Chapter 1
Polysomnographic Recording Technique
Patient Preparation and Laboratory Environment
The goal of a PSG study is to recreate a typical night’s sleep for the patient so that recorded parameters are most clinically relevant. Towards this end, most modern sleep laboratories have bedrooms that are quiet, clean, comfortable, and tastefully decorated. Televisions, Internet and telephone access, adjacent bathrooms, and individual temperature control are standard in most laboratories. Many patients are encouraged to bring their own pillows, blankets, pajamas, and reading material to improve the sense of familiarity, which eases patient anxiety and makes sleep easier. Within reason, lights-out and lights-on times (the beginning and the ending of the recording) should match the patient’s regular bedtime and waking time, so as to prevent falsely shortened or prolonged sleep onset and rapid eye movement (REM) sleep latencies. Children being studied in the sleep laboratory may often present with special needs, and this is discussed in depth in Chapter 17.
As mentioned, simultaneous video and audio recording to monitor behavior during sleep is invaluable during the PSG. It is advantageous to use two cameras to view the entire body. A low-light-level camera should be used to obtain good quality video in the dark, and an infrared light source should be available after turning the laboratory lights off. The monitoring station should have remote control that can zoom, tilt, or pan the camera for adequate viewing, and technicians should be alert to any abnormal movements and adjust the view accordingly. The camera should be mounted on the wall across from the head end of the bed. An intercom from a microphone near the patient should be available. Technicians should also document the nature of any abnormal behavior and whether the patient was easily awakened from it, and they should regularly inquire about recollection of the episode, as well as dream recall (especially in a patient with suspected REM behavior disorder).
Technical Considerations and Polysomnography Equipment
Sensitivity is expressed in microvolts per millimeter or millivolts per centimeter. Sensitivity switches should be adjusted to obtain sufficient amplitude for interpretation. Sensitivity and filter settings vary according to the physiological characteristics recorded (Table 1.1).
Electroencephalography
The main purpose of EEG recording performed during PSGs is to distinguish between wakefulness and the various stages of sleep. This is further elaborated on in Chapter 3. According to the 2007 AASM Manual for the Scoring of Sleep and Associated Events, a minimum of three channels (F4-M1, C4-M1, O2-M1) representing the right frontal, central, and occipital electrodes referenced to the contralateral mastoid electrode is recommended, with corresponding backup electrodes over the left hemisphere (F3-M2, C3-M2, O1-M2) referenced to the contralateral mastoid electrode, in case of malfunction of the primary electrodes. Although the montage just described would theoretically be sufficient to detect a posterior dominant rhythm in wakefulness (best seen in occipital leads) and major sleep architecture (vertex waves, sleep spindles, and K complexes, best seen in frontal and central derivations), there are serious limitations to adhering to this minimum prescribed montage. Recording over only one hemisphere may result in inability to score sleep accurately if that hemisphere is lesioned (as in a patient with stroke or tumor) or in missing serious pathological conditions if the contralateral hemisphere is involved. The absence of a temporal lead may result in missing epileptiform activity, which is most common in this region. The American Academy of Sleep Medicine (AASM) also recommends an alternative derivation that includes the midline and a central channel (Fz-Cz, Cz-Oz, C4-M1); however, in addition to the same concerns that arise with the recommended standard derivation, another limitation with this montage is the tendency for midline, centrally predominant activity such as sleep spindles, K complexes, and slow wave sleep to be attenuated and easily missed. This would pose a particular problem in elderly patients while scoring stage N3 sleep, in which the slow wave activity must meet particular amplitude criteria (see Chapter 3). Therefore we recommend a montage that records over both hemispheres and includes the temporal regions (Table 1.2) in addition to electrodes recommended by the AASM for the scoring of sleep. For patients in whom nocturnal seizures are suspected or likely to occur, a full seizure montage with parasagittal and temporal chains is recommended (Table 1.3; see also Fig. 2.1). It is important for the polysomnographer and the polysomnographic technologist to be familiar with major patterns of EEG abnormalities that may be encountered during PSG recording (see Chapter 2).
