(1)
Department of Neurology, Atkinson Morley Wing, St George’s University Hospital, London, UK
(2)
Department of Ageing and Health, Guy’s and St Thomas’ NHS Foundation Trust, London, UK
Abstract
Cognitive impairment is common after stroke and its recognition is crucial, as it impacts on rehabilitation. Deficits may affect specific cognitive domains such as language or may be more global. In this chapter, we review the literature studying the neuroanatomy and the clinical presentation of major types of cognitive deficit, and discuss which neuropsychological tests are appropriate for each cognitive domain. Pharmacological treatment options are limited, but several studies are analysing the effects of secondary stroke prevention and specific biological products and their role in preventing, altering, or reducing cognitive decline.
Keywords
Cognitive impairmentAphasiaDeliriumExecutive functionAttentionKey Messages
Commonly used screening tools may miss impairments other than memory problems.
Post-stroke delirium can remain in up to 30 % of patients and is associated with increased risk of institutionalisation and mortality.
Executive function problems are a hallmark of cerebral small vessel disease and should be evaluated using appropriate tests, such as the trail-making test.
Trials are ongoing studying the benefits of controlling risk factors for stroke on cognitive impairment.
Introduction
Post-stroke cognitive impairment falls under the umbrella term of vascular cognitive impairment, encompassing all forms of cognitive loss associated with cerebrovascular disease and ischaemic brain injury. Cognitive impairment related to stroke may be in association with cortical and/or subcortical haemorrhage or infarction, strategic haemorrhage or infarction, that produces an abrupt onset of cognitive impairment often without additional focal neurologic deficit and/or “silent” haemorrhage or infarction (without overt clinical decline at the time). A number of different domains of cognitive function may be affected, often depending on the structural location of the stroke. These include memory, language, orientation, attention, and executive function. Whilst the site, size, and depth of the lesion will impact significantly on the cognitive effects of the stroke, “distance effects” of diaschisis and disconnection syndromes will also be contributory. Diaschisis refers to the depression of activity in areas of the brain distant to the stroke, often confined to relatively discrete functions, and is supported by reduced blood flow and EEG abnormalities being demonstrated in non-infarcted brain areas. In younger individuals these effects commonly recover, but in older patients chronic diaschisis may result in a disconnection syndrome involving the loss of brain function by an intact area of brain at some distance from the stroke.
Epidemiology
Data from 1,618 patients of the South London Stroke Register suggest that the prevalence of cognitive impairment 3 months after a stroke is 22 %, as defined by abnormal mini-mental state examination (MMSE) or abbreviated mental test (AMT) scores [1]. After 5 years, 22 % of patients followed up remained impaired, and after 14 years, 21 % were affected. The prevalence was higher in patients with total anterior circulation strokes (50 %) but there was a stepwise deterioration in patients with lacunar stroke and small-vessel disease (SVD) [1]. In a Singaporean population of 252 patients with a transient ischaemic attack (TIA) or non-disabling ischaemic stroke, within 6 months of the event, only 56 % of patients were “cognitively intact,” 40 % were “cognitively impaired but not demented,” and 4 % were “demented” (using DSM [Diagnostic and Statistical Manual of Mental Disorders]-IV criteria). At 1-year follow-up, 31 % of those who were “cognitively impaired but not demented” improved to “cognitively intact”, 10 % of the “cognitively intact” group deteriorated to “cognitively impaired but not demented,” and 11 % deteriorated from “cognitively impaired but not demented” to “demented” [2]. Longitudinal observational studies such as these are limited by the lack of comparison with a non-stroke population under similar circumstances, the inclusion of a heterogeneous stroke population, and patients being lost to follow-up. Losing patients to follow-up introduces bias, as the severely disabled are more likely to drop out (from death or inability to attend), and therefore the actual prevalence at 5 years may be higher than that quoted. The aforementioned studies may also be limited by the use of cognitive assessment tools lacking sensitivity for the different cognitive domains affected after a stroke. More recently, the Secondary Prevention of Small Subcortical Strokes (SPS3) triallists undertook comprehensive neuropsychological evaluation of 1,636 patients within 6 months of suffering a lacunar stroke and found 47 % to have mild cognitive impairment (classified as z scores [converted from raw scores using published norms] being ≤−1.5 in memory and/or non-memory domains) with the largest deficits seen on tests of episodic memory, verbal fluency, and motor dexterity. Younger age (odds ratio [OR] per 10-year increase: 0.87), male sex (OR: 1.3), less education (OR: 0.13–0.66 for higher education levels compared to 0–4 years education), post-stroke disability (OR: 1.4) and impaired activities of daily living (OR: 1.8) were independently associated with mild cognitive impairment [3].
