Summary of Key Points
- •
Posterolateral fusion techniques are increasingly used in the treatment of degenerative thoracolumbar disease.
- •
Successful fusion is incumbent on the basic physiology of bone healing and the exploitation of appropriate autogeneic and allogeneic bone grafts.
- •
Posterolateral fusion surgery is accomplished via midline or paraspinal approaches and depends highly on the decortication of the fusion bed; this is true for facet joint, interlaminar, and intertransverse fusions.
- •
Known complications of posterolateral fusions include hemorrhage, infection, and pseudarthrosis.
Thoracic and lumbar spine fusions are typically performed to provide pain relief, preserve neurologic function, and maintain or restore spinal stability and alignment. Posterolateral thoracic and lumbar fusion procedures are performed for treatment of many spinal disorders. These disorders include traumatic, neoplastic, infectious, iatrogenic, and also degenerative conditions associated with deformity or instability. When surgical treatment of degenerative disorders is required, spinal fusion may be indicated following neurologic decompression in cases associated with spondylolisthesis or significant scoliosis. In a 1991 study of degenerative spondylolisthesis, Herkowitz and colleagues demonstrated better clinical and radiographic outcomes after decompression and noninstrumented fusion than after decompression alone. Spinal fusion may also be required in cases that result in significant iatrogenic instability secondary to extensive decompression or resection of sizeable lesions. However, lumbar spine fusion as a treatment of degenerative disc disease alone is generally not indicated. The addition of segmental instrumentation has been shown to increase fusion rates and improve clinical outcomes. Although internal fixation with instrumentation can be associated with complications, particularly in the setting of osteoporosis, it should be considered for patients who are candidates for spine fusion.
Historical Background
The technique for spinal arthrodesis was first described in the early 1900s. It was performed by Dr. Russell A. Hibbs in 1911 for spinal deformities and later by Dr. Fred H. Albee for tuberculosis. Albee created greenstick fractures of spinous processes in patients with Potts disease and used tibial autograft to augment the fusion. As familiarity grew with spinal fusion in the early 1920s, Mackenzie-Forbes and Hibbs introduced the technique of laminar decortication further extending the fusion laterally. In addition, Hibbs also introduced the concept of facet joint fusion in a series of his scoliosis patients, a technique that was further modified by Howorth, McBride, and Moe. Malvin B. Watkins first described the posterolateral fusion procedure for treatment of degenerative spine disorders in 1953. He described a paramedian approach to gain access to the transverse processes lateral to the paraspinous musculature, thus avoiding disruption of the spinous processes and interspinous ligaments. In addition, he described the utility of the posterolateral fusion in patients who had already undergone prior laminectomy at the site of surgery.
Since their inception, various techniques have been proposed for achieving posterolateral fusion. For example, as an alternative to posterior fusion, Mathieu and Demirleau introduced the intertransverse process fusion that proved beneficial for patients when laminectomy was indicated. Adkins subsequently implemented this technique in patients with spondylolisthesis. Wiltse and colleagues devised a muscle-splitting approach between the longissimus and multifidus muscles to gain access to the intertransverse process region. Furthermore, the introduction of spinal instrumentation with pedicle screw fixation further improved bony fusion rates. Thus, posterolateral fusions have been described classically as instrumented or noninstrumented approaches.
Biology of Bony Fusion
Spinal fusion is a dynamic biologic process that ultimately results in the surgical stabilization of spinal segments via bony union of two or more vertebrae. It depends highly on multiple factors, including the basic biology of bone healing, the selection of optimal graft material, the preparation of an appropriate milieu for healing, and various patient-specific concerns ranging from tobacco use to sufficient nutrition. Understanding the complex mechanisms that govern osseous fusion is a critical step toward decreasing rates of pseudarthrosis and improving surgical outcomes.
Posterior spinal fusion surgery is often performed to treat traumatic and degenerative spine pathology, such as spondylolisthesis or severe spinal deformity. Thus, it often used concurrently with neural element decompression and internal rigid fixation, both of which are associated with additional bony trauma during the procedure itself.