Table 1.2
Typical Overnight Polysomnographic Montage Used in Our Laboratory
Channel Number | Name |
1 | F3-M2 |
2 | C3-M2 |
3 | T3-M2 |
4 | O1-M2 |
5 | F4-M1 |
6 | C4-M1 |
7 | T4-M1 |
8 | O2-M1 |
9 | Left electro-oculogram (E1-M1) |
10 | Right electro-oculogram (E2-M1) |
11 | Chin EMG |
12 | ECG |
13 | Heart rate |
14 | Left gastrocnemius EMG |
15 | Left tibialis anterior EMG |
16 | Right gastrocnemius EMG |
17 | Right tibialis anterior EMG |
18 | Intercostal EMG |
19 | Oronasal thermistor∗ |
20 | Nasal pressure transducer∗ |
21 | Chest |
22 | Abdomen |
23 | Snoring |
24 | Arterial oxygen saturation |
ECG, Electrocardiogram; EMG, electromyogram.
Channels 1 to 8 record electroencephalographic activity from bilateral cerebral hemispheres in a referential chain; electrode designation per the 10-20 international system of electrode placement. M1 and M2, left and right mastoid, respectively. Channels 19 and 20 record airflow (“flow channels”). Channels 21 and 22 record respiratory effort (“effort channels”) (see also Fig 1.1).
∗In a continuous positive airway pressure (CPAP) titration study, flow channels are replaced by a CPAP signal (C-flow signal).
Table 1.3
Extended Electroencephalographic (“Seizure”) Montage
Channel Number | Name |
1 | F4-M1 |
2 | C4-M1 |
3 | O2-M1 |
4 | C3-M2 |
5 | Fp1-F7 |
6 | F7-T3 |
7 | T3-T5 |
8 | T5-O1 |
9 | Fp2-F8 |
10 | F8-T4 |
11 | T4-T6 |
12 | T6-O2 |
13 | Fp1-F3 |
14 | F3-C3 |
15 | C3-P3 |
16 | P3-O1 |
17 | Fp2-F4 |
18 | F4-C4 |
19 | C4-P4 |
20 | P4-O2 |
21 | Left electro-oculogram (E1-M1) |
22 | Right electro-oculogram (E2-M1) |
23 | Chin EMG |
24 | Right masseter EMG |
25 | Left biceps EMG |
26 | Right biceps EMG |
27 | Left tibialis anterior EMG |
28 | Right tibialis anterior EMG |
29 | Intercostal EMG |
30 | Oronasal thermistor∗ |
31 | Nasal pressure transducer∗ |
32 | Chest |
33 | Abdomen |
34 | Snoring |
35 | Arterial oxygen saturation |
36 | ECG |
37 | Heart rate |
ECG, Electrocardiogram; EMG, electromyogram.
Channels 1 to20 record electroencephalographic activity from bilateral cerebral hemispheres with referential and bipolar montages, including both temporal and parasagittal chains; electrode designation per the 10-20 international system of electrode placement. Channels 30 and 31 record airflow (“flow channels”). Channels 32 and 33 record respiratory effort (“effort channels”) (see also Fig. 2.1).
∗In a continuous positive airway pressure (CPAP) titration study, flow channels are replaced by a CPAP signal (C-flow signal).
Electro-oculography
The underlying concept is that the eye is an electric dipole, with relative positivity at the cornea and a relative negativity at the retina. Any eye movement changes the orientation of the dipole, and it is the movement of the dipole that is recorded as a potential difference between the two electrodes used to record the EOG. In this arrangement, conjugate eye movements produce out-of-phase deflections in the two channels, whereas the EEG slow activities contaminating the eye electrodes are in phase. The sensitivity and filter settings for EOG are similar to those used for EEG (see Table 1.1).
