Abstract
The development of hydrocephalus substantially worsens the neurodevelopmental prognosis after intraventricular hemorrhage (IVH). Frequent standardized measurements of ventricular dimensions and daily head circumference are essential after grade III and IV IVH to diagnose hydrocephalus and to assess progression. Free radical injury, inflammation, raised intracranial pressure, and physical distortion injure white matter and contribute to the high frequency of cerebral palsy and cognitive impairment in posthemorrhagic hydrocephalus (PHH). There is evidence that transforming growth factor β (TGF-β) is released after IVH and upregulates extracellular matrix proteins which, like cement, interfere with reabsorption of CSF, resulting in hydrocephalus. Ventriculoperitoneal shunt (VPS) surgery is contraindicated early because of the fragility of the infant, the high risk of infection as well as the blood, and protein in the cerebral spinal fluid, which cause blockage. Many different interventions have been used to treat PHH, but none has been shown objectively to avoid VPS that results in the infant acquiring a lifelong set of new potential problems. Standard treatment of excessive head enlargement or pressure symptoms is to begin with lumbar punctures if ventricular width exceeds the 97th centile plus 4 mm and to insert a ventricular reservoir or subgaleal shunt if repeated taps are needed. The only intervention shown by randomized trial to improve outcome is a demanding procedure—drainage, irrigation, and fibrinolytic therapy (ventricular lavage)—that is designed to remove free iron and proinflammatory cytokines. In an animal model, intraventricular mesenchymal stem cell injection 2 days after IVH prevented inflammation, myelin loss, neuromotor dysfunction, and PHH. This is now being tested in a feasibility and safety clinical trial.
Keywords
cerebral palsy, hydrocephalus, intraventricular hemorrhage, preterm infant, transforming growth factor β, ventricular reservoir, ventriculoperitoneal shunt
- •
Posthemorrhagic hydrocephalus is characterized by deposition of extracellular matrix proteins weeks following intraventricular hemorrhage.
- •
Periventricular white matter may be progressively injured by raised intracranial pressure, inflammation and free radical injury.
- •
There is not one standard approach to treating post hemorrhagic hydrocephalus.
- •
Ventricular lavage remains an experimental therapy.
Hemorrhage into the ventricles of the brain is one of the most serious complications of premature birth despite improvements in the survival of premature infants. Large intraventricular hemorrhage (IVH) has a high risk of neurologic disability, and more than 50% of children with IVH go on to have progressive ventricular dilation. Increasing survival of extremely premature infants is associated with posthemorrhagic ventricular dilation (PHVD) with high morbidity and considerable mortality. Overall, approximately two-thirds of these children have cerebral palsy and about one-third have multiple impairments. The term posthemorrhagic hydrocephalus is generally reserved for cases in which PHVD is persistent and associated with excessive head enlargement. This condition still does not have a safe and effective “cure,” but advances in our understanding of the pathophysiology and experience from clinical trials allow us to suggest some guidelines on assessment and management and to identify gaps in knowledge where further advances are needed.
A mother in her third pregnancy suffered a placental abruption at 28 weeks and delivered a male infant weighing 877 g at delivery. He was intubated at birth and received surfactant prophylactically. He was ventilated at low pressures and had a low oxygen requirement until, on day 2, he suffered a pulmonary hemorrhage with a period of hypotension (mean arterial pressure below 25 mm Hg for 2 hours), which was corrected with the use of dopamine and blood transfusion. His respiratory status stabilized within hours. On day 3 a cranial ultrasound scan showed bilateral IVH ( Fig. 3.1A ). He progressed from minimal ventilation settings to nasal continuous positive airway pressure. He then had scans twice a week. Ventricular dimensions progressively enlarged until day 18 (see Fig. 3.1B ). Head circumference had increased by 1.5 cm over 7 days. A lumbar puncture (LP) produced 12 mL (10 mL/kg) of port wine–colored cerebrospinal fluid (CSF). This procedure reduced head circumference by 0.3 cm. Two days later, head circumference had increased by 0.5 cm from the postpuncture measurement. A second LP was carried out, which again produced 12 mL (10 mL/kg) of CSF. Head circumference decreased by 0.3 cm but then increased by 0.5 cm from the postpuncture measurement 2 days later. A third LP produced only 6 mL of CSF before flow stopped. Head circumference did not decrease, the fontanelle remained full, and ultrasonography confirmed that the ventricles were still “ballooned.”