Rabadi et al. reported that post-stroke patients with cognitive impairment had more severe strokes with greater disability and delayed admission into rehabilitation compared with cognitively intact patients. However, the authors demonstrated that patients with cognitive impairment had significant improvements in their functional scores with rehabilitation [4]. Zinn et al. showed post-stroke cognitive impairment to be associated with poorer recovery to independence of activities of daily living despite similar rehabilitation opportunities [5]. In a Belgian study of 532 stroke patients, cognitive impairment, in addition to atrial fibrillation, age, and diabetes, was an independent predictor of mortality [6].
Pathophysiology
Vascular cognitive impairment is heterogeneous, and the pathophysiology of post-stroke cognitive impairment is unlikely to be explained by a single process. The supratentorial brain exhibits a number of distinct patterns of vascular supply, each particular to a peculiar zone, offering relative protection from and vulnerability to circulatory changes [7]. The basal ganglia and thalamus are supplied by long arterioles and muscular arteries from adjacent sources at the base of the brain; the cerebral cortex and corpus callosum (excluding the splenium) are supplied by short arterioles; and the subcortical association bundles or U-fibres are supplied by the terminal twigs of the longest cortical arterioles and by the earliest branches of the long medullary arteries and arterioles. For a given sector of the U-fibres, these two types of afferent vessels usually arise from different points on the brain surface, constituting a dual supply, and in the immediate subcortical region their terminal arterioles often appear to interdigitate. The external capsule area is supplied by the same two types of vessels as in the U-fibre area and, in addition, by lateral rami of the lateral striate arteries, thus constituting a triple blood supply. The terminal arteriolar territories of these three sources have also been shown to interdigitate [7]. The centrum semiovale is supplied by long, penetrating arteries and arterioles (20–50 mm in length and 100–200 μm in original diameter) originating from the pial network located on the brain surface. These penetrating vessels arise, close to each other, at right angles from the subarachnoid vessels, run through the cortical layers perpendicular to the brain surface and enter the white matter along the course of myelinated fibres [8]. The vessels penetrate to different depths, with the longest converging centripetally toward the angles of the lateral ventricles. These carrying vessels do not arborise but give off perpendicularly oriented short branches that irrigate the white matter, each of which provides the blood supply to a cylindrically shaped metabolic unit [9].
In addition to the aforementioned centripetal white matter blood supply, van den Bergh described cerebral arteries originating from choroidal and striate arteries which, after travelling toward the frontal horn, body, and posterior horn of the lateral ventricle and reaching a subependymal location, turned back into the white matter away from the ventricle, thus delineating a centrifugal or ventriculofugal supply (i.e. away from the centre of the brain or away from the ventricle). In this white matter region between the cortical and ventricular surfaces, ventriculopetal and ventriculofugal arteries have been said to form “a three-dimensional border area between the centripetal network surging from the periphery and a centrifugal network, dependent from well-defined branches”. [10]. Anastomoses between these networks are either scarce or absent [8] and this pattern of vascularisation has led to the theory that the subcortical white matter harbours an arterial border zone, or watershed, that is particularly susceptible to being injured as a result of systemic or focal decreases in cerebral blood flow [11].
The subcortical white matter may therefore be considered a distal irrigation field prone to injury in the face of impaired blood flow. In contrast to the cerebral territories described with interdigitating blood vessels providing dual or triple blood supply, the centrum semiovale, basal ganglia, and thalamus have no such interdigitating supply and are more vulnerable to ischaemia and are the most common sites for lacunar infarcts [12]. Furthermore, the supplying blood vessels themselves are subject to the changes of arteriosclerosis and the arrangement of white matter metabolic units is such that, although anastomoses do exist at the precapillary level, one distributing vessel irrigates only one metabolic unit [9].
Cerebral Small Vessel Disease
Cerebral arterioles and small penetrating arteries supplying subcortical structures, both of diameter less than 400 μm, undergo physiological, age-related, and arteriosclerotic changes, which may then be accelerated by disease states such as chronic hypertension and diabetes mellitus. The changes in the blood vessels include intimal atheroma formation, medial smooth muscle hypertrophy, and subsequent hyaline deposition in the walls [13]. Consequently, the vessel wall becomes rigid and the lumen becomes narrow, impeding blood flow. In addition, there is a general tendency to increasing tortuosity of cerebral arterioles with ageing, compounding any reduction in blood flow [14].
Reduced blood flow or complete occlusion of a single small artery (due to local arterial wall pathology or emboli) may produce necrosis in an artery’s territory of supply, with destruction of all of the cellular elements and subsequent removal of the necrotic tissue by a standard inflammatory response [13]. Such lacunar infarctions, whose size range from 1 to 20 mm in diameter, account for a quarter of ischaemic strokes and are the most common abnormality found in human brains at post-mortem examination [15].
