Postoperative Considerations
Postoperative care begins with the patient′s emergence from anesthesia. The results of the initial neurologic examination obtained after awakening, the first in the succession of frequent neurologic assessments, are compared with those of both the preoperative and subsequent examinations to document steady improvement as the anesthetics are metabolized, or to alert the clinician to an unexpected deficit. Prompt arousal reduces the apprehension typical of surgeons in the immediate postoperative period. The patient′s failure to awaken to the clinician′s satisfaction or the patient′s demonstrating a focal deficit, such as motor asymmetry or pupillary abnormality, may prompt an imaging study straight from the operating room.
Expectations of timely emergence must be tailored to several factors, such as the experience of the anesthetist, when the agents were discontinued, and the extent of surgery. Tumor size can also affect emergence.1 Transient neurologic changes known to occur during awakening from anesthesia, such as hyperreflexia, clonus, upgoing plantar responses, and pupillary abnormalities,2 are seen less often now that the inhalational agents halothane and enflurane are largely supplanted by isoflurane. Recently, sevoflurane has been shown to facilitate earlier post-anesthesia recovery and neurologic assessment when compared with isoflurane,3 and is now the inhalational anesthetic of choice for pediatric patients undergoing neurosurgical procedures.
Children and infants are always transported from the operating room with supplemental oxygen, as they are likely to be at greater risk than adults for postanesthetic desaturation.4 Once the patient is in the recovery room or the pediatric intensive care unit (PICU), standardized neurologic examinations at regular intervals, initially every 15 minutes, remain the most important determinant of the patient′s neurologic status. This requires skilled nursing, compulsive documentation, and efficient communication within a team approach; the physicians, although ultimately responsible, are only a part of the team. Knowledge of the patient′s preoperative status is essential for all postoperative caregivers in terms of a detailed history, adequacy of seizure prophylaxis, or existence of a preoperative deficit. Likewise, intraoperative data, such as fluid balance and estimated blood loss, whether brain retraction may increase the risk of brain edema, or if problematic hemostasis may herald a potential bleeding problem, are also key. Thus, all important preoperative and intraoperative information should be documented by the surgeons and disseminated to all physicians and nurses who will have a role in the postoperative care of the child.
As a general rule, children who undergo brain tumor surgery are monitored postoperatively in the PICU. Certain preoperative and perioperative variables have been found to be predictive of an extended need for PICU monitoring after brain tumor surgery. These include preoperative radiographic findings suggesting increased tumor severity (location, mass effect, and presence of midline shift), intraoperative blood loss, and the need to keep the patient intubated postoperatively.4 PICU interventions are much more common in these patients.5
Perioperative morbidity and mortality continue to be a necessary evil with intracranial surgery. A recent prospective study of complications occurring after pediatric neurosurgical procedures found an overall rate of 16.4%.6 Complications following brain tumor surgery were the second most common after cerebrovascular surgery.7 In general, the complication rate is lower after surgery for brain tumors than following less elective (i.e., trauma) intracranial procedures. Decades of advances continue to improve outcome in this population. As a result of refinements in surgical technique, Cushing8 in 1932 reported a decrease in mortality from 30.9% to 11.6% in a career-long retrospective of 2000 cases of intracranial tumor. The mortality rate of brain tumor surgery was further reduced by the advent of perioperative steroid use, dropping to less than 3% by the 1960s.9 A review published in the early 1990s of nearly 1800 craniotomies for supratentorial tumor resections found a perioperative mortality rate of 2.1%,10 and a very recent single-center experience found an even lower rate of 0.8%.11 Clearly, then, the great majority of children who undergo brain tumor surgery should have as their main nemesis the natural history of the disease (and the rigors of adjuvant therapy, if indicated) rather than negative sequelae of the operation itself. Surgery, in a word, should be the least of their problems.
Therefore, the goals in postoperative management are recognition of potential systemic, metabolic, or neurologic complications; prevention or minimization of the sequelae of these complications by intervention as necessary; and maintenance or restoration of homeostasis until the patient is stable enough to convalesce in a less vigilant setting. As all complications are considered potentially life threatening if unrecognized or untreated, meticulous attention to detail must be the rule in treatment of the postoperative patient ( Table 44.1 ).
Neurologic Assessment
As stated, repeated neurologic evaluation is the first line of defense in early detection of potential problems. These are performed every 15 minutes for the first hour upon arrival at the recovery room or PICU, and regular hourly assessment is the typical routine once the patient has been stabilized. Although age-related variations exist, the basic approach of clinical observation and response to stimulation (noxious, auditory, visual) remains the mainstay of the neurologic evaluation. Regardless of age, the level of alertness, brainstem reflexes, and motor function can be evaluated in all age groups.