As there was a need for repeated tapping of CSF and repeated LP was becoming impractical, an Ommaya reservoir (ventricular access device) was inserted frontally in the right ventricle with the patient under general anesthesia. At the time of its insertion, 13 mL (10 mL/kg) of CSF was removed. To avoid raised pressure and resultant CSF leak with a risk of infection, the reservoir was tapped daily, at 10 mL/kg per day for 5 days. Thereafter the reservoir was tapped as required to control excessive expansion or suspected pressure symptoms. Head circumference enlargement necessitated tapping 10 mL/kg every 1 to 2 days. Pressure measurement at the start of tapping typically showed a pressure of 6 to 7 mm Hg. After 10 mL/kg had been removed, pressure had fallen to 3 mm Hg. Clinically, apnea increased at the time of head expansion and decreased after tapping.
This regimen of tapping as required was reviewed every 7 days to confirm that head enlargement in 1 week had not been excessive. CSF protein was initially 4.1 g/L. Tapping the reservoir continued to be necessary for 6 weeks, and on a few days, it was obvious that 10 mL/kg had been insufficient to control head enlargement and the subsequent tap had been increased to 15 mL/kg. CSF protein continued to remain high (1.8–2.0 g/L), and tapping was continued for a further 2 weeks, by which time CSF protein had decreased to 1.45 g/L and the infant’s weight had risen to nearly 2500 g. Nasal catheter oxygen was no longer required. A ventriculoperitoneal low-pressure shunt was inserted when Infant A reached full-term gestational age. Postoperatively there was no pulmonary problem, CSF leak, or infection but Infant A initially needed to be placed on a considerable head-up tilt to facilitate adequate shunt function and control head circumference. Although control of head circumference and suspected pressure had been maintained, magnetic resonance imaging (MRI) at term showed considerable ventricular dilation with some loss of periventricular white matter.
Question 1: What Measurements of Ventricular Size Are Used in Diagnosis of PHVD?
The chances of progressive ventricular dilation increase with the amount of blood visible in the ventricles. With a small IVH (grade II on Papile scale or IIa on De Vries grading ), measurement of ventricular size once a week for 4 weeks and then at discharge is appropriate; with a large IVH (grade III on Papile scale or IIb on De Vries grading ), twice-weekly ultrasonography is needed because dilation is likely and may be rapid. Although large, balloon-shaped ventricles are obvious without formal measurements, quantitative documentation is essential if serial scans are being done by different ultrasonographers as well as in epidemiologic studies and clinical trials. Reference ranges for measurement of the width (midline to lateral border) of the lateral ventricles at the midcoronal level were first published in 1981. Since 1984, an “action line,” defined as width 4 mm higher than the 97th centile width for age, has been used as a definition of serious PHVD in therapeutic trials and as a secondary outcome in randomized trials of neonatal intensive care interventions ( Fig. 3.2A ). This measurement has the advantage of being highly reproducible among observers because it is relatively unaffected by anterior or posterior angulation of the scan head as the lateral wall of the ventricle in this orientation runs fairly parallel to the midline. The frequency of PHVD using this definition is 1 in 3000 births among residents of Bristol, United Kingdom. However, ventricular enlargement is not always sideways, and sometimes the most marked change is posterior enlargement or a change from thin slit to round balloon. With this in mind, Davies and colleagues published reference ranges for anterior horn width (to capture the change in shape to balloon) (95th centile approximately 3 mm), thalamo-occipital width (to capture posterior enlargement) (95th centile approximately 25 mm) ( Fig. 3.2B ), and third ventricle width (95th centile approximately 2 mm). My colleagues and I have found the anterior horn, thalamo-occipital, and third ventricle widths to be practical and useful but with greater interobserver variation. We have used all three measurements since 2003, requiring all three measurements (bilaterally) to be 1 mm over the 95th centile as a criterion for PHVD.


