPSP because of an inflow of positive ions into the localized area of the neuron, and there is a corresponding ‘source’ at a distance where positive ions come out of the neuron; current flows from the ‘source’ to the ‘sink’ in the extracellular space giving rise to the extracellular field potential. Thus, a recording electrode close to the synapse receiving an excitatory input (EPSP) would record a negative potential because of an inward flow of positive ions causing negativity in the extracellular space nearby, whereas a deep recording electrode at a distance would record positivity because of an outflow of positive ions associated with the current flowing through the extracellular space. The reverse is true for an inhibitory input (IPSP): A recording electrode close to the synapse receiving an inhibitory input (IPSP) would record a positive potential because of an inward flow of negative ions (or an outward flow of positive ions), whereas a recording electrode at a distance would record negativity because of an outflow of negative ions (or an inflow of positive ions) associated with the current flowing through the extracellular space.


Fig. 1.2
Basic mechanisms underlying the generation of potentials (electroencephalogram (EEG)) and of magnetic fields (magnetoencephalogram (MEG)) in the extracellular space of central nervous system. The description is based on the assumption that an extended neuronal process, e.g., a dendrite, is locally depolarized by the activation of an excitatory synapse. Adapted from [1]
Therefore, polarity of extracellular field potentials recorded by surface electrodes on EEG depends on the direction of current flow as well as on the position of the electrode relative to the location of the generator. This translates to the fact that superficial EPSPs and deep IPSPs will show the same polarity (negative) on a surface recording electrode. Likewise, superficial IPSPs and deep EPSPs will show the same polarity (positive) on a surface recording electrode (Fig. 1.3). Thus, orientation of neurons and their processes, as well as location of synaptic contacts with respect to the cortical surface, are important determinants of extracellular field potentials recorded by EEG electrodes.


Fig. 1.3
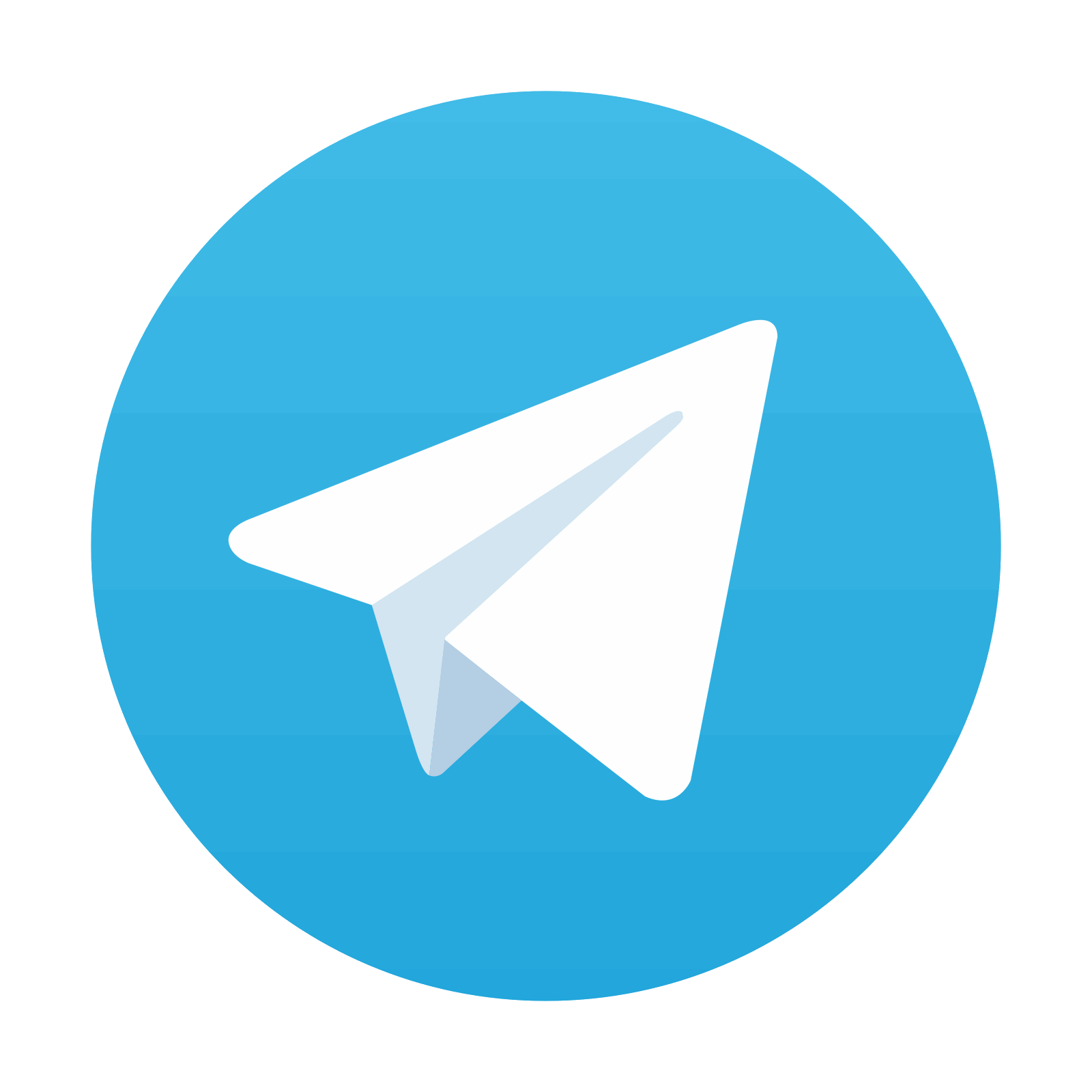
Membrane potential (MP) changes and field potentials (FPs) elicited by the activation of excitatory and inhibitory synapses in the central nervous system. The elementary processes are explained by means of a neuronal element (hatched area), the one end of which contracts the surface of a structure in the central nervous system. The MP of the neuron element is recorded at both ends by the microelectrodes ME1 and ME2. The extracellular field is picked up at the surface of the neuronal structure by the electrode E1, as well as in the vicinity of ME2 by the electrode E2. Active excitatory and inhibitory synapses are marked by open triangles and black triangles (S), respectively. a(1) The inward current at S generates