Recognize potential complications: systematic, metabolic, neurologic |
Prevent of minimize secondary injury resulting from complications; intervene as necessary |
Maintain or restore homeostasis |
Follow patient; give meticulous care |
Neurologic Examination
The Younger Patient
There are several modified versions of the Glasgow Coma Scale for application to infants and children ( Tables 44.2, 44.3, 44.4, 44.5, 44.6 ).12–16 There is no consensus opinion recommending one system over another, and the pediatric scales are likely subject to more intraobserver variability than is the adult scale. The neonate and infant can be observed for spontaneous facial and limb movements, eye opening and extraocular muscle function, respiratory pattern, and vocal output. With respect to motor function, the detection of asymmetry is the most important abnormal finding, alerting the clinician to a possible hemiparesis.17 Responses can be elicited with tactile or noxious stimuli, and the character of cry can also be assessed. Pupillary response and other brainstem reflexes can be tested. The ability to palpate an open fontanel cannot be overemphasized as a means of directly and rapidly providing a gross clinical assessment of intracranial pressure (ICP), with descriptions such as “flat,” “soft,” “full,” “bulging,” or “tense” being often utilized. Table 44.7 lists several findings that may be considered abnormal in older children and adults but normal in patients younger than 2 years of age.
Younger child | Older child |
Observation | Observation |
Level of alertness | Level of alertness |
Eye opening | Eye opening |
Facial and limb movement | Motor function |
Extraocular muscle function | Spontaneous |
Respiratory pattern | Purposeful |
Vocal output | Verbal output |
Fontanelle palpation (soft, flat, full, bulging, tense) | Orientation |
Elicited responses | Follow commands |
Brainstem reflexes | Midline |
Pupillary response to light | Lateralizing |
Corneal reflex | Three-step midline crossing |
Gag reflex | Elicited responses (unconscious) |
Motor response to stimulation | Brainstem reflexes |
Vocal response to stimulation | Pupillary response to light |
Corneal reflex | |
Gag reflex | |
Oculovestibular reflex | |
Motor | |
Response to noxious stimuli | |
Reflexes | |
Extremity tone | |
Deep tendon reflex | |
Plantar reflex (Babinski) |
The Older Patient
The neurologic assessment of older children and adolescents follows that used with adults. Typically, the level of alertness is assessed first, concurrent with notation of the presence or absence of spontaneous or purposeful movements. If they eyes are not spontaneously open, the ability of the patient to open his or her eyes to vocal or noxious stimuli is observed. Orientation and verbal responses are then gauged. The ability to follow commands can be graded, with a midline command (e.g., tongue protrusion) considered simpler or more primitive than a lateralizing command (e.g., the showing of two fingers). Having the patient touch the opposite ear with a specified finger, such as the thumb, is useful as a more complex three-step midline-crossing command. If the patient is not conscious, testing of the brainstem reflexes, including pupillary response and corneal and gag reflexes, is mandatory. Likewise the motor response to noxious stimuli and assessment of long-tract signs, including tone and deep tendon and plantar reflexes, is basic to the evaluation of an unconscious patient. The Glasgow Coma Scale can be useful under these conditions ( Table 44.3 ). Though initially validated as a prognosticator for head-injured patients,12,13 the scale has found widespread utility in the evaluation of neurologically compromised patients regardless of etiology.
Often the postoperative neurologic examination is tailored to the surgery performed. The examiner may pay closer attention to tests for ataxia, dysarthria, voice quality, and tongue deviation in patients who have undergone posterior fossa surgery. The visual fields may warrant closer inspection after cases of suprasellar or hypothalamic tumor surgery, whereas after supratentorial tumor resection the examiner may focus more on tests of motor function. In all cases, it is the early detection of neurologic deterioration through regular examination that offers the patient the best chance of optimizing outcome ( Table 44.7 ).
Vital Signs
In the postoperative patient, vital signs should be considered an essential component of the complete neurologic evaluation rather than a separate set of parameters to follow. These include body temperature, heart rate, and rhythm via surface electro-cardiography, respiratory rate and pattern, and arterial blood pressure. Pulse oximetry is always followed as well. Knowledge of the age-related and diurnal variations of the normal range for heart rate, respiratory rate, and blood pressure is a prerequisite.
Temperature
Younger patients can experience wide fluctuations in core temperature; esophageal or rectal probes are commonly used. Both elevated and depressed temperatures can affect the level of consciousness and the neurologic exam, and patients with compromised cerebral function are likely more sensitive to these changes ( Table 44.8 ).18 The definition of hypothermia varies from source to source, ranging from below 32.2°C19 to 35°C20 to any temperature significantly below 37°C.21 It may occur following general anesthesia, especially after prolonged surgery, and is seen commonly, with untoward effects resulting if the problem is not addressed. Prolongation of emergence secondary to hypothermia can delay the subsequent detection of a deficit; shivering and rewarming can increase oxygen consumption and potentially exacerbate an hypoxemia; and the pressure of air trapped intracranially can increase as the air warms.22 Arrhythmias, dehydration, and lactic acidosis can also result if hypothermia goes untreated.23 Hypothermia can also be seen with posterior hypothalamic dysfunction following resection of tumors near this region, such as gliomas or craniopharyngioma,19,22,24 or as a syndrome associated with agenesis of the corpus callosum (Shapiro syndrome).25 Therapy generally consists of use of heat lamps, blankets, humidified oxygen, and, if necessary, warmed intravenous fluids. Hypothermia is one of the major causes of postoperative morbidity in infants and neonates and as such is aggressively treated as outlined.