Question 2: How Can Ventricular Dilation Driven By Cerebrospinal Fluid Under Pressure Be Distinguished From Ventricular Dilation Caused By Loss of Periventricular White Matter?
The distinction of CSF under pressure and the loss of periventricular white matter as the cause of ventricular dilation are important because removing fluid that has accumulated as a replacement for dead brain is unlikely to improve outcome.
CSF-driven ventricular enlargement can be slow or rapid, it is characterized by balloon-shaped lateral ventricles, and if CSF pressure is measured, it is found to be raised or near the upper limit of normal (mean 3 mm Hg, upper limit 6 mm Hg ). Furthermore, head circumference growth over time is accelerated, although it may lag ventricular enlargement by 1 to 2 weeks. In contrast, ventricular enlargement from atrophy is always slow, it is more irregular in outline rather than balloon shaped, and if CSF pressure is measured, it is found not to be raised. Head circumference velocity is either normal or slow but is not accelerated. Nonprogressive mild ventricular dilation at term is a marker of periventricular leukomalacia.
Question 3: How Is Excessive Head Enlargement Defined?
Head circumference normally enlarges by approximately 1 mm/day between 26 weeks of gestation and 32 weeks, and about 0.7 mm/day between 32 and 40 weeks. We regard a persistent increase of 2 mm/day as excessive. Measuring head circumference accurately, although “low-tech,” is not as easy as it sounds. The relevant measurement is the maximum fronto-occipital circumference. Detecting a difference of 1 mm from day to day is difficult, and we do not react to a difference of 2 mm from 1 day to the next unless there is other evidence of raised intracranial pressure. However, an increase of 4 mm over 2 days is more likely to be real, and an increase of 14 mm over 7 days is definitely excessive.
Question 4: How Is Raised Intracranial Pressure Recognized?
It is possible to detect a change in palpation of the fontanelle from concave to bulging and to document excessive head enlargement. The preterm skull is very compliant and can easily accommodate an increase in CSF by expanding with separation of the sutures. When CSF pressure was measured with an electronic transducer in infants in whom ventricles were expanding after IVH, the mean CSF pressure was approximately 9 mm Hg, 3 times the mean in normal infants. There was a considerable range, with ventricle and head expansion in some infants at a pressure of 5 to 6 mm Hg, and in a small number with CSF pressure around 15 mm Hg. A CSF pressure of 9 mm Hg does not necessarily produce clinical signs but may be associated with an increase in apnea or vomiting, hypotonia, hypertonia, or decreased alertness.
Obtaining serial calculations of the Doppler flow-velocity resistance index (RI) on the anterior cerebral artery is a useful and practical way of detecting impairment of cerebral perfusion by raised intracranial pressure and can easily be done during ultrasound imaging. The resistance index is calculated as follows: (systolic velocity ‒ diastolic velocity)/systolic velocity. This measurement is independent of the angle of insonation. If intracranial pressure rises to a level exceeding the infant’s compensation, end-diastolic velocity tends to decrease, eventually becoming zero (the RI is then 1.0) ( Fig. 3.3 ). Serial increases in RI above 0.85 while the ventricles are rapidly expanding would be evidence that pressure is rising. This statement assumes that the infant does not have a significant left-to-right shunt at the ductal level and that the partial pressure of carbon dioxide (P co 2 ) has not decreased recently, because both of these physiologic changes could increase RI. Severe intracranial hypertension may cause reversed end-diastolic velocities. The sensitivity of the RI can be increased by applying pressure to the fontanelle during the examination. An infant who is close to the limit of cranial compliance responds with a large decrease in end-diastolic velocities—that is, an increase in RI. Amplitude-integrated electroencephalography (EEG) may show a deterioration, with electroencephalographic activity becoming less frequent as dilation increases and improving with effective CSF drainage.

Question 5: What Is Infant A’s Prognosis?
