31 Postoperative Rehabilitation of Lumbar Spine Patients
Lawrence W. Frank
Postoperative rehabilitation of lumbar spine patients seems like an intuitively good idea. However, the details of rehabilitation programs are a mystery for most spine physicians, and deemed unimportant by others.1–3 Fueling these attitudes is a paucity of quality research supporting rehabilitation outcomes, particularly in postoperative patients. Recent research into specific exercise programs on well-defined patient groups is showing promising results.4 In this chapter, we will address the available outcome studies on this topic, rehabilitation-related biomechanics of the lumbar spine, rehabilitation principles and goals for the postoperative patient, and example programs built upon the rational application of postoperative biomechanics.
Timing and Efficacy of Postoperative Rehabilitation
Lumbar Discectomy
It is generally accepted that the postoperative rehabilitation of discectomy patients is begun 4 to 6 weeks postsurgery. There is support, however, for early postoperative rehabilitation. One study investigating an immediate postsurgical rehabilitation program revealed lessened leg pain and improved lumbar range of motion (ROM) compared with a control group at short-term follow-up.5 A prospective study of an individualized neuromuscular exercise program started 2 weeks postsurgery had superior outcome on disability scales than a traditional program started 6 weeks postoperatively.6 At 4 to 6 weeks postoperatively, there is strong evidence that intensive exercise programs produce faster short-term improvement of function and return to work rates versus mild intensity programs.7–9 Additionally, none of the rehabilitative treatments produced significant rates of reherniation or reoperation.6,9
Lumbar Fusion
Postsurgery exercise programs for fusion patients are typically commenced at approximately 3 months to allow adequate bony union. There are no studies comparing early versus standard timing for postsurgical rehabilitation of fusion patients. The few studies assessing the efficacy of postoperative rehabilitation are supportive but also reveal that social support groups are equally if not more effective at 2-year follow-up for pain scores, functional tasks, and return to work outcome measures.10,11 Like many studies assessing rehabilitation outcomes, these studies are limited in that they assess a “one size fits all” program applied to nonhomogeneous patient groups.
Rehabilitation Biomechanics
To best understand lumbar spine rehabilitation, it is necessary to understand the functional biomechanics of the spine. The vertebral joints, discs, and ligaments limit end range spinal motion. These structures are the passive stabilizers of the lumbar spine and are susceptible to injury if normal spinal ROM is exceeded. On the other hand, muscles provide dynamic spinal stabilization by prepositioning the spine to avoid nonphysiologic ROM and contracting in preparation for large anticipated loads.12,13 Thus, muscles protect the joints, discs, and ligaments. It follows that insufficient strength or endurance of this protective musculature is the primary preventable cause of spinal injury.
Spinal rehabilitation programs are primarily directed at optimizing muscular control of the spine. Muscles control joint movement during either shortening (concentric) or lengthening (eccentric) contractions. When considering the functional biomechanics of the spine it is important to consider that acute injury occurs most commonly in the standing or bending posture or during gait. Human gait has sometimes been described as a “controlled fall,” and is biomechanically characterized by a fair degree of eccentric muscle contraction.14 Eccentric muscle contraction produces higher tension within muscles and tendons than concentric contraction,15 and thus places the muscle at greater risk of injury or failure. It follows that the greatest risk of injury occurs in activities when eccentric muscle strength is overcome, and that spinal rehabilitation programs must include eccentric strength training to protect against future injury.
Control of Lumbar Sagittal Balance
Spine biomechanics may be explored with the goal of controlling normal lumbar curvature during erect posture. It is clearly evident to spine specialists that the lumbar spine exhibits a physiologic sagittal lordosis (Fig. 31.1). In any orthopedic problem, it is important to assess the ROM of structures above and below an injured region. This is of interest to determine if undue forces from adjacent structures are being applied to the injured region. In this light, motion of the lumbar spine is intimately linked with thoracic spine mechanics and ROM of the pelvis and hip. For example, a fixed thoracic kyphosis will produce compensatory lumbar hyperlordosis in the erect standing posture, increasing mechanical forces on the posterior elements of the lumbar spine (Fig. 31.2). Also, limited hip flexion due to hamstring contracture produces a secondary abnormal flexion moment on the anterior elements of the spine in positions of forward flexion, i.e., bending to pick an object off the ground (Fig. 31.3).
Cadaveric studies have shown that static stabilizers such as ligaments and bones contribute minimally to the stability of the vertebral column when the musculature is removed.16 Musculature is thus a major determinant of lumbar curvature. Observations of muscle imbalance about the spine and pelvis have revealed characteristic patterns of muscle shortening and weakness in humans (Table 31.1).17 The cause for these imbalances may be under central nervous system control.18 The sum effect of these muscle imbalances is the tendency in humans for exaggerated lumbar lordosis in erect standing posture.