Postoperative fevers in children are common. Temperatures above 38.5°C often occur within the first 24 to 48 hours after surgery, typically a self-limiting stress-induced pyretic response. Though commonly attributed to atelectasis, and there is some controversy regarding this contention,26,27 a diagnostic workup during this period is generally of low yield. There is little agreement and wide variation in the literature as to the incidence of postoperative fever, ranging from 14 to 91%.28 An incidence of 73% and 76% has been reported after pediatric orthopedic and craniosynostosis surgeries,29,30 respectively, and as high as 82% after hemispherectomy. A true infectious source was found in only 1 to 6%,29–31 though up to 14% in the immunocompromised postsurgical child.32 A survey of nosocomial infection in the PICU found bloodstream, pulmonary, urinary tract, and surgical sites to be the predominant sources.33 Meningitis is always a concern in the postoperative patient for whom another source cannot be found, and the workup may eventually lead to a lumbar puncture for cerebrospinal fluid (CSF) evaluation. Drug-induced fever by phenytoin is a well-known phenomenon,19,34 usually diagnosed by exclusion, as is tumor fever.26 Fevers secondary to anterior hypothalamic dysfunction (neurogenic or central) are usually high, approaching 40°C.19 The postictal state is also commonly associated with elevated temperature.
Recent studies in pediatric traumatic brain injury have found that fever increased both length of stay and risk for lower hospital discharge Glasgow Coma Scale score.35,36 Fever can negatively affect the neurologic status of a patient, even leading to coma if greater than 42°C,37 and is known to increase CO2 concentration and cerebral blood flow (CBF), which can exacerbate increased ICP.38,39 Hence, a search for a treatable source is typically initiated, guided by clinical status and the timing of fever onset relative to surgery. Every attempt should be made to aggressively lower the temperature below 38.5°C23 with cooling blankets or more aggressive measures, if antipyretics and source-specific treatment plans are not effective.
Hypothermia |
Anesthesia |
Posterior hypothalamic dysfunction |
Congenital |
Drugs |
Hyperthermia |
Wound infection |
Meningitis |
Atelectasis |
Pneumonia |
Urinary tract infection |
Anterior hypothalamic dysfunction |
Seizure |
Tumor fever |
Drugs |
Heart Rate and Blood Pressure
Close continuous monitoring of the heart rate and arterial blood pressure is absolutely essential in the postoperative patient. Changes can indicate a physiological response to several potentially dangerous situations, prompting imaging studies, more invasive monitoring, or therapeutic interventions. Therefore, fluctuations must be correlated with the clinical status to help determine the best course of action. The development of sinus tachycardia may result from inadequate analgesia, agitation, fever, hypovolemia, or increased ICP.40,41 Primitive cardiovascular autonomic control in infants and young children translates to a predominance of parasympathetic tone and decreased sympathetic tone.40 This can result in an exaggerated vagotonic response and reflex bradycardia in several situations, including hypoxia, laryngotracheal stimulation, gastroesophageal reflux, as well as increased ICP as part of the Cushing reflex ( Table 44.9 ).
The combination of hypertension and bradycardia, along with irregular respiration, in association with increased ICP, was described by Cushing at the turn of 20th century.42,43 In the postoperative setting, these signs should raise concerns about the possibility of an expanding intracranial mass lesion, such as hemorrhage or edema. Local pressure on the brainstem may also manifest in a Cushing reflex, as later work demonstrated the reflex to be secondary to pressure-sensitive areas in the dorsal brainstem at the pontomedullary junction, along the floor of the fourth ventricle.44 In children, the hypertension component of the reflex may be absent or not clinically obvious, and brady-cardia alone should likewise raise suspicions of increased ICP.19 Similarly, isolated hypertension in the absence of bradycardia has also been noted in children with high ICP.45
Decreased heart rate |
Hypoxia |
Intubation |
Gastroesophageal reflux |
Increased ICP |
Elevated heart rate |
Pain-related agitation |
Fever |
Hypovolemia |
Although hypertension most commonly occurs secondary to pain, it should be controlled because the risk of hemorrhage into the tumor resection bed is increased with higher pressures. Simple maneuvers, such as analgesia or sedation, may be successful, and acetaminophen with or without codeine is usually sufficient. If sedating agents are used, they should be reversible (e.g., morphine). However, the use of antihypertensive agents may be necessary. This may not be straightforward in a patient with increased ICP because overly aggressive lowering of the pressure may compromise cerebral perfusion. Adrenergic receptor antagonists, such as labetalol, are often preferred over direct vasodilators, such as nitroprusside, as the latter may negatively affect ICP.46 Continuous labetalol infusion has been found to be safe and efficacious in the management of hypertensive crisis in children under 24 months of age.47 Infusions of calcium channel antagonists, such as nicardipine and clevidipine, are also particularly effective in the pediatric population at providing controlled hypotension during surgery as well as management of hypertension after surgery.48,49
Hypotension must quickly be corrected to avoid compromise of systemic and cerebral perfusion. Loss of blood must be ruled out as an etiologic factor ( Table 44.10 ). Although ongoing, hemodynamically significant blood loss after brain tumor surgery is rare, inadequate resuscitation from intraoperative blood loss is one of the most common causes of serious morbidity and even mortality in young postoperative patients. In procedures with significant blood loss, the hematocrit is measured immediately following surgery, and usually every 6 hours for the next 24 hours. It is difficult to resuscitate a patient once the hematocrit falls below 17%. Additional causes of hypotension in the postoperative patient include fluid depletion and hypovolemia, which can result from diuretic or mannitol use during treatment for increased ICP; diabetes insipidus (DI) following resection of tumors near the pituitary gland; vasodilatation secondary to fever or sepsis; and adrenal insufficiency secondary to inadequate exogenous glucocorticoid coverage. The latter occurs in steroid-dependent patients who are unable to mount an appropriate perioperative stress response.50
Knowledge of the blood pressure is crucial in the management of increased ICP and cerebral perfusion. Cerebral perfusion pressure (CPP) is the difference between the systemic mean arterial blood pressure (MAP) and ICP:
CPP = MAP – ICP
Thus, it can be appreciated how fluctuations in blood pressure can affect the CPP and, through complex interactions soon to be discussed, the ICP as well. Therapeutic modalities aimed at manipulating these interactions are increasing, and blood pressure will likely take on even greater significance as our sophistication increases.