Lipohyalinosis and Microatheromatosis
In autopsy studies, two types of vascular pathology underlying occlusion of perforating arteries causing lacunar infarction have been distinguished: lipohyalinosis and microatheromatosis [16, 17]. Lipohyalinosis refers to a continuum of arteriolar alterations, from the earliest thickening of the vessel wall to progressive narrowing, post-stenotic dilatation, thrombosis, and fibrinoid necrosis, and was present mainly in patients with small, multiple, asymptomatic lacunes. Microscopically, the muscle and elastic laminae are replaced by collagen with a generalised increase in subintimal hyaline material. As a result of the histopathological continuum also including microaneurysm formation in association with impaired vessel wall integrity, this same lipohyalinotic pathology has also been implicated to be the driving force behind deep brain haemorrhage of subcortical origin. In contrast, microatheromatosis refers to a small atheromatous plaque narrowing or occluding a small artery proximally at its orifice and was found mainly in patients with single, larger lacunes [17].
Leukoaraiosis
Many ischaemic episodes are insufficiently prolonged to produce true infarction but may damage vulnerable tissue elements. Different cell types have varying degrees of vulnerability to ischaemia, with neurons being more vulnerable than oligodendrocytes, which themselves are more vulnerable than astrocytes [13]. Individual cells may undergo apoptosis involving the generation and sensation of signals that continued cell survival is no longer advantageous, the transduction of these signals by activation of a cascade of proteases, and subsequent cleaving of the genomic DNA into small fragments, thus terminating cellular viability. In this way, cells are removed without an accompanying inflammatory response and the rest of the tissue remains intact [18]. However, many combinations of neuronal and glial damage, that are intermediate between the extremes of focal neuronal loss and infarction, occur producing varying degrees of loss of myelin and axons, reactive astrocytosis, and tissue rarefaction falling short of cavitation [19]. The radiological correlate of these pathological changes is leukoaraiosis demonstrated by hypointensities on CT scanning or hyperintensities on T2 weighted and FLAIR MRI scanning. Quantitative perfusion MRI studies have shown that cerebral blood flow is reduced in the periventricular areas in patients with leukoaraiosis, and the degree of hypoperfusion correlates with the severity of leukoaraiosis [20].
Leukoaraiosis has been associated with impaired blood-brain barrier function, with endothelial cell retraction, increased vascular permeability, and greater susceptibility to white matter injury for relatively small insults [21]. One study has demonstrated intravenously injected contrast agent to leak into the brain, particularly in the territory of the perforating arteries, more in those with white matter hyperintensities on brain MRI than in controls [22]. Iwata et al. observed a small area of enhancement in the thalamus on contrast MRI 24 h before the development of a lacunar infarct at that location, leading the authors to suggest that breakdown of the blood-brain barrier may be the initiating event in lacunar infarction [23].
Cerebral Large-Vessel Disease
Large-vessel disease appears to be the fundamental process underling the aetiology of cortical ischaemic stroke. Atheroma mainly affects the large (aortic arch) and medium-sized arteries (carotid bifurcation, vertebral artery origins, and the basilar artery)—with hypertension, diabetes mellitus, hyperlipidaemia, and cigarette smoking being the major risk factors for its development. Atheromatous plaques consist of early deposition of lipid in the artery wall, buildup of fibrous material, necrosis, inflammatory cell infiltration, and calcification with subsequent haemorrhage leading to ulceration and platelet-fibrin thrombus formation on the plaque surface. Atherosclerotic plaques are in a dynamic process, becoming active and unstable or remaining dormant. It is unclear what mechanism is involved in mediating the plaque instability and subsequent rupture, but an exogenous antigen such as low-grade infection or an inflammatory response may be important. Thrombus on the plaque surface may become incorporated into the plaque with subsequent endothelialisation, obstruct the arterial lumen, be lysed by natural fibrinolytic mechanisms, and/or embolise to occlude a distal artery. Emboli consist of a combination of platelet aggregates, cholesterol debris, and fibrin. Hence, atherosclerosis may result in ischaemic stroke through insufficient blood flow caused by acute in situ thrombotic arterial occlusion, low flow distal to a severely narrowed or occluded artery, and embolism of atherosclerotic plaque or thrombus from a large vessel to occlude a smaller intracranial artery. Emboli may also arise from thrombi of cardiac origin as a result of atrial fibrillation, causing intra-atrial stasis and thrombus formation in the left atrial appendage, valvular heart disease, or a dyskinetic left ventricle due to ischaemic or non-ischaemic cardiomyopathy, causing thrombus formation in the left ventricle.
Endothelial Dysfunction
The normal cerebral endothelium plays a crucial role in the regulation of cerebral blood flow and the blood-brain barrier and, in addition, presents an anticoagulant phenotype to blood. However, under stimulation by numerous agents, the endothelium may be activated to undergo a number of changes including loss of vascular integrity, cytokine production, expression of leukocyte adhesion molecules, and a change in phenotype from antithrombotic to prothrombotic [24]. A number of separate lines of evidence suggest that chronic endothelial dysfunction plays an important role in the pathogenesis of cerebrovascular disease [25]. These include histopathological evidence of endothelial cell activation and retraction with increased vascular permeability and increased circulating levels of leukocyte adhesion molecules (shed from the surface of activated endothelial cells) and markers of coagulation activation (including thrombin-antithrombin complex and prothrombin fragments1+2) in patients with cerebrovascular disease compared with controls [25–28].