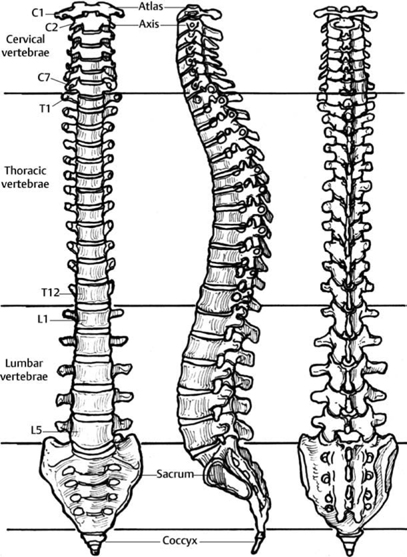
Fig. 31.1 The lumbar spine exhibits physiological lordosis in the sagittal plane.
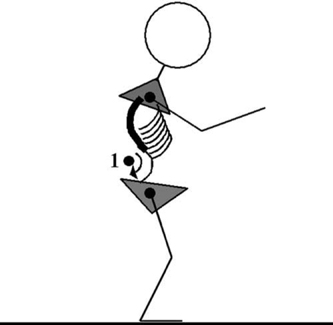
Fig. 31.2 A fixed thoracic hyperkyphotic deformity (bold) produces a compensatory lumbar hyperlordosis (1) in erect standing posture. Patients compensate for excess load on the posterior elements with hip and knee flexion.
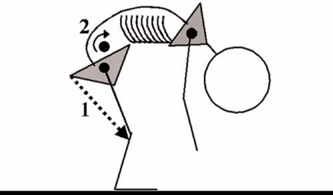
Fig. 31.3 Shortened hamstrings (1) prevent physiologic pelvic flexion over the hips during forward bending. This produces an excessive kyphotic force upon the lumbar spine (2). Patients compensate for hamstring tightness with knee flexion, increased thoracic kyphosis, and shoulder protraction when attempting to touch the floor.
Shortened Muscles | Weak Muscles |
Hamstrings | Gluteus maximus |
Rectus femoris | Rectus abdominis |
Iliopsoas | |
Erector spinae |
Muscles Exhibiting Tendency toward Shortening
Iliopsoas
The psoas portion of the iliopsoas complex originates from the transverse processes of T12 through L5 and crosses the pelvis and hip before attaching to the lesser trochanter of the femur (Fig. 31.4). Concentric contraction of the iliopsoas muscle is associated with increases in lumbar lordosis in experimental subjects19 as well as hip flexion. The eccentric function of this muscle is to prevent uncontrolled hip extension. Shortening of this muscle mimics concentric contraction, and is associated with hyperlordosis (Fig. 31.5), secondarily causing increased mechanical force upon the posterior elements.20–23
Rectus Femoris
The rectus femoris originates from the anterior inferior iliac spine and crosses the hip before inserting into the patella via the common quadriceps tendon (Fig. 31.6). It is a “two joint muscle” and its effect on the hip and pelvis are realized only with the knee in full extension, as in static standing. Concentric contraction of the rectus femoris produces hip flexion and knee extension. In static standing with the knee fully extended, shortening of this muscle produces flexion of the pelvis over the femoral heads, producing a secondarily exaggerated lumbar lordosis (Fig. 31.5) and again, increased mechanical force upon the posterior elements. The eccentric function of this muscle about the hip is to prevent uncontrolled hip extension, although this effect is diminished when the knee is flexed, as in the swing phase of gait.
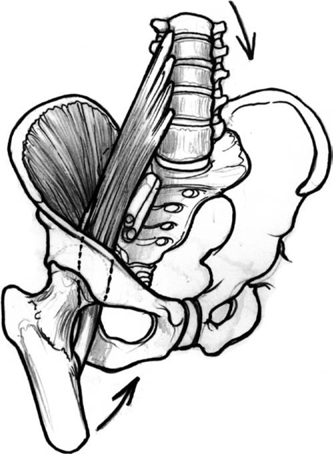
Fig. 31.4 Concentric iliopsoas contraction produces lumbar lordosis and hip flexion. Eccentric contraction controls hip extension.
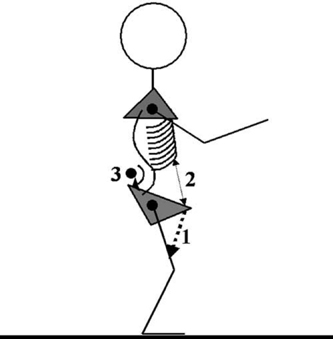
Fig. 31.5 Hyperlordosis of the lumbar spine (3) in erect standing posture may be caused by contracture of the hip flexors (1), weakness of the rectus abdominis (2), or both.