Eye movements are generally characteristic of the sleep stage in which they occur and are an essential part of scoring. Eye blinks, seen only in wakefulness, are conjugate vertical eye movements occurring at 0.5 to 2 Hz with the eyes open or closed. Rapid eye movements (conjugate, irregular, sharp eye movements with an initial deflection of less than half a second) occur in wakefulness along with high chin EMG tone, eye blinks, and a posterior dominant rhythm, but they also occur in REM sleep, especially in phasic REM, where they occur in bursts seen in all directions (horizontal, oblique, and vertical) and are accompanied by low to absent chin tone (interspersed with similar phasic bursting) and a desynchronized, amorphous EEG pattern. In REM sleep, rapid eye movements are frequently preceded by sawtooth waves (see Fig. 3.15), although both may occur independently. The frequency with which bursts of rapid eye movements occur in REM sleep is called REM density. It typically increases in later REM cycles during the course of a normal PSG; this may be reversed in patients with depression.
Slow lateral eye movements are seen in drowsiness and light sleep and are defined as conjugate, sinusoidal, regular eye movements with an initial deflection of greater than half a second (Fig. 1.1). These eye movements are not under voluntary control and cannot be volitionally simulated. In patients who do not generate a posterior dominant rhythm, their appearance heralds stage N1 sleep. Although they may persist into stage N2 during the early part of the night, they generally disappear in stage N3 and REM sleep. However, patients on antidepressants such as selective serotonin reuptake inhibitors (SSRIs) like fluoxetine and paroxetine, as well as serotonin-norepinephrine reuptake inhibitors (SNRIs) such as duloxetine, may have unusual eye movements that appear to be a mixture of rapid and slow eye movements occurring well into stage N3 and often into REM sleep (colloquially referred to among polysomnographers as Prozac eyes); their presence makes sleep staging difficult (Fig. 1.2) and can render scoring of a multiple sleep latency test (MSLT) equally frustrating. The role of EOG in sleep staging is further discussed in Chapter 3.
FIGURE 1.1 A 30-second epoch from the overnight polysomnogram of a 46-year-old man with a long-standing history of snoring, witnessed apneas, and excessive daytime sleepiness. Note the occurrence of slow lateral eye movements in the electro-oculogram (EOG) channels, E1-M1 and E2-M1. A posterior dominant rhythm is still present in more than half this epoch, which is therefore still scored as stage W. Top eight channels, Electroencephalographic recording with electrodes placed according to the 10-20 international electrode placement system; Chin1-Chin2, submental electromyogram (EMG); ECG, electrocardiogram; HR, heart rate; INTRC, intercostal EMG; LtTib, RtTib, left and right tibialis anterior EMG; LGAST, RGAST, left and right gastrocnemius EMG; OroNs1-OroNS2, oronasal airflow; PFLOW, nasal pressure transducer recording; Chest and ABD, effort belts; Sao2, arterial oxygen saturation by finger oximetry. Also included is a snore channel.
FIGURE 1.2 A 60-second epoch from the overnight polysomnogram of a 35-year-old man complaining of excessive daytime sleepiness and with a prior diagnosis of obstructive sleep apnea. He also has a history of depression and is on citalopram, a selective serotonin reuptake inhibitor (SSRI). This epoch represents stage N2 sleep, as evidenced by the presence of K complexes and sleep spindles. Note the presence of excessive eye movements, representing a combination of slow and rapid eye movements. Eye movements generally do not persist into stage N2 and beyond but are often seen in patients on SSRIs (colloquially referred to as Prozac eyes). Top eight channels, Electroencephalographic recording with electrodes placed according to the 10-20 international electrode placement system; Chin1-Chin2, submental electromyogram (EMG); ECG, electrocardiogram; HR; heart rate; LtTib, RtTib, left and right tibialis anterior EMG; LGAST, RGAST, left and right gastrocnemius EMG; OroNs1-OroNS2, oronasal airflow; PFLOW, nasal pressure transducer recording; Chest and ABD, effort belts; Sao2, arterial oxygen saturation by finger oximetry. Also included is a snore channel.