Cerebral Amyloid Angiopathy
Cerebral amyloid angiopathy is characterised by the deposition of amyloid Β-protein in the walls of the leptomeningeal cerebral vessels [29] and can lead to intracerebral haemorrhage, after vessel wall rupture, and less commonly cortical microinfarcts [30]. Haemorrhages caused by amyloid angiopathy are typically lobar and at the border of grey and white matter of the cerebral hemispheres [31]. Initially the disease affects leptomeningeal and neocortical vessels, and then progresses to the hippocampus, entorhinal and cingulate cortex, amygdala, hypothalamus, and the cerebellum [32]. The main risk factors identified are advancing age and concomitant Alzheimer’s disease [33].
Post-Stroke Cognitive Assessment
Whilst neuroimaging assesses structural brain damage, neuropsychological examination provides precise and sensitive indices of a patient’s mental efficiency [34]. Cognitive tests are standardised techniques that yield quantifiable and reproducible results that are referable to the scores of normal persons of an age and demographic background similar to those of the individual being tested [35]. Table 12.1 lists some of the cognitive tasks used in clinical practice. The most ideal test battery for cognitive impairment should be as short as possible to reduce the burden on the patient, but should be sensitive at detecting the common cognitive domains known to be affected in the disease process. A screening tool should be something that can be used in a typical clinic, whereas more precise cognitive batteries can be used by neuropsychologists to assess impairment in more detail. Most dementias are due to progressive neurodegenerative processes and therefore the cognitive tests should be sensitive to change over a defined period.
Table 12.1
Cognitive tasks description
Cognitive domain | Test | Description |
---|---|---|
Memory | Five-item recall/repetition | Recall five items told at different points |
Digit span forwards | Repeat a string of numbers | |
Digit span backwards | Repeat a string of numbers backwards | |
Executive function | Trail making | Patient asked to join alternate letter and number sequences (e.g. 1-A-2-B) as fast as possible |
FAS verbal fluency | Say as many numbers beginning with F then A then S in 1 min each | |
Wisconsin card sorting test | Participant is told to match the cards, but not how to match | |
Digit symbol | Match the symbol to the digits (e.g. 1 with ρ) | |
Letter number sequencing | A task that requires the reordering of an initially unordered set of letters and numbers | |
Attention/orientation | Stroop test | Show a sequence of names of colours written in a different colour. Ask patient to say the written colour not the actual colour |
Serial 7s | Subtract 7 from 100, 93, 86, 79, and 72 | |
Recite months of the year backwards | December through to January | |
Aphasia | Frenchay Aphasia Screening Test (FAST) | Test of comprehension (e.g. pointing at objects in a scene, verbal expression [describe the scene]), reading, and writing |
Ullevaal Aphasia Screening Test (UAST) | Expression, comprehension, repetition, reading, reproduction of a string of words, writing, and free communication |
National Institute for Health and Care Excellence (NICE) quality standards state that all stroke patients should be screened within 6 weeks for cognitive impairment using a validated tool [36]. This should then allow for the best treatment for the individual patient. In general, simple tests that elicit discrete responses are valuable in determining focal brain damage. On the other hand, multidimensional tests, being dependent upon several aspects of cerebral function, tend to be non-specific but are very sensitive to changes in general intellect and mental efficiency [34]. The MMSE (range 0–30) was designed to be a screening tool for dementia and has been used widely as a cognitive assessment test in a variety of clinical genres, including stroke. It is short and easy to administer, which has made it popular worldwide. It covers temporal and spatial orientation, memory, attention, language and visuospatial assessment with a maximum score of 30° points. A cut of score of 24/30 provides a reliable score for diagnosing dementia with high specificity and sensitivity [37]. However, evidence suggests that the MMSE has many limitations as it misses mild cognitive impairment and does not test for executive dysfunction. It also cannot detect changes in the severely impaired patients towards the end stages of disease. It is also criticised for repetition bias in mild cognitive impairment [38]. As a test of global cognitive dysfunction in stroke patients, the MMSE has been somewhat superceded by the Montreal Cognitive Assessment (MOCA). This also has a range of 0–30, but has been shown to be superior to the MMSE as a cognitive assessment tool in patients with cerebrovascular disease and, as such, is recommended in current guidelines [39–42]. In one study, the MOCA identified cognitive impairment in 67 % of stroke patients and 48 % of TIA patients with normal MMSE scores [43]. More recently, however, the MOCA has been shown to lack accuracy in post-stroke patients with cognitive problems not affecting memory [43].