Hamstrings
The hamstrings consist of the semimembranosus, semi-tendinosus, and biceps femoris long and short heads. Like the rectus femoris, this is a two joint muscle, and its effect on the hip and pelvis are realized only with the knee in full extension, as in static standing. The hamstrings originate from a common tendon off the ischial tuberosity and insert into the medial proximal tibia and posterior capsule of the knee (Fig. 31.7). Concentric contraction of the hamstrings produces hip extension and knee flexion. In static standing with the foot planted and knee fully extended, shortening of this muscle produces extension of the pelvis over the femoral heads, limiting hip flexion during bending at the waist, producing a secondarily exaggerated lumbar kyphosis (Fig. 31.3). This kyphotic force places increased mechanical force upon the anterior column of the spine, including the disc.24 This is one of the reasons that it is recommended to bend at the knees when picking objects off the floor. The eccentric function of this muscle about the hip is to prevent uncontrolled hip flexion,25 but this effect is markedly diminished when the knee is flexed, as in the swing phase of gait.
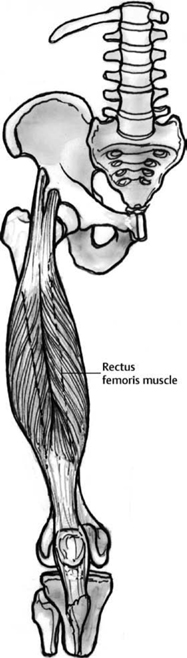
Fig. 31.6 Rectus femoris concentric contraction produces hip flexion and knee extension. Its eccentric function about the hip and pelvis is limited by a short lever arm and maximized with the knee in full extension.
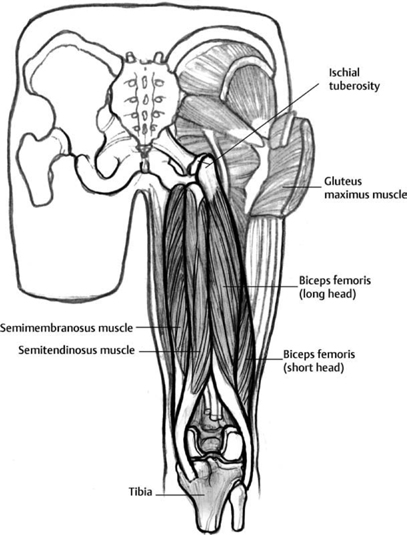
Fig. 31.7 Concentric contraction of the hamstrings produces hip extension and knee flexion. Its eccentric role about the hip in erect posture is to guard against uncontrolled pelvic flexion over the hips.
Muscles Exhibiting Tendency toward Weakness
Rectus Abdominis
The rectus abdominis originates from the anterior ribs and traverses the anterior abdominal wall, attaching into the symphysis pubis (Fig. 31.8). Concentric contraction of the rectus muscle produces lumbar flexion, reducing lordosis. The eccentric function of this muscle is to prevent uncontrolled lumbar extension.26,27 Eccentric weakness of this muscle produces hyperlordosis by allowing flexion of the pelvis over the hips in erect posture (Fig. 31.5), secondarily causing increased mechanical force upon the posterior elements.
Gluteus Maximus
The gluteus maximus originates from posterior iliac crests and thoracolumbar fascia and inserts into the greater trochanter and iliotibial band (Fig. 31.9). Concentric contraction of the gluteus maximus muscle produces hip extension, and it is the most powerful of the hip extensors. The eccentric function of this muscle is to prevent uncontrolled hip flexion.28 Eccentric weakness of this muscle (and the hamstrings) during dynamic forward flexion causes the secondary activation of the erector spine29 and other paraspinals to prevent hyperflexion of the trunk. As the paraspinal muscles have significantly less contractile strength than the gluteus, the paraspinals are subject to failure, leading to muscle sprain or injury to the anterior column structures, including the disc.
Thoracolumbar Fascia
The thoracolumbar fascia controls lumbar lordosis by its attachment to the lumbar spinous processes (Fig. 31.10). Tension of the thoracolumbar fascia is provided by the pull of the transversus abdominis and latissimus dorsi on the edges of the fascia,30 and maintains normal lordosis by tending to align the spinous processes in the plane of the fascia. Weakness of the attached muscles allows hyperlordosis due to laxity of the thoracolumbar fascia.