Respiratory Rate
Following resection of a brain tumor, patients may experience depressed respiratory drive secondary to pharmacological influences or central impairment of brainstem autonomic centers. The resultant hypercarbia and hypoxemia can increase cerebral blood volume (CBV) and CBF and potentially increase ICP.45,51 So-called pneumotactic nuclei within the pontine tegmentum appear to coordinate respiration and circulation.52,53 Compression syndromes here may contribute to a decline in respiratory function.52 The main areas of autonomic coordination of inspiration and expiration are within the reticular formation of the medulla oblongata.52,53 Sudden mass effect on these medullary centers, as in a cerebellar hemorrhage following posterior fossa surgery, can cause sudden respiratory standstill without warning.52
Hypotension |
Blood loss |
Hypovolemia |
Dieresis |
Fever/sepsis |
Adrenal insufficiency |
Hypertension |
Pain |
Agitation |
Increased ICP |
Brainstem pathology |
Hyperventilation can be associated with fever and sepsis, anxiety, drugs, and brainstem lesions. Theories include interference with cortical inhibitory influences normally exerted on the brainstem respiratory centers,54 and stimulation of these centers by either drugs54 or local pH changes secondary to brainstem tumor metabolism.55,56 The therapeutic use of hyperventilation and hypocarbia to vasoconstrict cerebral arteries, decrease CBV, and therefore lower ICP has long been known. However, there is a rationale for avoiding hyperventilation in the tachypneic patient and intervening as necessary. The danger of prolonged hyperventilation is attributed to the ability of the cerebral vasculature to adapt to the chronically low arterial partial pressure of carbon dioxide (PaCO2) and eventually vasodilate back to the baseline vessel diameter despite the low PaCO2.57 If the lower PaCO2 is elevated back to normal, the vessels will react to the “new” hypercarbic stimulus and vasodilate further. This increases CBV and ICP.51
Historically, certain classic changes in respiratory patterns were used to help localize lesions in the brains of comatose patients ( Table 44.11 ). In the postoperative setting they would indicate serious neurologic decline if acute. Cheyne-Stokes respirations follow a crescendo-decrescendo hyperventilation pattern interspersed with apnea, the result of abnormally heightened dependence on CO2 drive. It always implies bilateral damage, seen with widespread cortical injury, such as infarctions, deeper thalamic dysfunction (most likely),55 or any bilateral descending pathway disturbance from forebrain to upper pons. Metabolic disturbance can underlie the pattern, and it may provide a warning of impending transtentorial herniation.37
The following patterns can occur with brainstem injury, such as expanding posterior fossa lesions. Apneustic breathing is characterized by long end-inspiratory pauses, possibly alternating with prolonged end-expiratory pauses. Damage is localized to the mid- or caudal level pons, specifically to the pneumotactic centers involved with the coordination of respiration mentioned earlier.14 Cluster breathing appears as clusters of breaths with brief apneic pauses in between. It may result from low pontine or high medullary lesions.55 Ataxic breathing has a completely irregular pattern of deep or shallow inspiratory gasps with intermittent apnea. It is seen in agonal patients, heralds complete respiratory failure, and indicates damage to the dorsomedial aspect of the medulla.37,55
Decreased respiratory rate |
Drugs |
Brainstem pathology |
Airway compromise |
Increased respiratory rate |
Drugs |
Anxiety |
Fever/sepsis |
Brainstem pathology |
Respiratory irregularities can aid in the early identification of problems with airway maintenance, which may be compromised in any patient with a depressed level of consciousness. The tongue may fall back against the pharynx and partially or completely occlude the airway. Lower cranial nerve dysfunction after brainstem tumor surgery can also compromise airway integrity in terms of swallowing difficulties.22 Oropharyngeal or nasopharyngeal airways may avoid intubation in patients with partial airway obstruction, but concerns about aspiration may yield to endotracheal intubation.58 Such decisions should be individualized to the patient and overall clinical status, keeping in mind that, after blood loss and hypothermia, aspiration is the next greatest cause of morbidity in pediatric postoperative patients. Arriving at a diagnosis entails, in succession, direct observation, obtaining worsening arterial blood gas values and relative hypoxemia, and noting the development of fluffy infiltrates on chest radiographs, more commonly on the right side if unilateral.59
Increased Intracranial Pressure
From the preceding discussions, it can be seen that concerns regarding ICP are of primary importance in management of the postoperative patient. Early detection and institution of appropriate therapies can prevent devastating and irreversible consequences of increased ICP. Hence, a detailed discussion of the pathophysiology, recognition, and treatment strategies of increased ICP is presented.