In patients with subcortical infarction and cerebral SVD, in whom episodic memory is typically preserved, O’Sullivan et al. designed a clinical battery which took 20 min to administer [44] and had a high sensitivity and specificity. This battery included the digit symbol, FAS verbal fluency, digit span backwards, and trail-making tests. It was effective for determining deficits in patients with SVD and discriminating them from the normal ageing population, and it showed that bedside cognitive screening is possible in SVD [44]. Recently, the Brief Memory and Executive Test battery (B-MET) has been designed, which lasts 10 min and in a small group (n = 45), had a sensitivity of 91 % and specificity of 85 % for differentiating SVD patients with cognitive impairment from patients with Alzheimer’s disease. As a comparison, the MMSE had lower sensitivity (63 %) and specificity (62 %) [45]. The B-MET includes the five-item repetition, letter-number sequencing, five-item recall, and five-item recognition tests and an awareness assessment, analysing the patient’s awareness of how well they performed. The B-MET has a short assessment for memory and orientation but increased assessment for executive function and information processing speed. Therefore it may miss impairment in memory while identifying more executive dysfunction.
The Birmingham Cognitive Screen (BCoS) is a screening test designed to assess different forms of praxis in patients with spatial neglect and aphasia [46]. Because many patients with stroke have neglect and aphasia, it is a useful test to identify such higher cognitive deficits. It is a short screen including the Florida Apraxia Screening Test for pantomime, gesture recognition test, movement imitation test, and multiple object use test, and in conjunction with tests of activities of daily living, it allows one to review the effect of the apraxia on their daily functioning. A very well-known test used for decades to assess visuospatial and executive functioning and praxis is the Clock Drawing Test. It is quick to administer and as a screening tool can be valuable to identify hemi-neglect, apraxia, and episodic memory [47]. It may predict future decline in cognition, although more data is required to confirm this.
Post-stroke cognitive impairment may sometimes be clinically silent, but relatives and carers may report abnormal behaviour resulting from lack of strategic planning, reduced speed of cognitive processing, and personality changes including apathy and irritability. As such, assessment of behaviour and function may be crucial to ascertain the impact of cognitive dysfunction on patients’ ability to undertake complex, goal-directed, purposeful activities, which form part of daily life, such as cooking, dressing, shopping, and housework. Various scales have been derived to assess disability and have been verified in stroke. The most commonly used scales are the modified Rankin Scale [mRS] and Barthel index. Carers need to be educated about the impact of cognitive impairment and recognise that it can vary over time [48].
Cognitive Impairments After Stroke
Memory
Memory is the process in which information is encoded, stored, and retrieved. In simple terms, memory is divided into short-term memory (working memory) and long-term memory. Working memory, which refers to holding in and manipulating new information, is defined as memory duration of 15–30 s, and is closely associated with attention. An example is remembering a telephone number and may be assessed by the digit span test. The prefrontal cortex, which receives information from visual and auditory sensory cortices, as well as the hippocampus, plays an important role in working memory. Long-term memory is further divided into explicit and implicit memory. Explicit memory is divided into episodic memory (relating to events and experiences) and semantic memory (relating to facts and concepts). Long-term memory relies on the parahippocampal and perirhinal cortices. These brain regions receive information from the parietal and temporal cortex and then project to the entorhinal cortex and hippocampus. Synaptic changes in the hippocampus allow memory storage, which then reinstates the pattern of cortical activation, allowing learning. Damage to the hippocampus affects recent memories only as older memories are consolidated in the cortex. Implicit memory is the unconscious procedural memory, e.g. how to drive a car or emotional memory, e.g. fear of a known trigger. The neuroanatomy of implicit memory is not fully understood but is likely to involve a complex circuit. Lesional studies suggest that the hippocampus is not involved [49, 50].
Pure amnesic strokes are rare, as patients are likely to have focal neurologic deficits from their stroke. Akiguchi et al. described acute amnesic syndromes due to unilateral infarcts in 26 patients [51]. Eight had an anteromedial thalamic stroke and 18 had a medial temporal lobe plus hippocampal stroke. Prolonged recent memory loss was associated with new learning disabilities of verbal memory in left-sided lesions and non-verbal and visuospatial memory in right-sided lesions [51]. Careful neuropsychological testing may help identify patients with amnesia. Szabo et al. described hippocampal infarcts in 11 of 57 patients to be initially associated with prominent aphasia, but more detailed testing revealed more problems with verbal and non-verbal episodic memory loss [52].
Whilst the general screening tools (MMSE and/or MOCA) have been shown to be good screens for memory impairment, more detailed tests of memory exist. These include the Adenbrooke’s Cognitive Examination-Revised (ACE-R) [53], Test Your Memory [54], Raven’s Coloured Progressive Matrices, [55] and Sheffield Screening Test for Acquired Language Disorders [55]. Many patients can have transient amnesia immediately after the stroke such that testing of memory often improves with time.