an EPSP that appears in the region of ME1, as well as in that of ME2. Because S is located superficially, the FP generated, due to the direction of the extracellular current flow (arrows), is of negative polarity at the surface (E1) and of positive polarity in the deeper recording (E2). a(2) The activation of a deep excitatory synapse elicits a current flow with inverse direction as compared with a(1). Therefore, the extracellular FP consists in a positive deflection at the surface and in a negative one at the depth. b(1) The outward current at S generates an IPSP in the region of ME2, as well as in that of ME1. Due to the direction of the extracellular current flow, the FP generated consists in a positive fluctuation in the depth (E2) and in a negative one in the surface recording (E1). b(2) The current flow during the activation of a superficial inhibitory synapse is inverse as compared with b(1). Therefore, the FP recorded from the surface consists of a positive fluctuation. Differences in the time course of the various potentials are caused by the electrical properties of the tissue. c Schematic summary of the polarity of the potential recorded on the scalp with an EEG recording electrode based on the occurrence of (superficial vs. deep) EPSP or IPSP. Adapted from [1]
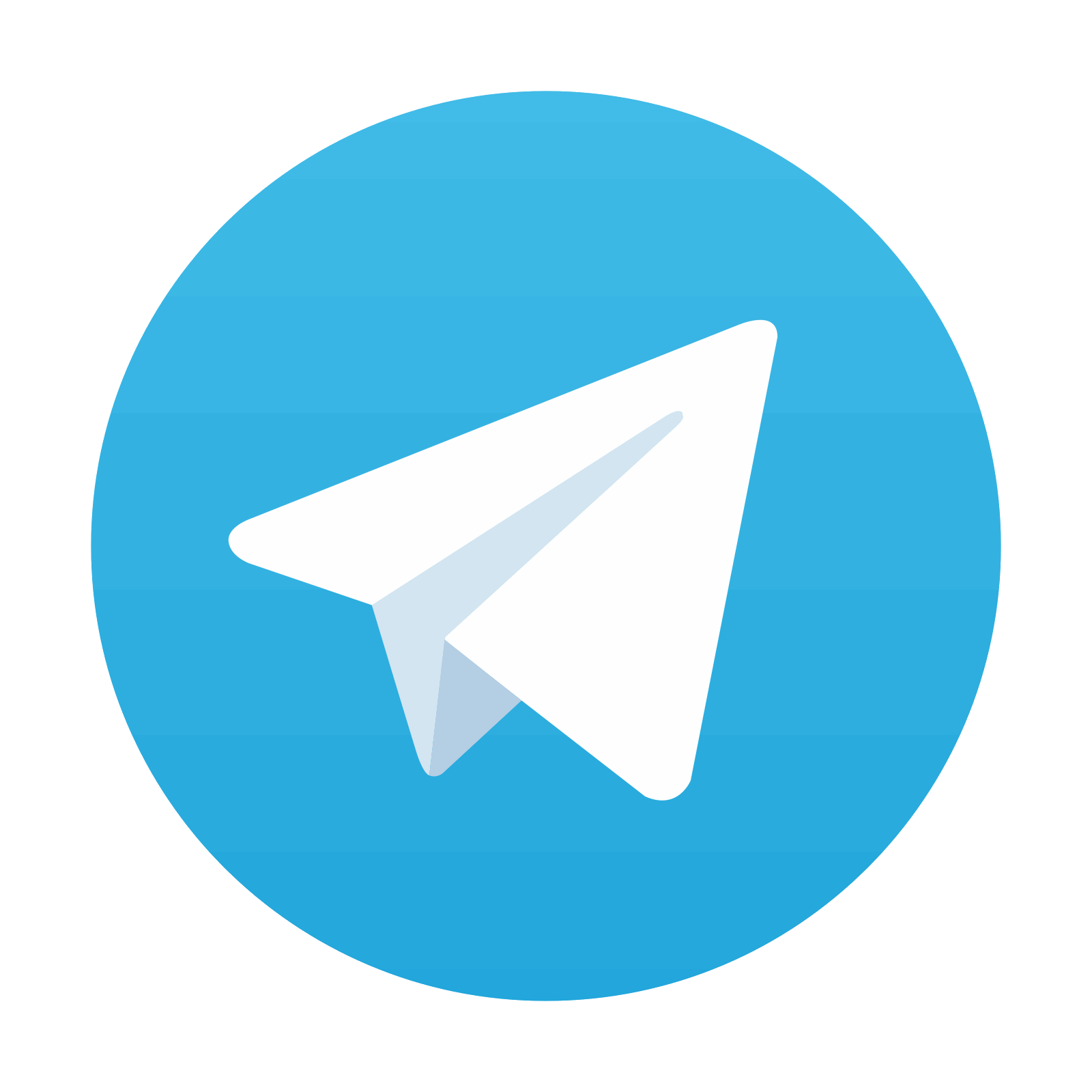
Stay updated, free articles. Join our Telegram channel

Full access? Get Clinical Tree
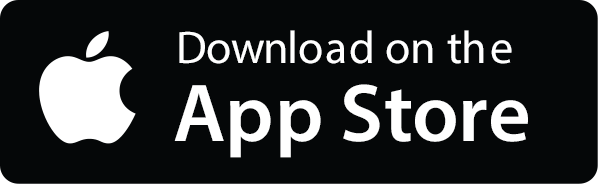
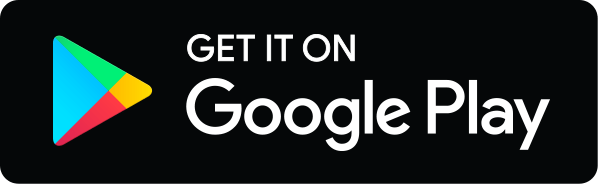
