Fig. 3.1
Relationship between CSF–serum ratio of blood (white circles) and brain-derived (black circles) compounds and their hydrodynamic radius under “steady-state” conditions (Modified according to Felgenhauer (1995)). Alb albumin, α2-M α2-Makroglobulin, Ca calcium, Cl chloride, Cp ceruloplasmin, EPO erythropoietin, Ig immunoglobulin, PO 4 phosphate, Ser serine, Thr threonine)
The basic principles of secretion and transport at the blood–CSF barrier are identical to those of the blood–brain barrier. These are active transportation and facilitated diffusion, so that the inner lining of the plexus (epithelium) is either characterized by secretion or absorption of isotonic fluid (Davson et al. 1970). The most important transportation system at the blood–CSF barrier is the sodium+/potassium+ ATPase located at the apical ciliated ridge (microvilli) of the plexus choroideus (Keep et al. 1999). In addition, carboanhydrase for the exchange of HCO−3 and Cl− plays a role. Blocking these transportation systems is used therapeutically (furosemide, acetazolamide) to reduce CSF formation (Carrion et al. 2001). Although the main route of transport is unidirectional from the blood to the subarachnoid space, there is also a bidirectional transport at the blood–CSF barrier (Oreskovic and Klarica 2010; Brinker et al. 2014). This way, certain substrates (inorganic ions, metabolites of neurotransmitters, antibiotics) can be removed from the brain and the CSF. According to a recent two-photon imaging study visualizing fluorescent tracers through a cranial window of living mice, a significant proportion of CSF recycles into the brain along paravascular pathways mediated by aquaporin-4 channels (Iliff et al. 2012). The authors conclude that AQP4-mediated water flow along vessels may be relevant for clearing brain interstitial water, interstitial waste, and soluble proteins.
3.4 CSF Proteins and Factors Influencing Their Concentration
The composition of the lumbar CSF has been intensively studied, since the introduction of the spinal tap more than a century ago (Quincke 1891; Wynter 1891). The CSF is a cell-free clear fluid and contains approximately 0.3 % plasma proteins, or approximately 150–450 mg/L, depending on age and sampling site (ventricular vs. lumbar).
The majority of the protein content of the lumbar CSF is blood derived (80 %), and the remainder consists of brain-derived or intrathecally produced proteins (20 %) (Fig. 3.1, Tables 3.1 and 3.2) (Felgenhauer 1974; Thompson and Zeman 1992).
Table 3.1
Major blood-derived proteins in CSF (80 % of total CSF proteins)
Compound | CSF | Blood (serum or plasma) | CSF–serum ratio | ||
---|---|---|---|---|---|
Reference range (mean) | |||||
Total protein | 200–500 | mg/L | 60–80 | g/L | |
Albumin | 150–350 | mg/L | 35–55 | g/L | <8 × 10−3 |
IgG | <40 | mg/L | 7–16 | g/L | <6 × 10−3 |
IgA | <6 | mg/L | 0.7–4.0 | g/L | <4 × 10−3 |
IgM | <1 | mg/L | 0.4–2.3 | g/L | <1.8 × 10−3 |
Table 3.2
Mainly brain-derived proteins in CSF (20 % of total CSF proteins)
Compound | CSF | Blood (serum or plasma) | Intrathecal synthesis (%) | |||
---|---|---|---|---|---|---|
Reference range (mean) | Noteworthiness | |||||
Transthyretin | 17 | mg/L | 250 | mg/L | 93 | Produced by choroid plexus; thyroid transporter |
Prostaglandin-d synthase (beta-trace) | 15 | mg/L | 0.5 | mg/L | >99 | Marker for CSF fistula; enzyme and retinol transporter |