Monro-Kellie Doctrine
The Monro-Kellie doctrine is often utilized to partially explain the dynamics of increased ICP.60,61 The skull is depicted as a rigid container with no distensibility and three compartments, each filled to capacity with brain tissue, blood, or CSF. The addition of any mass to either compartment must be balanced by the removal of an equivalent amount from elsewhere within the container or the pressure within the entire system will rise. The initial compensatory mechanism to offset a rise in ICP is displacement of CSF, either into the CSF spaces of the spine or into the venous drainage system of the brain. If the ICP is still high, venous drainage from the head ensues, until either the ICP is normalized or the overall buffering capacity of these two compartments is exhausted.45 It then becomes easy to conceptualize how hydrocephalus, for example, can cause increased ICP: volume is added to the CSF compartment. Likewise, cerebral vasodilatation can expand the blood volume, and edema, hemorrhage, or tumor can augment parenchyma. Most sources regard the patient with an open fontanel or split sutures as an exception to the Monro-Kellie doctrine, yet even the dural container should at some point behave as an unforgiving confine if faced with progressive volume expansion.
A simplified pressure-volume curve is illustrative of this concept ( Fig. 44.1 ).62 At lower pressures, increases in volume have little effect on ICP, as the compensatory mechanisms described above offset the added volume. As more volume is added, however, compensatory mechanisms are exhausted and ICP rises rapidly. The slope of the curve is steeper in infants than in adults63; hence, even relatively small increases in volume are poorly tolerated and cause a rapid increase in ICP in very young patients.
Cerebral Hemodynamics
A discussion of some cerebral hemodynamic neurophysiology is required before approaches to the bedside can be entertained. The negative effects of increased ICP are in part due to global ischemia, which develops as cerebral perfusion declines and the metabolic demands of the brain are no longer being met.41 The cerebral metabolic rate of oxygen (CMRO2), a measurement of brain metabolism, is closely coupled to CBF. If CMRO2 is high, as during seizure activity, CBF will be correspondingly high. If the CMRO2 is low, as during sleep, CBF will decrease.64,65 CBF, to effect delivery of oxygen and nutrients, is in turn dependent on CPP, defined earlier as the difference between MAP and ICP. CPP can therefore be compromised by either a decrease in MAP or an increase in ICP.
Autoregulation is the ability of the brain to maintain an adequate CBF to meet metabolic demands despite fluctuations in MAP or ICP. CBF will remain nearly constant over a wide range of CPP values, allowing adequate delivery of O2 and nutrients ( Fig. 44.2 ). This is mainly accomplished by vasoconstriction and vasodilatation of cerebral arterioles, which have the capacity to increase their diameter by 200 to 300%, subserving a potential increase in blood volume of 400 to 900%.51 In other words, CPP is the driving force behind CBF, and autoregulation, via changes in cerebral arterial tree resistance, permits fluctuations in MAP or ICP without significant alterations in CBF and substrate delivery.66
Under pathological conditions, the compensatory mechanisms that buffer increases in ICP by displacement of CSF and venous blood are already limited, and changes in arteriolar vessel diameter can then significantly affect ICP. For example, in response to a decrease in CPP, autoregulation demands the cerebral arterioles vasodilate to maintain CBF. This in turn leads to an increase in CBV and a subsequent rise in ICP. The vasoconstriction and vasodilatation cascades are models developed by Rosner that may explain some of the cerebral hemodynamic interactions seen in patients with increased ICP ( Fig. 44.3 ). The models permit conceptualization of treatment paradigms and conditions that may positively or negatively affect ICP in patients with intact autoregulation and allow us to understand why some authorities promote maintenance of CPP as the focus of therapy independent of ICP. For example, a pharmacologically induced elevation of MAP will increase CPP. Autoregulation then responds with cerebral arteriolar vasoconstriction to maintain a steady CBF, which leads to a lower CBV. This in turn results in a lower ICP.67


Symptoms and Signs
The symptoms and signs of increased ICP represent a spectrum that may differ relative to the rate of formation, pathology, and baseline neurologic status of the patient. Complaints such as headaches, nausea, and visual disturbances may be heard with the onset of increased ICP, yet may not be apparent in the very young, lethargic, or noncommunicative patient. These same subjective symptoms may be fleeting or absent in the patient who rapidly deteriorates and promptly manifests signs of brainstem involvement. Symptoms and signs may therefore develop secondary to global increases in ICP, or regional increases, with focal compression of specific structures. The majority of postoperative patients will not have increased ICP upon arrival at the ICU, having just had their mass lesion decompressed or having had normal ICP preoperatively. The development of increased ICP in this setting can be acute, as with hemorrhage into the resection bed, or delayed, as in the development of edema. Peak edema formation typically occurs 36 to 72 hours postoperatively, but clinical signs may manifest sooner, within 6 to 12 hours.51
Patients may experience cyclical rises and falls in ICP during which they may have impaired consciousness, complain of headaches, or experience nausea and vomiting.41,68 Described as plateau waves by Lundberg,68 they can occur sporadically throughout the day or occur in clusters, lasting for 5 to 20 minutes per episode. The vasodilatation and vasoconstriction cascades help to explain the cyclical nature of these symptomatic ICP spikes ( Fig. 44.3 ). The models show how a decline in CPP can perpetuate such a pressure wave and elevate the ICP. The initial stimulus is likely a drop in systemic blood pressure and MAP.69 This continues until vasodilatation is maximal (the lower limit of autoregulation) or a stimulus increases the CPP, such as a subsequent rise in MAP. Vasoconstriction then lowers the ICP51 ( Table 44.12 ).