Language
Dysphasia is the most common language disturbance caused by a stroke, affecting approximately one-third of patients. The most common types are expressive (affecting language formulation) and receptive (affecting comprehension and understanding). It is well accepted that expressive dysphasia is usually caused by a stroke in Broca’s area (inferior frontal gyrus) and receptive dysphasia is usually caused by a stroke in Wernicke’s area (superior temporal gyrus), both usually in the left hemisphere. Language is predominantly lateralised to the left hemisphere of the brain, with disconnection of left hemispheric brain regions having been shown to impair word generation in patients with cerebrovascular disease [21]. Other forms of dysphasia include transcortical sensory (where a patient has poor comprehension and naming with fluent spontaneous speech with paraphasias), anomic (inability to name objects), and conduction (fluent, paraphasic speech with poor speech repetition). Conduction dysphasia occurs when the connection between the Broca and Wernicke areas has been broken due to a lesion in the arcuate fasciculus. Dysphasia may also occur after damage to the insula or to deep brain structures such as the thalamus, again, as above, usually in the left hemisphere. Mutism is associated with large fronto-putaminal lesions [56].
Various language assessment scales have been used to assess the presence and degree of aphasia. The Frenchay Aphasia Screening Test (FAST) is the most popular and reliable screening test for patients with aphasia [57]. It has a specificity of 87 % and sensitivity of 80 %. The Ullevaal Aphasia Screening Test (UAST) has also been shown to be accurate for identifying aphasia with a sensitivity of 75 % and specificity of 90 %.
Orientation
Orientation forms a part of a global attentive process alongside concentration, exploration, and vigilance. It should be the beginning of all neurological and cognitive examination. Time orientation should include the year, season, month, date, and day of the week, while place orientation should include the building, floor, town, county, and country. These form the first section of the MMSE; however, only six of these ten items are included in the MOCA. Using the MMSE on 177 patients who had stroke, Desmond et al. [58] showed that 40.7 % of patients were disorientated 10 days after their stroke and 22 % remained disorientated at 3 months. The persistence of disorientation was associated with stroke severity, but not stroke location or subtype. Orientation has a significant impact on rehabilitation, with a reduction in Barthel Index scores, even after 6 months [59]. It affects activities of daily living and social function, and therefore limits recovery after a stroke.
Attention
Attention has been described as the foundation of other cognitive functions that allows an individual to select relevant information and filter out irrelevant information. Most studies have implicated the frontal and parietal lobes in the control of attention, more commonly in the right hemisphere. Post-stroke, attention is impaired in 46–92 % of patients, mainly due to neglect or inattention [60]. Deficits in attention impact on learning motor skills and are associated with falls and balance problems [60].
Hemispatial neglect is a special form of inattention when the patient is unaware of one side of their body or the world. This can be visual or sensory, but sensation and visual pathways are usually intact. Neglect is related to lesions in the temporo-parietal lobes. Most often the lesion is in the right hemisphere, as there is redundant processing of the right visual fields by both hemispheres. The right hemisphere can compensate for left hemisphere neglect, whereas the left cannot compensate for right hemisphere lesions. In the early phase of recovery, neglect impacts greatly on rehabilitation, with more severe neglect associated with less improvement in limb paresis [61].
There are various tests of attention such as serial 7s, digit span, spelling “WORLD” backwards and reciting the months of the year backwards. Of all these, the digit span test is regarded as a relatively pure test of attention but it is impaired in global and focal brain damage and, therefore, is not specific. The normal median (forward) digit span for an educated 60- to 74-year-old person is 8 ± 1 and over 75 years is 6 ± 1 [62].
Executive Function and Information Processing Speed
Executive function is a term used to describe complex cognitive processes required to achieve a particular goal. It enables planning complex tasks, switching attention, and selection of appropriate responses, and relies on working memory [63]. Information processing speed is a cognitive function involving the ability to perform fluently and effectively a relatively simple task automatically, and it relies on the skill to process information automatically and speedily without intentionally thinking through the task. Impairments of executive function and information processing result from cortical-subcortical and cortico-cortical disconnection, due to white matter tract disruption, compromising the integration of information from large-scale neural networks [64]. A number of distinct fibre systems have been described, and these include dorsolateral prefrontal-subcortical circuits mediating volition, planning, purposive action, and effective performance; orbitofrontal-subcortical circuits providing frontal inhibition of the limbic system, preventing impulsivity and uninhibited behaviour; and anterior cingulate-subcortical circuits whose interruption results in apathy and abulia [65].
Since executive function requires intact functioning of frontal cortices and their connections via association fibres and short U-fibres, and information processing speed is dependent on the integrity of subcortical neural circuits, they are both impaired early in cerebral SVD. In the Rotterdam Scan Study, whilst strategic lacunes in the thalamus were associated with memory impairment, non-thalamic lacunes were associated with the impairments of information processing speed [66]. The Leukoaraiosis and Disability study showed thalamic lacunar infarcts to correlate with motor speed and executive dysfunction, and lacunes in the putamen and pallidum correlated with memory and motor speed [67]. In patients with a clinical lacunar stroke, Benjamin et al. showed the number of lacunes and the volume of tissue destroyed by the lacunes to correlate with worse executive function and information processing speed and showed thalamic lacunes, especially, to be associated with impaired information processing speed [68].