Cystatin C (γ-Trace) | 3 | mg/L | 0.5 | mg/L | >99 | Proteinase inhibitor |
Apolipoprotein E | 6 | mg/L | 93.5 | mg/L | 90 | Lipid transporter |
Neopterin | 4.2 | nmol/L | 5.3 | nmol/L | 98 | Marker for microglia and macrophage activity |
Neuron-specific enolase (NSE) | 5 | mg/L | 6 | μg/L | >99 | Marker for neuronal damage |
Glial fibrillary acidic protein (GFAP) | 0.12 | mg/L | 0 | 100 | Marker for glial activity or damage | |
Ferritin | 6 | mg/L | 120 | μg/L | 97 | Marker for subarachnoid bleeding |
S-100 protein | 2.9 | mg/L | 0.12 | μg/L | >99 | Marker for glial activity or damage |
Myelin basic protein (MBP) | 0.5 | mg/L | 0 | 100 | Marker for myelin damage | |
Tau protein | 0.2 | mg/L | 0 | 100 | Marker for neuronal and axonal damage | |
Neurofilaments | 0.1 | mg/L | 0 | 100 | Marker for axonal damage |
Under physiological conditions, blood-derived proteins enter the CSF compartment via passive diffusion across the blood–CSF barrier. Depending on their molecular size and their blood concentration, CSF proteins of blood origin show a specific CSF-to-blood ratio The larger the protein size, the higher the concentration gradient between blood and CSF (e.g., IgM > IgA > IgG > albumin) (Fig. 3.1, Tables 3.1 and 3.2) (Felgenhauer 1974).
When molecular size and serum levels are known, the CSF concentration of a given blood-derived protein can be calculated. A concentration in the CSF exceeding the calculated value indicates a local production of that given protein within the CNS, i.e., intrathecal synthesis (Felgenhauer 1995).
Erythropoietin (EPO) in CSF may serve as an example (Fig. 3.1): Based on the molecular weight (30 kd) and the corresponding hydrodynamic radius of 24 Å, the estimated CSF–serum ratio of EPO would be 0.01, if EPO found in CSF originated from the blood compartment via passive diffusion only. The CSF–serum concentration ratio of EPO in symptomatic controls (tension-type headache) appears however to be 0.126. Therefore, the approximate proportion of CSF EPO originating from intrathecal synthesis can be estimated being 92 % (Widl et al. 2007). Additional evidence for its intrathecal origin is that CSF EPO concentrations are independent of blood–CSF barrier function, i.e., there is no Qalb.-related increase of EPO in the CSF.
Another way to determine the extent to which a given protein is produced within the CNS formula taking into account the serum concentration of the respective protein (e.g., IgG) and the albumin CSF-to-serum ratio can be used. Various formulae such as IgG index, IgG synthesis rate, and IgG(loc) have been developed to discriminate between blood- and brain-derived fractions of IgG (Link and Tibbling 1977; Tourtellotte et al. 1980; Reiber and Felgenhauer 1987).
Another important factor influencing the protein content is the permeability at the blood–CSF barrier (Felgenhauer et al. 1976). The function of the blood–CSF barrier is best characterized by the albumin CSF-to-serum ratio, since albumin is exclusively produced in the liver and not by the nervous system (Brettschneider et al. 2005).
In blood–CSF barrier dysfunctions (elevated albumin CSF–serum ratio), the concentration of proteins originating from blood (IgG, IgA, IgM) increases in accordance with a nonlinear hyperbolic function (Reiber 1994).