Osseous healing, along with the incorporation of any implanted bone graft materials, subsequently proceeds in a three-stage process similar to that seen during the healing of traumatic fractures. The first phase is hematoma formation at the surgical site that initiates the inflammatory phase of bone healing by recruiting various inflammatory cells and fibroblasts to the site of injury. Among these are macrophages, which may exist locally in both the bony periosteum and endosteum or may be recruited via injured mesenchymal cells and complement-mediated mechanisms. Once activated, macrophages infiltrate the exposed bony surfaces and remove the necrotic debris associated with the hematoma. Moreover, these and other inflammatory cells secrete additional growth factors (e.g., vascular endothelial growth factor, platelet-derived growth factor ) and inflammatory mediators that drive local angiogenesis, promote proliferation and differentiation of primitive mesenchymal cells into cells of osteoid or chondroid lineage, and induce the formation of granulation tissue at the fusion site over the fibrin scaffold provided by the hematoma. Presumably, exposure during this stage of bone healing to anti-inflammatory medications may significantly impact the critical signaling pathways that underlie this complex process.
The second phase of fusion, or reparative phase, involves the expansion of vascular-rich granulation tissue into a fibrous callus and immature bone. A cartilaginous matrix is deposited by chondroblasts while osteoid is secreted by osteoblasts forming the soft callus. As the defects in the bone are filled, bony graft material placed at the time of surgery may be integrated, in part, via mesenchymal osteoblastic activity. Subsequent mineralization leads to primary or woven bone formation at the site of fusion, and paves the way for the third phase of bony fusion known as the remodeling stage. In this final stage, woven bone segments are resorbed and remodeled in response to compressive and distractive forces at the fusion site. Interestingly, proper immobilization is critical at this stage of bone healing because the callus is typically not sufficiently robust to independently resist movement. Excessive disruption of healing bony surfaces leads to incomplete ossification and results in fibrous nonunion or pseudarthrosis. Ultimately, through a combination of osteoblastic and osteoclastic activity, the bony fusion is formalized. Over 3 to 6 months, areas of bones under stress are reinforced while inefficient bone is resorbed.
Bone Grafts in Posterolateral Thoracolumbar Fusions
Osseous healing in a spine fusion depends on a host of factors, including the preparation of an optimal fusion bed and the application of appropriate bone graft material. To achieve a solid arthrodesis, graft material may be selected in accordance with its osteogenic, osteoinductive, or osteoconductive capacities. Osteogenesis is dependent on the presence of osteoprogenitor cells within the graft capable of depositing extracellular matrix while balancing osteoclastic resorption and bone remodeling. In contrast, osteoinductive grafts actively recruit and promote mesenchymal stem cell differentiation into osteoblasts and chondroblasts. Occurring primarily in cancellous rather than cortical bone grafts, this process is mediated by graft-derived growth factors, relying heavily on bone morphogenic proteins (BMP)-2, -4, and -7. Finally, osteoconductive grafts provide a biologically appropriate scaffold for ingrowth of vascular and bone progenitor elements from the recipient host bed. As new bone is deposited, it is distributed evenly in accordance with the supportive ultrastructure provided by the graft. Interestingly, cortical bone grafts provide initial rigid support for the healing fusion mass, whereas cancellous bone is preferred for its comparatively larger surface area and its superior capacity for bony and vascular ingrowth.
The ideal graft should contain an ample number of viable osteoprogenitor cells, the growth factors necessary for osteoinduction, and the osteoconductive ability to create and support bone healing for graft incorporation. Autogenous bone grafts embody all these properties and have the additional benefit of being entirely antigenic. Moreover, these grafts often contain both cortical and cancellous components, providing both the strength of the former and the rapidly vascularized, osteoinductive ultrastructure of the latter. Autologous bone harvest, however, is associated with significant morbidity and is often limited in quantity. Thus, local bone autograft is increasingly used in posterolateral fusion surgery, obtained during concurrent decompression or from harvesting the bony facets. Depending on the harvest method, up to 35 cc of local bone autograft may be collected, providing sufficient graft material without the morbidity of harvesting bone from the iliac wing. When used in posterolateral fusions, local bone autograft may be as effective as iliac crest bone graft in achieving short segment lumbar fusion, but it may be further paired with bone graft extender to maximize the capacity to achieve bony union.