Electromyography Recording During Standard Polysomnography
EMG channels provide important physiological characteristics that help determine sleep stage, as well as aiding in the diagnosis and classification of a variety of parasomnias. At a minimum, a PSG consists of chin EMG channels recording activity from the mentalis and submental muscles (the mylohyoid and anterior belly of the digastric) and bilateral leg EMG channels recording activity from the tibialis anterior muscles. EMG is recorded using a gold cup or a silver–silver chloride electrode applied to a clean surface using a tape or electrode glue. For chin EMG recordings at least three EMG electrodes are applied so that in the event of a problem with one of the electrodes the additional electrodes can be connected during the recording without disturbing the patient. The electrode impedance should be less than 5000 ohms. The high- and low-frequency filter settings for the EMG recordings are different from those used for EEG and EOG and are listed in Table 1.1. The sensitivity should be at least 20 μV/mm for mental or submental EMG activity.
Many patients with a history of abnormal movements or behavior in sleep require a more extended EMG montage, known as a multiple muscle montage, which includes extra channels that record from additional cranially innervated muscles (such as the sternocleidomastoideus, masseter, and mentalis), upper limb muscles (e.g., biceps, triceps, extensor digitorum communis), lower limb muscles (e.g., quadriceps, gastrocnemius), and axial muscles (e.g., paraspinals, rectus abdominis, intercostals) (Table 1.4; see also Fig. 9.4). This is of particular utility in patients with suspected RBD, in whom REM without atonia may be missed if an adequate number of muscles is not sampled. Although a standard montage for RBD has not yet been agreed upon, Frauscher et al found that simultaneous recording and quantitative analysis of the mentalis and flexor digitorum superficialis in 3-second miniepochs was 100% specific for RBD, when activity was present in more than 31.9% of miniepochs. The heterogeneity of RBD appears to be expressed in the dissociated EMG findings in muscles innervated by the cranial nerves, as well as the arms and the legs, requiring recording from multiple muscles. Multiple muscle montage recording may also be useful in patients with suspected RLS, because PLMS may also occur in the arm muscles or, rarely, in the axial or cranially innervated muscles (see Fig. 9.3).
Table 1.4
Multiple Muscle Montage (Extended Electromyographic Channels for Parasomnias and Other Movement Disorders in Sleep)
Channel Number | Name |
1 | F3-M2 |
2 | C3-M2 |
3 | O1-M2 |
4 | F4-M1 |
5 | C4-M1 |
6 | O2-M1 |
7 | Left electro-oculogram (E1-M1) |
8 | Right electro-oculogram (E2-M1) |
9 | Chin EMG |
10 | Right masseter EMG |
11 | Right sternomastoid EMG |
12 | Left biceps brachii EMG |
13 | Left triceps EMG |
14 | Right biceps brachii EMG |
15 | Right triceps EMG |
16 | Right lower rectus abdominis EMG |
17 | Right lumbar paraspinals EMG |
18 | Left quadriceps EMG |
19 | Left hamstrings EMG |
20 | Right quadriceps EMG |
21 | Right hamstrings EMG |
22 | Left gastrocnemius EMG |
23 | Left tibialis anterior EMG |
24 | Right gastrocnemius EMG |
25 | Right tibialis anterior EMG |
26 | Intercostal EMG |
27 | Oronasal thermistor∗ |
28 | Nasal pressure transducer∗ |
29 | Chest |
30 | Abdomen |
31 | Snoring |
32 | Arterial oxygen saturation |
33 | ECG |
34 | Heart rate |
ECG, Electrocardiogram; EMG, electromyogram.
Channels 1 to 6 record electroencephalographic activity from bilateral cerebral hemispheres with referential parasagittal chains. Channels 9 to 26 record EMG activity, including from additional muscles not routinely studied on typical polysomnograms. Channels 27 and 28 record airflow (“flow channels”). Channels 29 and 30 record respiratory effort (“effort channels”) (see also Fig. 9.4).