Several neuropsychological tests have been used to assess subcortical executive cognitive deficits such as verbal fluency, trail making, digit symbol, and stroop tests, although in general, trail making and digit symbol tests are the most sensitive, and specific individual tests to identify cognitive impairment in people with mild cognitive impairment due to SVD who have normal MMSE scores [69].
Post-Stroke Delirium
Delirium is an acute and fluctuating confusional state characterised by altered consciousness, cognitive impairment, and perception. It can present as agitation associated with increased motor activity, or reduced motor activity and lethargy. It occurs in 13–48 % of stroke patients, although the aetiology is poorly understood [70].
Delirium is more common after an intracerebral haemorrhage and large hemispheric infarcts [71]. Patients with delirium have a poorer prognosis, with worse disability and increased chances of nursing home placement [72]. They also have a higher mortality and length of hospital stay [70]. Increased age and metabolic disturbances increase the risk of post-stroke delirium [73]. Whilst EEG studies have shown generalised cortical dysfunction, the pathogenesis of delirium is still unknown, although various neurotransmitter disturbances such as excess of dopamine or lack of cholinergic transmission have been suggested [74]. This theory is supported by studies showing that atypical antipsychotics, which act on D2 dopaminergic receptors, are effective in treating delirium [75]. Evidence for cholinergic medications is still lacking, however, but with the advent of biomarkers, pharmacological trials in this field are likely to increase [76].
There are no specific tools designed to diagnose delirium after stroke. The two most commonly used tools for diagnosing delirium are the Confusion Assessment Model (CAM) [77] and the Delirium Rating Scale (DRS) [78]. There have been no studies looking at the treatment of delirium specifically after a stroke. However, guidelines from the Royal College of Physicians outline basic measures, including treating the cause (e.g. infection), nursing in a well-lit room, and only using sedation sparingly [42]. If sedation is required, haloperidol is the drug of choice despite weak evidence of its superiority.
Managing Cognitive Impairment After Stroke
Non-pharmacological Therapies
Lifestyle modification has been associated with reduced cognitive decline in observational studies, and in a small randomised study (n = 38), aerobic exercise has been shown to improve post-stroke cognitive function and sensorimotor learning [79, 80]. Smoking cessation is also associated with improved cognition in normotensive patients [81]. There is, however, a paucity of randomised controlled trial evidence to suggest cognitive training activities benefit post-stroke cognitive impairment [82].
Memory Improvement
Only two trials assessing post-stroke interventions to improve memory were identified in a Cochrane review, with a total of 18 patients between them. Both provided intervention in groups rather than individual therapy. A trial on 12 patients randomised to six memory strategies versus pseudo-treatment “drill and practice” control group showed that, after 4 weeks, the treatment group improved significantly on the Name-Face Paired Associated Memory Test. There was no difference, however, in 15 Words, Oxford Recurring Faces, or Memory Questionnaire tests [83]. Another study using imagery-based mnemonics for 10 weeks showed an improvement in delayed recall of day-to-day events [84]. However, there was no improvement in memory function overall, and the positive results may have been due to short term learning effects.
Occupational therapists are predominantly involved in cognitive rehabilitation. In the absence of a strong randomised controlled trial evidence base of interventions to target post-stroke memory impairment, they teach simple techniques such as association (i.e. associate the new information with something one already knows), visualisation, repetition, rehearsal, and compensation (e.g. write it down) in a pragmatic fashion.
Language Therapy
Speech and language therapy is the mainstay of post-stroke language rehabilitation with treatment being individualised to maximise the patient’s ability to communicate via verbal, written, or non-verbal means. Whilst the ACTNOW study, including 170 post-stroke patients, showed 4 months of speech and language therapy did not have any benefit on speech recovery compared to regular communication [85, 86], a meta-analysis of 39 randomised controlled trials, involving 2,518 participants, demonstrated speech and language therapy to result in significant benefits to patients’ functional communication and receptive and expressive language [87]. More recently, promise has been shown for very early intensive rehabilitation of dysphasia post-stroke, with patients receiving twenty 1-hour rehabilitation sessions over 5 weeks after their stroke recovering better than patients who received standard therapy [88].
Technological advances may improve the outcome of speech and language therapy further. Transcranial magnetic stimulation (TMS) is a non-invasive technique that causes depolarization or hyperpolarization of a region of the brain via weak currents. A coil is placed against the scalp and a short pulse is applied through the skull. This stimulates the desired region of the brain, and repetitive TMS over Broca’s area has been shown to improve scores on language and mood scales in a small randomised controlled trial (n = 30) [89]. A computer-based software program called Fast Forword language has also been developed and has shown some improvement in comprehension scores compared with traditional one-to-one treatment over the same period of time [90]. Such techniques need to be tested on larger groups with different deficits to assess their use in clinical practice.