In the setting where autograft is unavailable or impractical, allogeneic grafts may provide the osteoconductive matrix necessary for fusion. However, having undergone sterilization and processing, allografts have limited osteoinductive properties. Tissue processing may also structurally weaken the graft, but it is necessary to address concerns over the possibility of disease transmission, ensure sterility, and reduce antigenicity. Nevertheless, allografts may evoke a significant host immune response that can critically impact bone graft incorporation. Although adequate fusion rates can be achieved with allograft when supplemented with segmental instrumentation in young patients with thoracolumbar scoliosis, thoracolumbar spine fusion rates are much higher with autograft than allograft alone. A higher pseudarthrosis rate has also been demonstrated with the use of allograft when compared with autograft for intertransverse and interlaminar fusion in the lumbar spine.
Efforts to improve the success of allograft, as well as synthetic graft, incorporation have led to the development of a variety of bone graft alternatives. Some of these function in an osteoconductive capacity, acting as scaffolds for bony healing. The more common osteoconductive materials include ceramics, synthetic polymers, such as polymethylmethacrylate and polyetheretherketone (PEEK), and natural polymers, such as collagen. Many osteoconductive materials are combined with osteoinductive bone graft substitutes, which contain the signaling molecules necessary to induce the formation of new bone. For example, demineralized bone matrix (DBM) supplies potent BMPs to the fusion bed on a collagenous osteoconductive substrate.
Similarly, high-dose recombinant human BMPs, particularly BMP-2 (rhBMP-2), have been used extensively to help stimulate bone synthesis in posterolateral fusions. In 2002, Boden and colleagues reported results of a pilot prospective trial of 25 single-level posterolateral fusion patients. They identified significantly higher fusion rates in those who were treated with rhBMP-2 than in the patients who received iliac crest autograft. This finding has been repeatedly replicated since then, most recently by Hurlbert and associates who reported in approximately 200 patients that fusion rates were 94% with rhBMP-2 versus 69% in patients who received iliac crest. The successful application of rhBMP-2 in posterolateral fusions has helped expand efforts to identify additional recombinant osteoinductors and has driven the development of novel cellular and genetic solutions to stimulate arthrodesis. At the time of this writing, the Food and Drug Administration (FDA) does not approve the use of rhBMP-2 for posterolateral fusion.
Surgical Technique
Position
Dorsal thoracic and lumbar fusions are usually performed with the patient positioned prone on either chest rolls or a spinal frame. Pressure points are padded to prevent skin breakdown. Special attention should be paid to avoid pressure on the patient’s eyes while in the prone position. The arms should be abducted less than 90 degrees, and positioned on arm boards with the elbows flexed and padded to prevent ulnar nerve injury. In cases of restricted shoulder range of motion and upper thoracic fusions, the arms may be tucked by the patient’s side. The hips should be positioned in extension to maximize the lumbar lordosis. However, if there are significant hip flexion contractures, the lower extremities should be positioned with the hips flexed. The pelvis and knees should be adequately padded, and pillows should be used to support the legs. Of note, in preparation for surgery in the prone position, sequential calf compression devices may be applied to prevent deep venous thrombosis, and urinary catheterization should be performed if expected operative time exceeds 2 to 3 hours.
Approaches to the Thoracolumbar Spine
Midline Approach
A midline approach may be used to expose the thoracic and lumbar spine for surgical fusion. The dissection is carried down through superficial fascia to reach the deep thoracolumbar fascia. Self-retaining retractors are placed to improve visualization. The spinous processes are palpated through the deep fascia, and the fascia is divided over the spinous processes. At the appropriate level, the dissection is advanced laterally in a subperiosteal plane, starting at the tip of each spinous process, with the use of a Cobb elevator. Bovie electrocautery is often used to cut the paraspinal musculature at its attachment to the spinous processes. As the dissection is advanced laterally and subperiosteally along the spinous process and the lamina, pars interarticularis, and medial portion of the facet joints are exposed. The facet capsule should be preserved until the levels of fusion are confirmed radiographically because disruption of the superior segment facet joint can be associated with instability and increased risk of adjacent segment degeneration. The lateral surface of the pars and facet joints are then cleared of their muscular attachments using electrocautery. Advancing the dissection further exposes the dorsal surface of the transverse processes lateral to the facet joint. As needed, bipolar cautery is used for the terminal branches of the lumbar segmental artery, located rostral, caudal, and lateral to the facet joints. The self-retaining retractors are advanced to maintain exposure of the transverse processes. In the thoracic spine, the transverse processes can be exposed by lateral and rostral extension of the subperiosteal exposure of the lamina. Exposure of the L5 transverse process and sacral ala requires elevation of the multifidus and sacrospinalis origin from the sacral ala and dorsal sacrum. The L5 transverse process, lateral surface of the facet joint, and sacral ala should be denuded of the soft tissue attachments and decorticated to provide an optimal fusion bed for lumbosacral fusion.