∗In a continuous positive airway pressure (CPAP) titration study, flow channels are replaced by a CPAP signal (C-flow signal).
Additional EMG channels aid in the analysis of unusual movements in sleep, especially myoclonus, and help assess their propagation and thus their generators. A dystonic muscle burst refers to prolonged EMG activity of 500 to 1000 milliseconds or longer (see Fig. 9.6). Myoclonic muscle bursts are also phasic bursts, which are characteristically noted during REM sleep and may be seen as excessive fragmentary myoclonus also during non-REM (NREM) sleep in many sleep disorders. Myoclonic bursts refer to EMG activity lasting for a brief duration of generally 20 to 250 milliseconds. In patients with tremor, EMG may record rhythmical activity in agonist-antagonist muscle pairs.
It is often helpful to also include intercostal and diaphragmatic EMG channels to record respiratory muscle activity. The intercostal EMG recorded from the seventh to ninth intercostal space with active electrodes on the anterior axillary line and the reference electrodes on the midaxillary line may also include some diaphragmatic muscle activity in addition to the intercostal activity. Diaphragmatic activity can be recorded by placing surface electrodes over the right or left side of the umbilicus or over the anterior costal margin, but these are contaminated by a mixture of intercostal activity and such noninvasive techniques are unreliable for quantitative assessment of diaphragmatic EMG. True diaphragmatic activity is typically recorded by intraesophageal recording. Intercostal and diaphragmatic EMG is particularly useful in the differentiation between central and obstructive apneas, especially when the respiratory channels are unreliable; continued bursts of activity in these channels during such an event would identify it as obstructive, whereas the absence of such bursts would implicate a central event (Fig. 1.3). The 2007 AASM Manual for the Scoring of Sleep and Associated Events recommends the use of the intercostal and diaphragmatic EMG channels for scoring apneas/hypopneas when the airflow channels are unreliable.
FIGURE 1.3 A representative 90-second epoch from the overnight polysomnogram (PSG) of a 46-year-old man with a long-standing history of snoring, witnessed apneas, and excessive daytime sleepiness. Three respiratory events are depicted in this epoch. Note that during the second event (B) the chest lead shows artifacts making it difficult to determine whether it is central or obstructive in nature. However, the intercostal EMG channel (INTRC) shows the absence of inspiratory bursts during the event, confirming that it is a central apnea. Also noted is cardioballistic artifact in the abdomen (ABD) lead, another indicator of a central event. For comparison, the first and third events (A and C) are clearly obstructive apneas, and intercostal EMG bursts continue (albeit at a lower amplitude) throughout their duration. The intercostal EMG channel is often very helpful in distinguishing between obstructive and central apneas when effort channels are unreliable or show artifacts. Top eight channels, Electroencephalographic recording with electrodes placed according to the 10-20 international electrode placement system; Chin1-Chin2, submental electromyogram (EMG); ECG, electrocardiogram; HR, heart rate; LtTib, RtTib, left and right tibialis anterior EMG; LGAST, RGAST, left and right gastrocnemius EMG; OroNs1-OroNs2, oronasal airflow; PFLOW, nasal pressure transducer recording; Sao2, arterial oxygen saturation by finger oximetry. Also included is a snore channel.