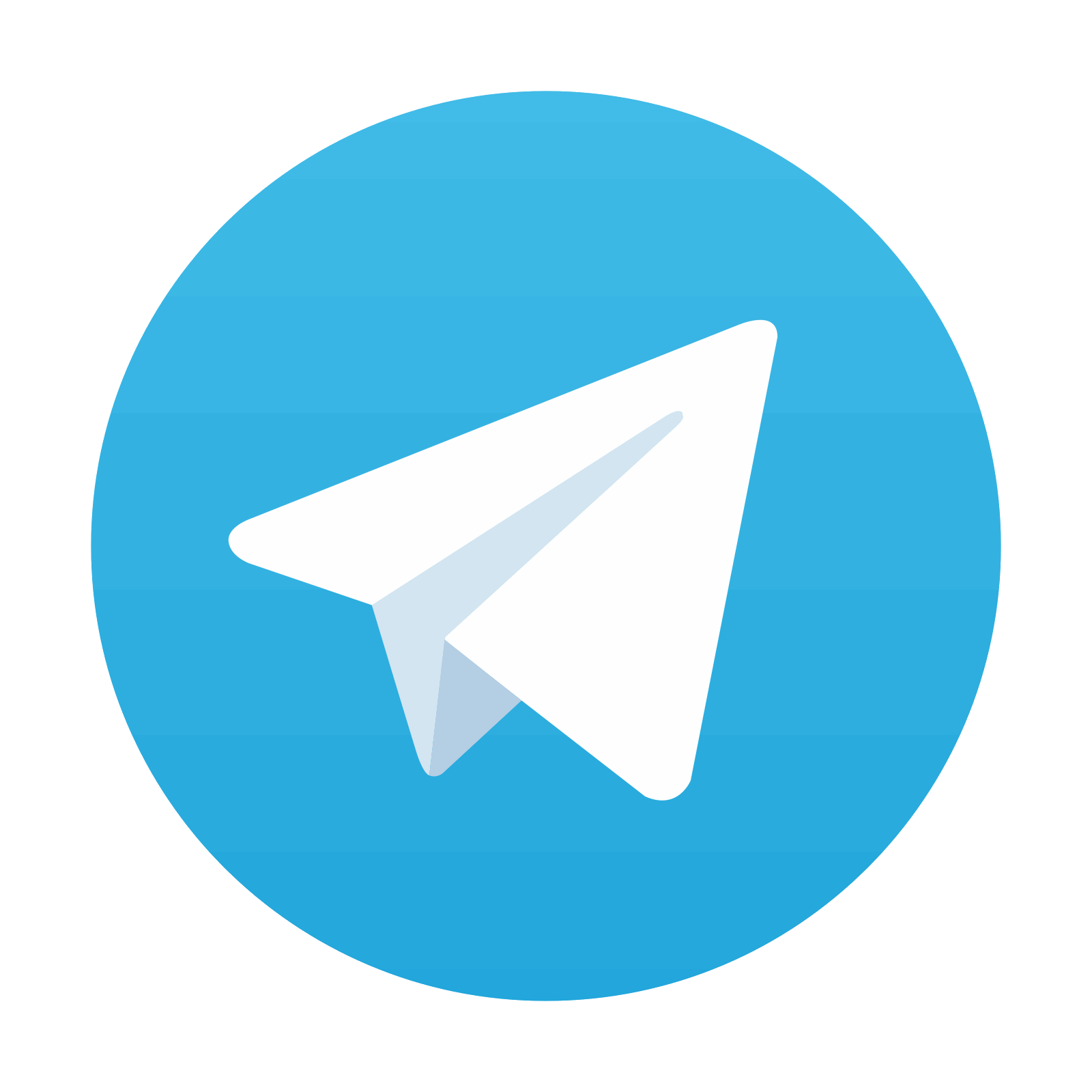
Stay updated, free articles. Join our Telegram channel

Full access? Get Clinical Tree
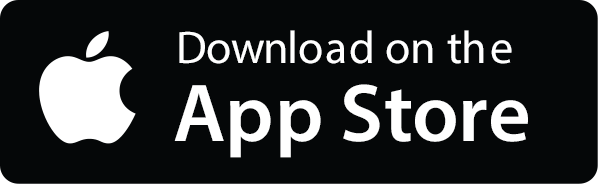
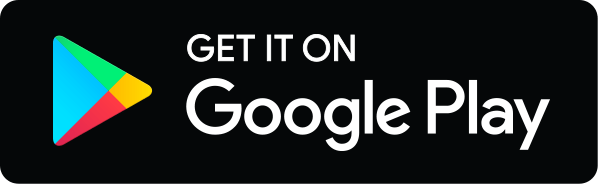