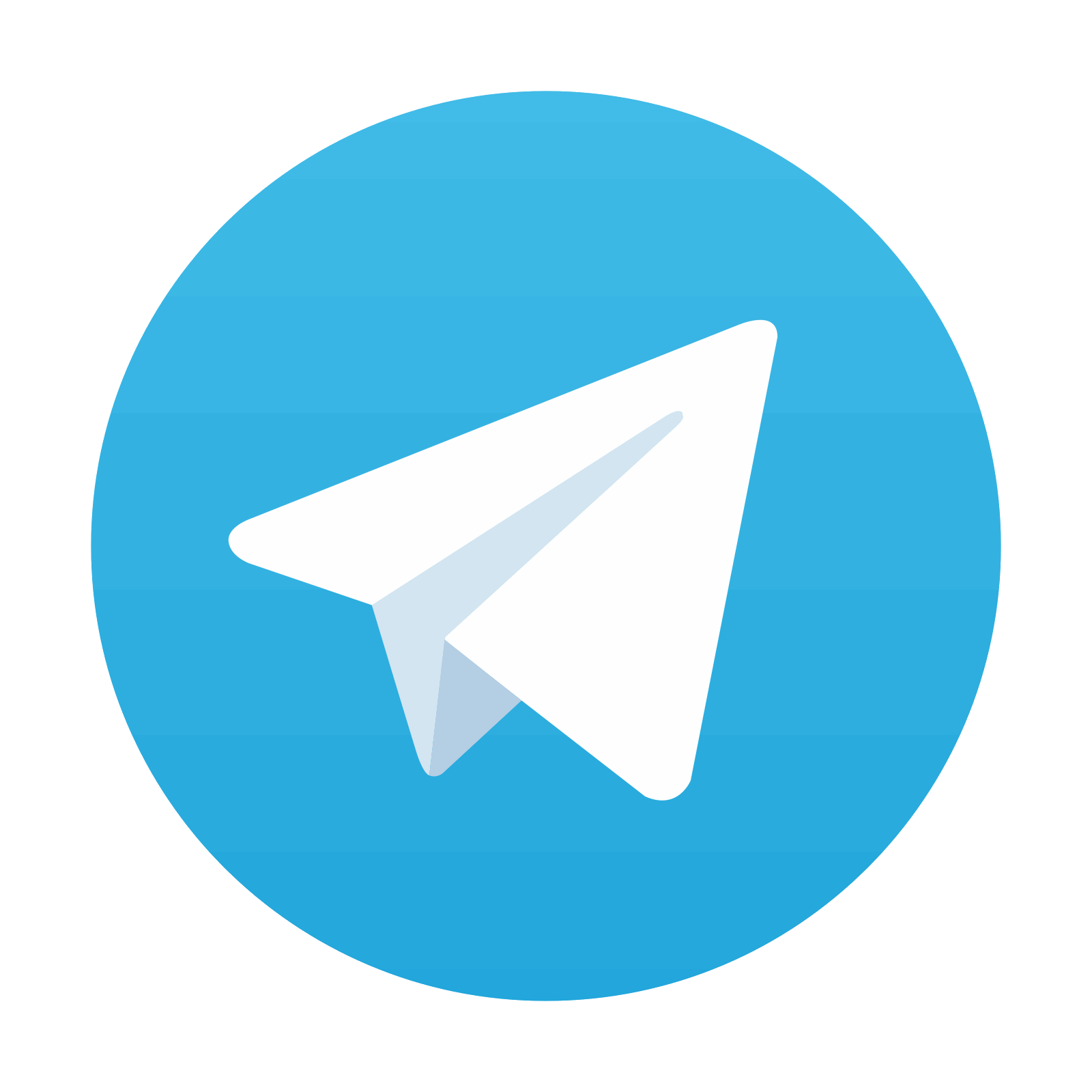
Stay updated, free articles. Join our Telegram channel

Full access? Get Clinical Tree
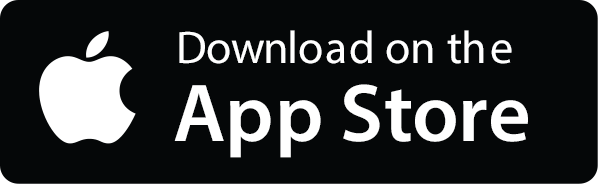
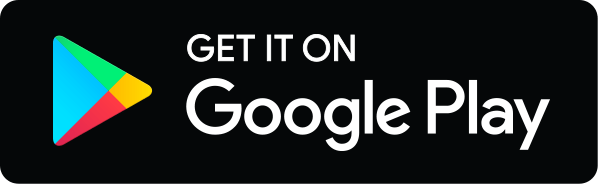