Paraspinal (Wiltse) Approach
In the paraspinal (Wiltse) approach, two paramedian incisions are made approximately 5 cm from the midline, just medial to the posterior superior iliac spine. The incision may be planned from the preoperative magnetic resonance imaging (MRI) images by measuring the distance of the plane of separation between the multifidus and longissimus muscles and the midline ( Fig. 79-1 ). Additionally, intraoperative fluoroscopy may be used to localize the incisions, which should overlie the radiographic position of the pedicles in the medial-lateral plane as seen via an oblique view down their central axis. The dissection is advanced to the deep dorsal fascia, where fascial incisions are curved medially at their caudal ends to provide adequate muscular retraction. The muscle fascia is then divided, with dissection directed medially to reach the transverse processes and lateral surface of the facet joints. Notably, the interval between the longissimus and the multifidus muscles can be separated by blunt finger dissection. The self-retaining retractors are placed deep to the fascia, and the transverse processes are exposed subperiosteally.

Preparation of Fusion Bed and Bone Grafting
Preparation of Interlaminar and Intertransverse Regions
The dorsal surface of the lamina, lateral surface of the facet joint, mamillary body, and lateral pars are cleared of soft tissues. The exposed area is decorticated by using either a rongeur or a high-speed bur. Graft material is then placed over the decorticated bony elements ( Fig. 79-2A ).

Facet Joint Fusion
In the thoracic spine, the inferior articular process is excised by using an osteotome, high-speed bur, or a Capner gouge to expose the facet joint and cartilaginous surface of the superior articular process. The cartilage is then removed to expose bleeding cancellous surface, and bone graft is placed on this exposed surface ( Fig. 79-2B ). Alternatively, in the lumbar spine, the facet joints are exposed by excising the facet capsule. Once exposed, the cartilage from the joint is removed with a narrow rongeur or a high-speed bur. The exposed bleeding joint surface may then be packed with cancellous bone chips to facilitate fusion across the joint (see Fig. 79-2A ).
Complications and Avoidance
Hemorrhage
Perforation of terminal branches of lumbar and thoracic segmental arteries during the exposure of the facet joints, lateral pars interarticularis, and transverse processes can lead to significant blood loss. These arteries are found deep to the paraspinal muscles at the superior and inferior aspects of the facet joints (rostral and caudal articular arteries) and on the dorsal surface of the transverse process (communicating artery) ( Fig. 79-3 ). Appropriate identification and cauterization of these vessels prior to dissection can significantly reduce bleeding. Venous bleeding in the lumbar spine can be avoided by careful positioning of the patient to prevent increased intra-abdominal pressure. This decreases pressure on the vena cava, thus reducing the pressure in the epidural and paravertebral venous plexus. Autologous blood donation and the use of cell savers can be considered in patients in whom excessive intraoperative blood loss is anticipated.

Loss of Lumbar Lordosis
Normal lumbar lordosis can be maintained intraoperatively by keeping the lower extremities in an extended position by limiting flexion at the hips.
Pressure and Traction Injuries
Pressure injuries to skin at the bony prominences can be avoided by proper padding of the bony prominences of the upper and lower extremities. Traction injury to the brachial plexus can be avoided by limiting shoulder abduction to less than 80 degrees. Pressure on the eyes should be avoided to prevent ocular injury. Elbows should be properly padded to prevent ulnar nerve compression.
Pseudarthrosis
Successful fusion is defined as the presence of continuous bridging trabeculae of bone between spinal segments. A successful fusion inherently requires the absence of motion between the segments and may relieve symptoms caused by mechanical instability. Failure of fusion at the surgical site at or after 1 year from the index surgery indicates a pseudarthrosis and needs further investigation into etiology and treatment. Failure of fusion may present with persistence of back pain, progression of deformity in scoliosis, or recurrence of symptoms in spondylolisthesis. Pseudarthrosis has been associated with worse clinical outcomes and is one of the leading causes for revision lumbar spine surgery. The incidence of pseudarthrosis is highest at the thoracolumbar and lumbosacral junctions.