Signs of brainstem involvement imply progression to a herniation syndrome. The pupillary irregularities and anisocoria seen with transtentorial herniation are indicative of oculo motor palsy from midbrain compression rather than direct pressure on the third cranial nerve as previously thought.70 Hemiparesis or hemiplegia may also be due to midbrain dysfunction, though weakness can also be caused by supratentorial hemispheric lesions. Laterality of the deficit and location of the tumor resection bed may help in discerning the difference. False localizing signs may be confounding, however ( Fig. 44.4 ). Loss of consciousness occurs as a result of brainstem reticular activating system dysfunction,45 and cardiorespiratory abnormalities may ensue with medullary involvement. Abnormal flexion or extension motor responses to stimulation are usually late events. Papilledema, although a sensitive sign of increased ICP, is probably less helpful in this setting because it is usually not present with acute deterioration, nor is its presence significant in patients who had significant mass effect preoperatively.
Symptoms |
Headache |
Nausea |
Vomiting |
Mental status changes |
Agitation |
Signs |
Fontanel tension |
Papilledema |
Brainstem involvement |
Papillary irregularities or anisocoria |
Hemiparesis or hemiplegia |
Loss of consciousness |
Vital sign abnormalities |
Abnormal motor responses (“posturing”) |
Radiographic Studies
Once suspicions have been raised that ICP is increased, whether on clinical grounds or via a functioning ICP monitor, or a new focal deficit manifests, the patient is usually transported to the computed tomography (CT) scanner for imaging. CT is chosen over magnetic resonance imaging (MRI) because it is quicker, cheaper, easier in terms of systemic monitoring of the patient during the study, and yields adequate detection of hemorrhage or edema formation in most cases.

Compression or obliteration of the basal cisterns |
Midline shift |
Loss of sulci against inner table of the skull |
Loss of the gray matter–white matter interface |
Compression of lateral ventricles |
Hemorrhagic mass |
Hydrocephalus |
Rounded third ventricle |
Temporal horns |
Ventriculomegaly |
Preoperative monitor/ventriculostomy |
Intraoperative considerations |
Observation of edema formation |
High index of suspicion for edema formation/prolonged retraction |
Limited neurologic exam |
Prolonged anesthesia/inability to extubate |
Heavy sedation/pharmacological paralysis |
Mechanical ventilation with high mean airway pressures |
Neurologic deterioration |
Radiological evidence |
Edema |
Hydrocephalus |
Hemorrhage |
There is increasing data on the cumulative lifetime cancer risks for children exposed to the ionizing radiation, including in the form of a head CT.71–73 Although the numbers represent fairly small individual risks, children are more sensitive to radiation than adults74; they have more dividing cells and have more time to express a cancer.72 This estimated lifetime cancer risk drops precipitously with age, but can be as high as one case for every 1,000 and one case for every 2,000 scans in neonates and young children, respectively.72,73 These risks should be taken into account whenever a head CT is ordered on any patient; frequent scanning is to be avoided unless clinically indicated.
Computed tomography findings that have been correlated with increased ICP in head trauma75–77 are also applicable to patients with nontraumatic coma78 ( Table 44.13 ). Compression or obliteration of the basal cisterns appears to be the most sensitive finding correlated with increased ICP.65,75 Midline shift is also significant.77 Loss of sulci against the inner table of the skull and loss of the gray matter–white matter interface may also be noted. If no hemorrhage or hydrocephalus is seen, and evidence of edema is noted, therapy is instituted. Hydrocephalus mandates emergency placement of a ventriculostomy catheter for decompression. A new hemorrhagic lesion may or may not be surgical, depending on several factors, including size, location, mass effect, and clinical status of the patient. In general, postoperative bleeds large enough to cause mass effect in neurologically deteriorating salvageable patients are emergently evacuated immediately after the scan.
Intracranial Pressure Monitoring
The direct measurement of ICP allows for early detection of upward trends or acute increases in ICP, as well as the ability to gauge the efficacy of therapies aimed at attenuating high ICP. In the absence of (or prior to) the development of increased ICP, monitoring also offers assurances that ICP is maintained within a normal range during surveillance. The ICP monitor should be thought of as a means of measuring CPP and of ensuring its adequacy to maintain CBF, because the ultimate consequence of insufficiency is ischemia and potentially irreversible damage.