The prognosis at diagnosis of PHVD using the preceding criteria is influenced by the presence of identifiable parenchymal lesions. The Ventriculomegaly Trial and the PHVD Drug Trial used the 4 mm plus 97th centile definition of PHVD and had standardized follow-up. Of children in whom ultrasonographic examination shows no persistent echodensities or echolucencies (cysts), approximately 40% will have cerebral palsy and about 25% will have multiple impairments. Cerebral MRI at term is increasingly used to assess infants with PHVD because this technique can reveal parenchymal injury that cannot be easily demonstrated with ultrasonography. Lesions detected by MRI include abnormal signal in the white matter without cyst formation, gray matter abnormality, and cerebellar secondary atrophy. In Infant A’s case, there were no ultrasonographic abnormalities in the parenchyma, and thus the risk of some level of cerebral palsy would be no higher than 40%.
Question 6: What Is the Mechanism of PHVD?
After a large IVH, multiple blood clots can obstruct the ventricular system or channels of reabsorption, initially leading to a phase of CSF accumulation. Although tissue plasminogen activator (TPA) can be demonstrated in posthemorrhagic CSF, fibrinolysis is very inefficient in the CSF, which has low levels of plasminogen and high levels of plasminogen activator inhibitor. This potentially reversible obstruction by thrombi may lead to a chronic obliterative, fibrosing arachnoiditis, and subependymal gliosis involving deposition of extracellular matrix proteins in the foramina of the fourth ventricle and the subarachnoid space. Fig. 3.4A shows the brainstem and cerebellum of an infant with PHVD who died at age 2 months. A layer of collagenous connective tissue surrounds the brainstem. Fig. 3.4B shows perivascular deposition of the extracellular matrix protein, laminin, in the subependymal region in another infant with PHVD who also died at age 2 months.

Transforming growth factor β (TGF-β) is likely to be a key mediator of this process because TGF-β is involved in the initiation of wound healing and fibrosis. TGF-β elevates the expression of genes encoding fibronectin, various types of collagen, and other extracellular matrix components, and is involved in a number of serious diseases in which there is excessive deposition of collagen, including diabetic nephropathy and cirrhosis. TGF-β has three isoforms, β1, β2, and β3. TGF-β1 is stored in platelets. Thus IVH, by definition, provides a store of TGF-β1 for many weeks in the CSF. TGF-β is elevated in the CSF of adults with hydrocephalus after subarachnoid hemorrhage, and intrathecal administration of TGF-β to mice resulted in hydrocephalus. My colleagues and I have demonstrated that TGF-β1 and TGF-β2 concentrations in CSF from infants with posthemorrhagic ventricular dilation are 10 to 20 times those in nonhemorrhagic CSF and that the concentration of TGF-β in CSF is higher in those shunted later. Heep and colleagues have confirmed elevations of TGF-β1 in posthemorrhagic hydrocephalus CSF as well as a product of TGF-β, amino-terminal propeptide of type I collagen. Chow and associates have demonstrated elevation of TGF-β2 and nitrated chondroitin sulfate proteoglycans (an extracellular matrix protein) in CSF from preterm infants with posthemorrhagic hydrocephalus.
A rat pup model of PHVD has also provided evidence of the involvement of TGF-β and its downstream products, fibronectin, laminin, and vitronectin. Transgenic mice that overexpress TGF-β1 in the central nervous system are born with hydrocephalus. Thus there is a strong possibility of a role for the TGF-βs in the development and/or maintenance of hydrocephalus after ventricular hemorrhage. However, two drugs that inhibit TGF-β, pirfenidone and losartan, did not reduce ventricular size or improve neuromotor performance in a rat pup model of PHVD.