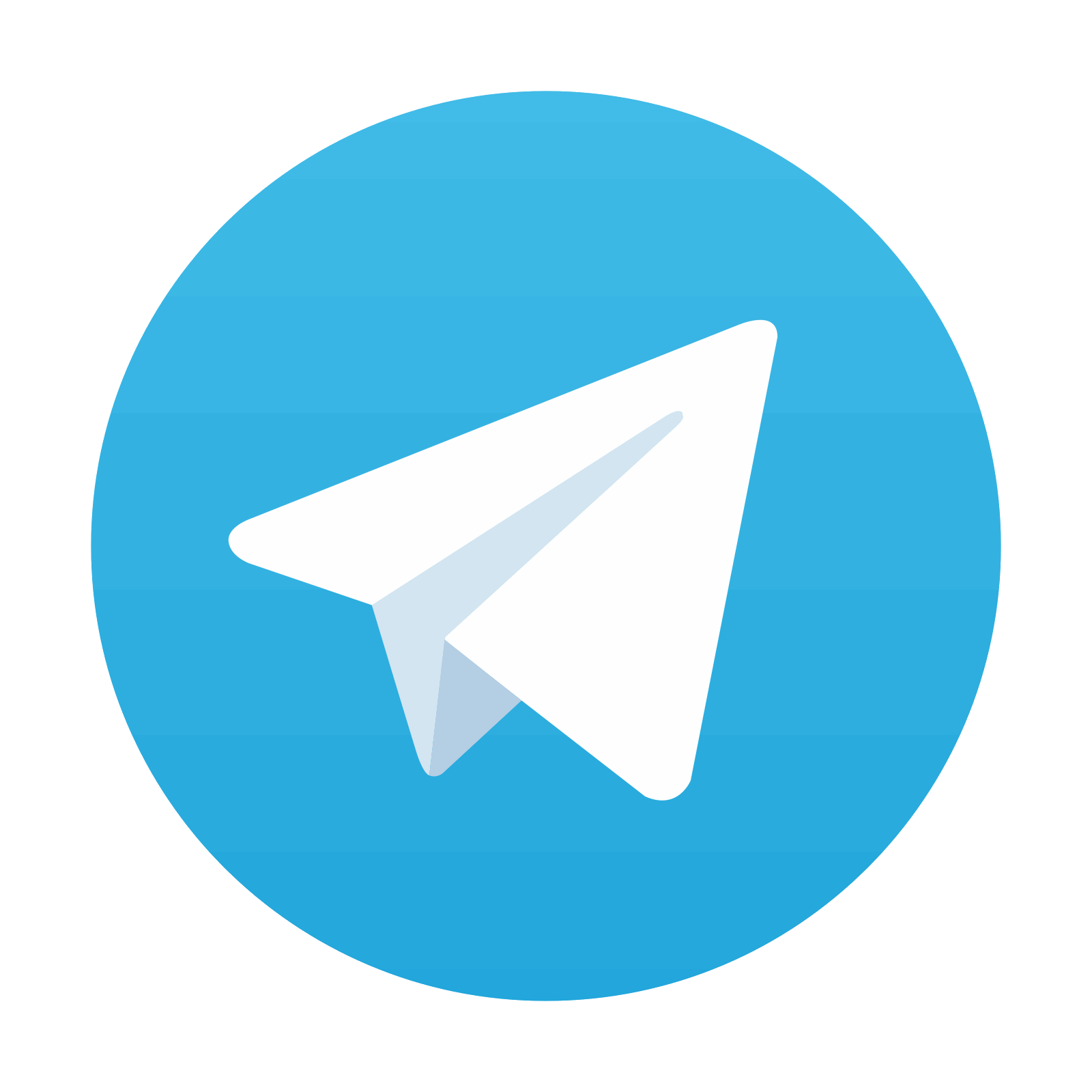
Stay updated, free articles. Join our Telegram channel

Full access? Get Clinical Tree
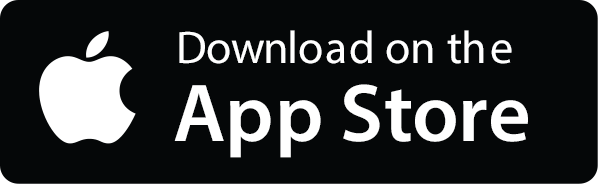
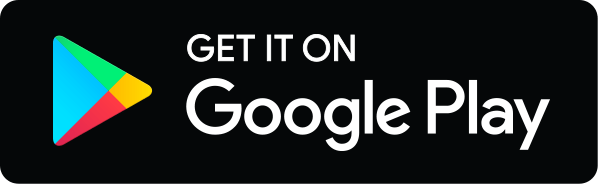