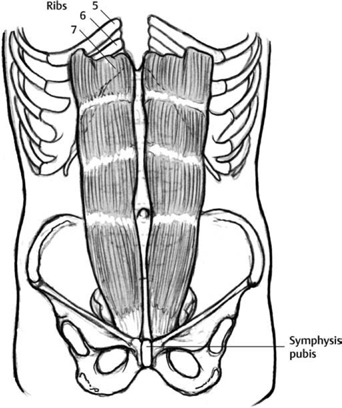
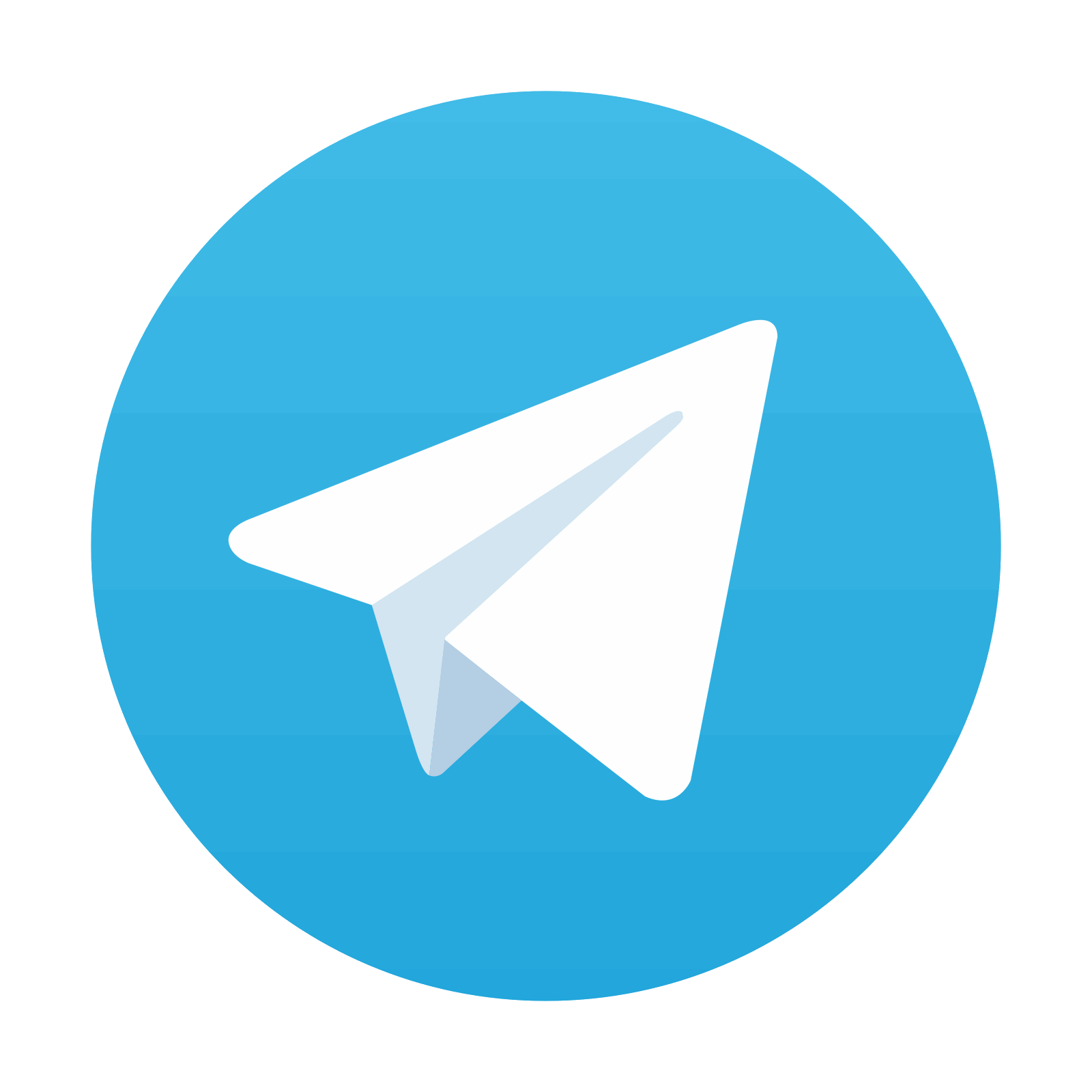
Stay updated, free articles. Join our Telegram channel

Full access? Get Clinical Tree
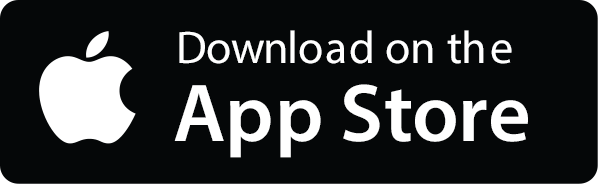
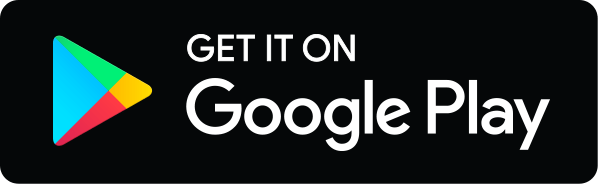