Use of flexion/extension radiographs for diagnosis of pseudarthrosis is controversial and is affected by high interobserver variability. Thin-cut computed tomography (CT) scans are considered more accurate than plain radiographs in determining the integrity of the fusion. The presence of metallic artifact in instrumented fusion, however, decreases the sensitivity of CT scan as the diagnostic modality of choice. Thin-slice CT scan combined with a high index of clinical suspicion may be considered the most reliable diagnostic option. However, surgical exploration of the fusion mass is considered the most specific and sensitive test for diagnosis of pseudarthrosis.
A variety of factors are thought to be responsible for pseudarthrosis, including metabolic abnormalities, smoking, infection, and persistent motion at the fusion site. Smoking has been associated with increase in nonunion rates from 8% in nonsmokers to 40% in smokers. A 2014 study by Bydon and coworkers demonstrated that this difference in nonunion rates was not statistically evident in patients who underwent single-level lumbar fusions; however, pseudarthrosis rates significantly increased in patients after two-level fusions (29.2% in smokers versus 10.9% in nonsmokers, p < 0.05). Persistent motion across the fusion segments is also thought to be associated with pseudarthrosis. Moreover, the incidence of pseudarthrosis increases as additional spinal levels are fused owing to the presence of a longer area that needs to be bridged by the fusion process as well as the presence of more motion across the fusion zone. Spinal instrumentation has been shown to increase the fusion rate by limiting the motion across fusion segments. A prospective randomized study by Fischgrund and colleagues showed significantly improved fusion rates at the end of 2 years when lumbar decompression and dorsolateral fusion were combined with instrumentation as compared to noninstrumented decompression and dorsolateral fusion.
The treatment of pseudarthrosis should begin with identification of biologic and mechanical factors that contribute to poor bone healing after spine fusion. Correction of endocrine and nutritional factors may assist in achieving solid fusions. Addition of nonrigid mechanical fixation has also been shown to lead to higher fusion rates. Surgical treatment includes repair of pseudarthrosis by exposure of the fusion area, removal of instrumentation, thorough decortication, and bone grafting with large quantity of autologous iliac crest bone graft. Local bone graft can also be harvested in clinically important quantities in order to aid fusion. Instrumentation is then reapplied with compressive forces across the fusion area. RhBMP-2 and osteogenic protein-1 have been useful in increasing the fusion rates in lumbar spine fusion surgeries and may be used effectively in cases of pseudarthrosis. At this time, the use of rhBMP-2 in a posterolateral fusion is considered off label use by the FDA. The most recent guideline update for the performance of fusion procedures for the lumbar spine recommends supplemental use of pedicle screw fixation as an adjunct to posterolateral fusion in patients who are at high risk for pseudarthrosis: specifically, patients who smoke, are on long-term steroids, require revision surgery, or may have medical conditions, such as osteopenia or osteoporosis, that preclude appropriate fusion.
Infection
Infection rates after spine surgery procedures have been shown to correlate with the duration of the procedure, associated patient comorbidities, and use of instrumentation. The rate of infection ranges from 2% to 4% in noninstrumented spine fusion and 6% to 11% in instrumented spine fusion. Staphylococcus aureus is the most common organism in postoperative spinal infections. Postoperative infections may result from direct inoculation or through hematogenous spread of infection from a remote source of infection. Statistically significant preoperative risk factors that are associated with increased risk of infection include age more than 60 years, smoking, diabetes, previous surgical infection, increased body mass index, and alcohol abuse. Intraoperative factors that are associated with increased risk of infection include staged procedure, operative time exceeding 5 hours, and fusions involving 7 to 13 levels.
Attempts to reduce preoperative and intraoperative risk factors may decrease the risk of infection and improve patient outcomes after spine fusion surgery. Surgeon familiarity with decortication, bone harvesting, and instrumentation techniques may reduce the operative time and decrease the risk of bacterial colonization. Preoperative antibiotics and repeat administration of antibiotics every 4 hours for long-duration surgeries, adequate intraoperative wound irrigation, intermittent repositioning of self-retaining retractors, and limiting the use of monopolar cautery may also decrease the incidence of postoperative infections. The safety and use of local application of vancomycin powder for the prevention of surgical site infections has produced conflicting results. Although the majority of the supporting literature is based on class III evidence, a systematic review and meta-analysis of the clinical evidence supports the safety and use of vancomycin powder for the prevention of surgical site infections in spine surgery. However, it is worthwhile to note that high local concentrations of vancomycin are purported to affect local wound healing and osteoblastic migration, and they may, therefore, contribute to the risk of pseudarthrosis.