There are two ways to adequately monitor ICP: placement of an intraparenchymal ICP monitor, or placement of an external ventricular drain (EVD). The latter, in addition to measuring ICP, has the added benefit of enabling drainage of CSF, which is one of the first steps in the pathway for treating increased ICP.79 A recent comparison of the two modalities found that measurements of ICP by EVD and by intraparenchymal monitor were highly correlated.80
Indications
There are several indications to monitor ICP following surgery for brain tumors, most based on the premise that increases are detectable prior to the development of neurologic symptoms and signs45 ( Table 44.14 ). If the child required ICP monitoring prior to surgery, the monitor should be left in place postoperatively until normalization of ICP is verified. Likewise, if a child did not require an ICP monitor preoperatively, then it is usually not necessary to place one after uncomplicated supratentorial or infratentorial tumor surgery. However, observations of brain swelling intraoperatively or concerns regarding the risk of brain swelling due to prolonged retraction for example should lead to ICP monitor placement prior to leaving the operating room. Inability to reliably follow the neurologic status of a child requires ICP monitor placement. This may be secondary to prolonged surgery and inability to extubate, or to a requirement for heavy sedation or paralysis. Mechanically ventilated patients with high mean airway pressures, raised not only by positive endexpiratory pressure (PEEP) but also by peak airway pressure or inspiratory time, can transmit pulmonary pressures to the CSF column (via the vertebral venous plexus) and to the jugular vein (through the superior vena cava).81 These patients are at risk for development or exacerbation of increased ICP and should be monitored. ICP should also be followed in patients who deteriorate neurologically, especially if found to have edema on CT.
Treatment of Increased Intracranial Pressure
The normal range of ICP increases with age. Reported values for normal newborn term infants range from subatmospheric (less than zero) to mean pressures of approximately 5 to 10 cm H2O or lower.82–92 Data obtained within the past 30 years, primarily via noninvasive monitoring over the anterior fontanel, are in general agreement with reports from the 1920s and earlier of CSF pressures in infants.63 Transfontanel noninvasive readings are affected by application force on the sensor,93 and Table 44.15 shows selected reports of normal neonatal and infant ICP that correlated noninvasive readings with direct intracranial or lumbar measurements. After infancy there is a gradual rise through early childhood to pressures lower than 15 cm H2O in the older child and adult.88,94,95 Figure 44.5 presents an example of the normal ICP range through childhood, obtained by recordings performed in 147 infants and children over a period of years.88
With such a wide reported range, specific threshold values above which treatment to lower ICP is initiated vary among different centers. In addition, transient increases in ICP do not always correlate with worse outcome, and neurovascular compression syndromes secondary to local mass effect may occur without appreciable increases in global ICP. Therefore, even age-related treatment thresholds are not likely to be as relevant as decision trees that incorporate overall neurologic status and radiological findings.96 In addition, in the absence of signs of herniation, therapies to maintain an adequate CPP may be appropriate despite high ICP. Several groups have measured CPP in newborn term and preterm infants, with mean values of 34 to 39 mm Hg.87,97,98 Optimal CPP treatment thresholds of 40 to 65 mm Hg as an age-related continuum have been advocated in children and adolescents.79,99,100