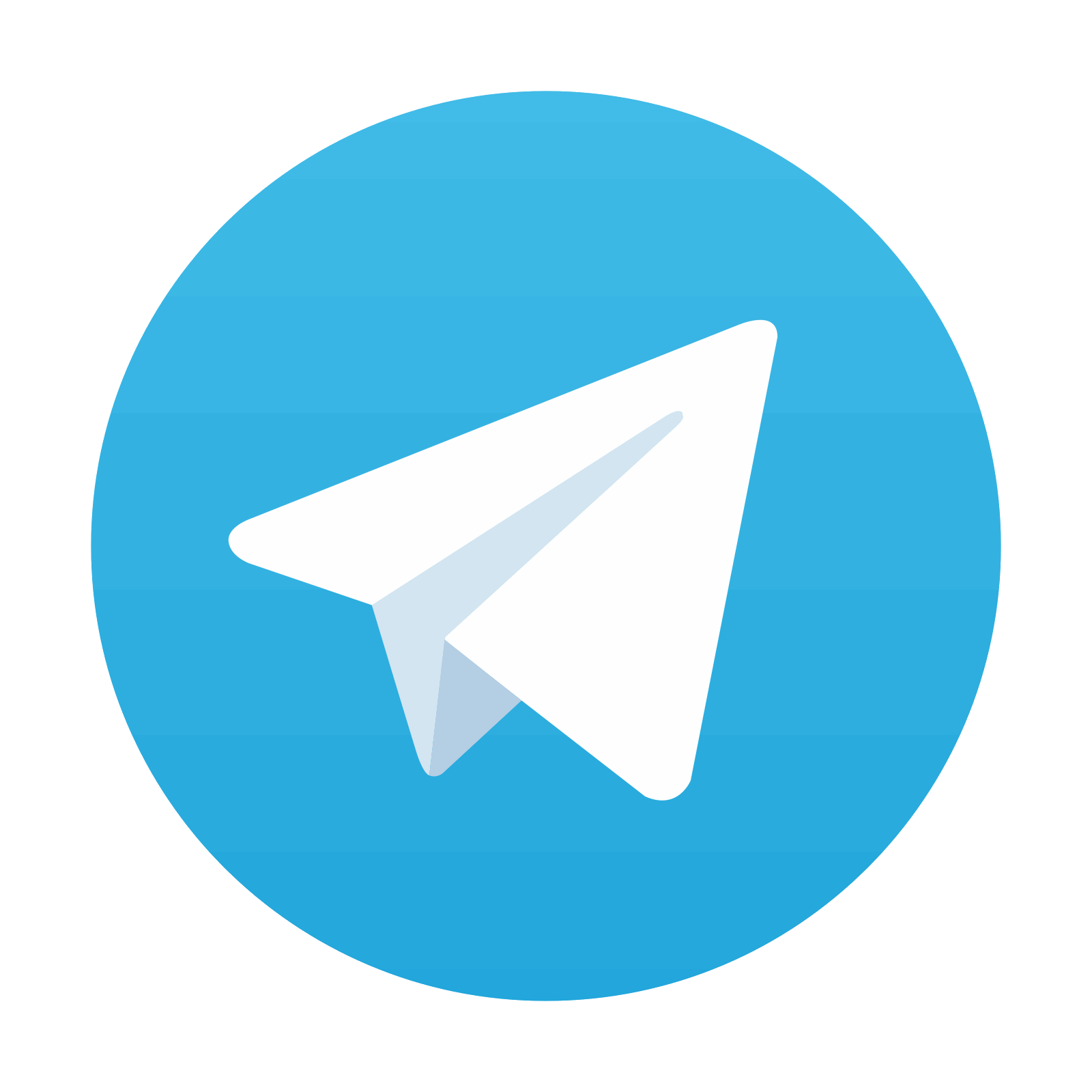
Stay updated, free articles. Join our Telegram channel

Full access? Get Clinical Tree
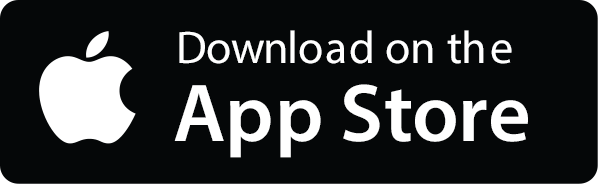
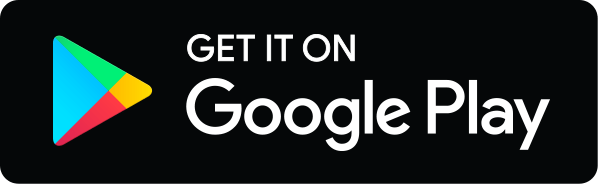