The risk of a hematogenous spread of infection can be decreased by recognition and treatment of remote sources of infection. Early patient mobilization and the use of incentive spirometry may help to decrease the risk of pulmonary infections and deep venous thromboses. Careful sterile technique is essential during administration of the Foley catheter, and early removal of indwelling catheters, if appropriate, is encouraged to prevent catheter-related urinary tract infections.
Surgical treatment of postoperative wound infections should include debridement of infected and necrotic tissues and obtaining sufficient cultures. Exposure of the fusion mass and the hardware with removal of infected unincorporated bone graft material followed by thorough antibiotic irrigation with a pulsatile lavage system should be carried out. Bone grafting material can then be replaced. Postoperative wound infection can usually be treated without removal of hardware.
Adjacent Segment Disease
The use of posterior lumbar fusion (PLF) has increased in the treatment of various spinal degenerative pathologies including spondylolisthesis, scoliosis, and degenerative disc disease. In light of this development, special attention should be paid to more long-term postoperative sequelae of lumbar fusion, namely adjacent segment disease (ASD). Adjacent segment disease can be defined as any lumbar segment pathology occurring adjacent to the solid fusion construct that is manifested by clinically significant symptoms. This may range from worsening or new development of spinal stenosis, segmental instability, radiculopathy, or deformity. Although ASD has been described in the past to be one of the more rare entities following posterior thoracic or lumbar fusion, longer-term follow-up studies have revealed that ASD may occur in up to 20% of the fusion population over a 10-year span. Several studies have reported the prevalence and potential risk factors that may lead to ASD after fusion surgery; however, many of the described outcomes are conflicting and unclear. The incidence of radiographic ASD is described in the literature to be anywhere between 8% and 100%, whereas the incidence of symptomatic ASD is estimated between 5.2% and 18.5%. Furthermore, the incidence of surgical revision for symptomatic ASD is even lower, between 2% and 15%.
As a result of the contradictory data regarding ASD, few long-term studies with large cohorts of patients undergoing posterior lumbar interbody fusion (PLIF) or PLF have been published in the more recent literature. In a retrospective study for a cohort of 490 patients who underwent lumbar fusion of three or fewer segments by PLIF and PLF, Lee and associates further elucidated the prevalence and incidence of ASD as well as described the risk factors for its development. In a chart review of patients treated between August 1988 and December 2007 (mean follow up 51 months), the authors demonstrated that ASD requiring surgery was predicted for 5.8% of patients at 5 years and 10.4% for patients at 10 years after lumbar spinal fusion. Additionally, they found PLIF was associated with 3- to 4-times higher incidence of ASD requiring surgical intervention than PLF. Lee and associates also reported that risk for symptomatic ASD requiring surgery was 2.5 times higher in patients over the age of 60 than those younger than 60 years.
In a retrospective study of 511 patients who underwent posterolateral fusion for degenerative spine disease, Bydon and colleagues demonstrated that more than 15% of patients developed ASD during a period of 40 months. Of these patients, 256 underwent floating fusion (L1 through L5) and 255 underwent lumbosacral fusion; ASD developed in 19.14% of patients who underwent floating fusion versus 12.16% in the lumbosacral fusion group. These findings were consistent with a 2008 study by Disch and coworkers who reported that patients who undergo posterior lumbar fusions, not including the lumbosacral junction, may be at heightened risk for ASD. Nevertheless, the majority of these conclusions are based primarily on retrospective reviews. Therefore, additional prospective studies are ultimately necessary to further delineate the risk and incidence of adjacent segment disease in patients undergoing posterior thoracic and lumbar fusion.