Treatment modalities, largely extrapolated from pediatric traumatic brain injury literature, have been divided into first-and second-tier therapy in the critical pathway for treatment of elevated ICP.79 The traditional modalities make up the first tier and include sedation, analgesics, head-of-bed elevation, CSF drainage if available, chemical paralysis, hyperosmolar therapy, and avoidance of prolonged hyperventilation and hypotension. Second-tier therapy includes lumbar drainage, decompressive craniectomy, high-dose barbiturates, and moderate hypothermia79 ( Table 44.16 ).

Position and Elevation of Head
The effectiveness of this simplest of maneuvers in lowering an increased ICP has long been known. The effect is so rapid that a brief lowering of the head of the bed is frequently done to ensure that a recently placed ICP monitor is functioning properly. The rationale is that facilitating cerebral venous outflow into the systemic circulation lowers cerebral venous blood volume and therefore reduces ICP. Likewise, jugular venous outflow can be impeded by head rotation; therefore, a neutral midline position should always be maintained, and the use of constricting endotracheal tube tape should be avoided.101–103
However, concerns regarding the possibility of head elevation causing a decrease in CPP have been raised. Several studies have not found a significant drop in CPP unless the head is elevated more than 30 degrees,104,105 whereas others have provided evidence that CPP is maximal when patients are flat (zero degrees) despite a higher ICP in this position.106,107 In addition, the ICP will increase in up to one third of adults when the head is elevated, likely due to activation of the vasodilatation cascade as a result of decreased CPP.51 A study that measured CBF concluded that head elevation to 30 degrees reduced ICP in the majority of patients without significantly compromising mean CPP or CBF, yet a significant decrease in CBF occurred in 23% of patients.108 Some groups therefore favor the supine position due to the belief that it optimizes CPP.109 Others have suggested that optimal head positioning should be established individually in patients with high ICP owing to an unpredictable response.110
First-tier therapy |
Sedation |
Analgesics |
Head of bed elevation |
Cerebrospinal fluid drainage |
Chemical paralysis |
Hyperosmolar therapy |
Avoid hyperventilation and hypotension |
Second-tier therapy |
Lumbar drainage |
Decompressive craniectomy |
Barbiturate therapy |
Moderate hypothermia |
There are few objective data addressing this issue specifically in children. In one study, newborn infants had significantly lower ICP at 30 degrees, as compared with the flat or dependent head positions.111 We currently maintain a head elevation of 30 degrees in postoperative patients at risk for or with evidence of increased ICP, while recognizing that decreases in blood pressures are less likely in patients with adequate volume status. Head-of-bed elevation has also been implemented in care bundles shown to reduce the incidence of ventilator-associated pneumonia in pediatric ICU care.112
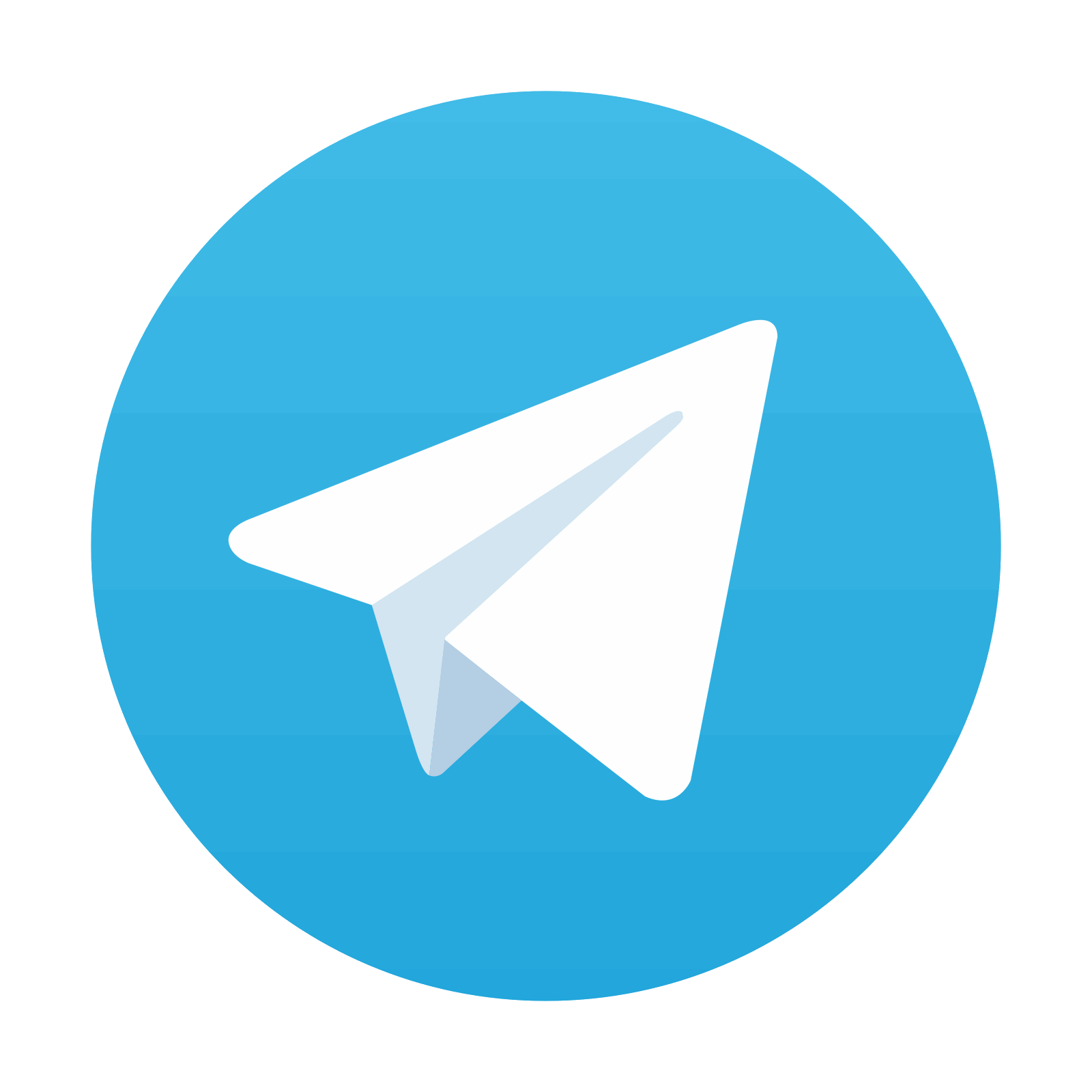
Stay updated, free articles. Join our Telegram channel

Full access? Get Clinical Tree
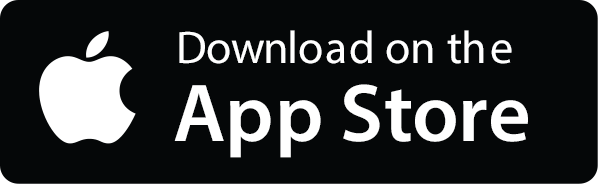
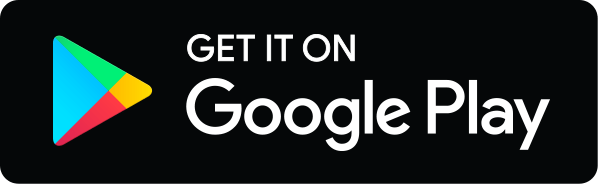