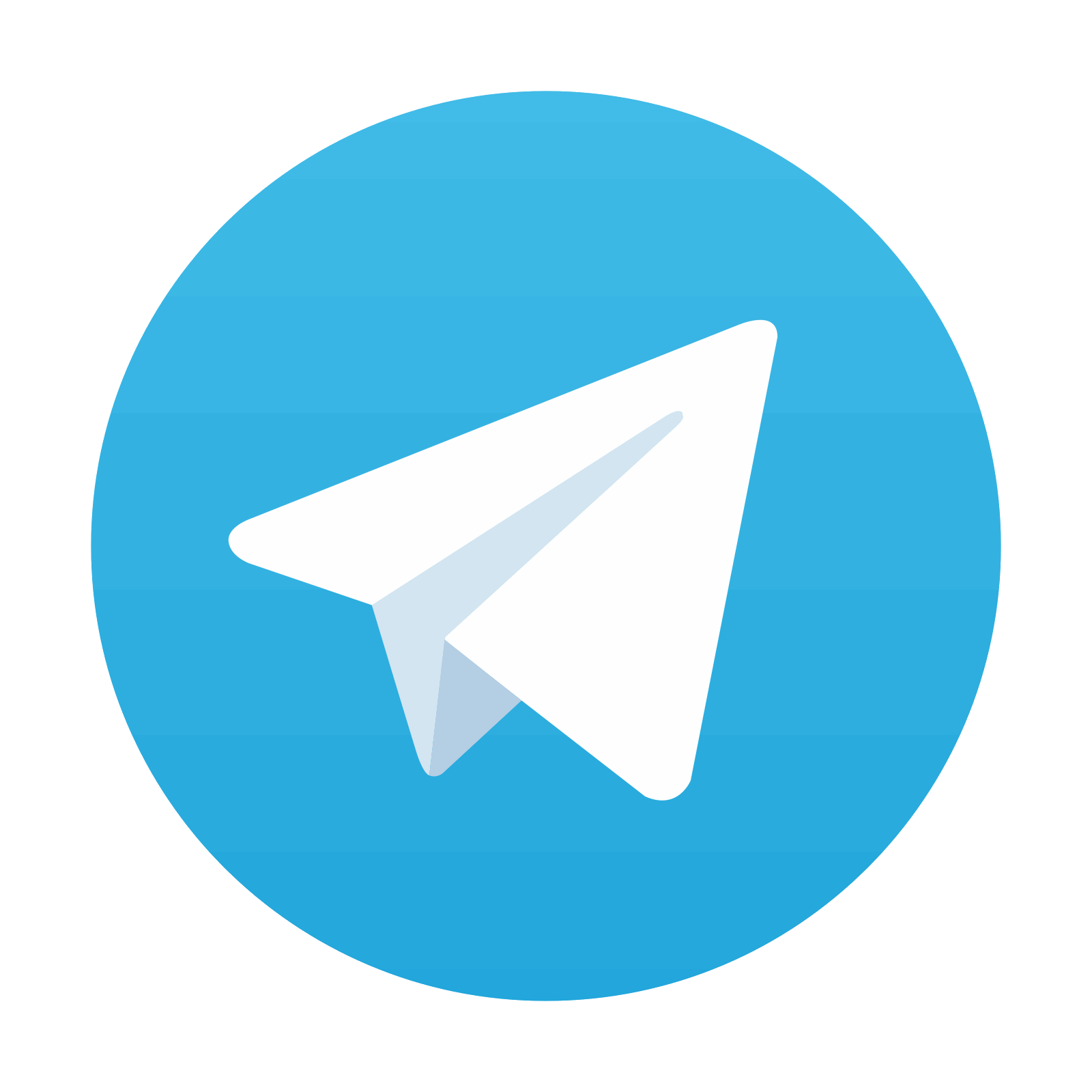
Stay updated, free articles. Join our Telegram channel

Full access? Get Clinical Tree
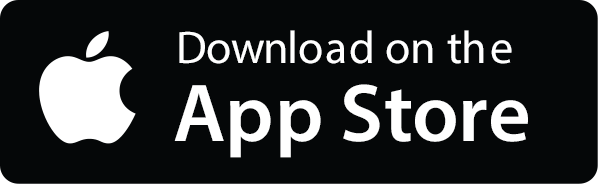
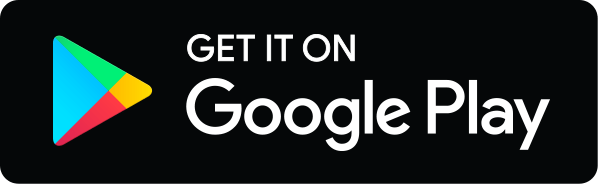