ADAPTIVE PLASTICITY
Even if lost cells are not replaced, spared neurons and fibres retain a considerable degree of plasticity to adapt to lost connections by adaptive biochemical compensation both at the presynaptic level – for example by changes in cell firing, transmitter synthesis, release and reuptake – and postsynaptically, by changes in receptor density, receptor sensitivity and post-synaptic signalling (Figure 7.1C)2,3.
The first clear evidence that Cajal’s dictum was overly pessimistic in relation to the mammalian CNS came from the demonstration that if a septal cell loses some of its normal axonal inputs then other afferent axons can sprout into the vacated spaces to form new synaptic connections with the target cell4. If the new inputs are from a different source they are by and large not functional. However, collateral sprouting of spared fibres of the same systems do appear to be able to sustain functional recovery in a number of model circuits of the brain (Figure 7.1D)3,5,6.
A major problem for extensive axonal reorganization in the adult brain is that although axons can undergo a degree of local sprouting, they do not typically retain the developmental capacity for longdistance growth through the CNS to distant targets (Figure 7.1E)3,7. Long-distance growth of axons can nevertheless be promoted by providing an alternative substrate for growth such as Schwann cells from the peripheral nervous system (PNS) which can be used to bridge a gap caused by a lesion cutting a pathway, or be implanted as a track along which new axons can grow (Figure 7.1H)8.
When essential populations of neurones are lost they may be replaced by transplantation. The techniques are now well established for transplantation of embryonic cells derived from the CNS into the brain of adult or aged experimental animals (Figure 7.1G), and such grafts have been demonstrated to survive, repopulate areas of denerva- tion, replace deficient innervations, and restore lost functions in a wide variety of model systems9,10. There is now compelling clinical evidence that such grafts can provide a substantial alleviation of symptoms in Parkinson’s disease11, and clinical trials are now underway in Huntington’s disease, spinal cord injury and stroke12-14. It remains a matter of speculation whether neuronal transplantation can alleviate the more diffuse and widespread degeneration associated with ageing and the dementias.
Neuronal connections are dependent upon trophic support from their targets, and neurodegenerative diseases of ageing may in part be attributable to a decline in growth factor support15. Thus, an alternative approach to prevention of progressive neurodegeneration, and to induction and guidance of regenerative axon growth, is to apply or replace identified trophic factors explicitly. For example, dopaminergic neurones, which decline in several neurodegenerative diseases of ageing, are dependent upon trophic factors (including brain-derived neurotrophic factor (BDNF) and glial cell-derived neurotrophic factor (GDNF)) for trophic support. Chronic infusion of GDNF into the striatum or midbrain can inhibit progressive lesion and age-related atrophy of midbrain dopamine neurones and block the functional decline in motor abilities (Figure 7.1F) both in experimental animals16 and in man17. A similar strategy involving chronic central infusions of nerve growth factor (NGF) has been attempted in a pilot experiment in Alzheimer’s disease with only modest success18
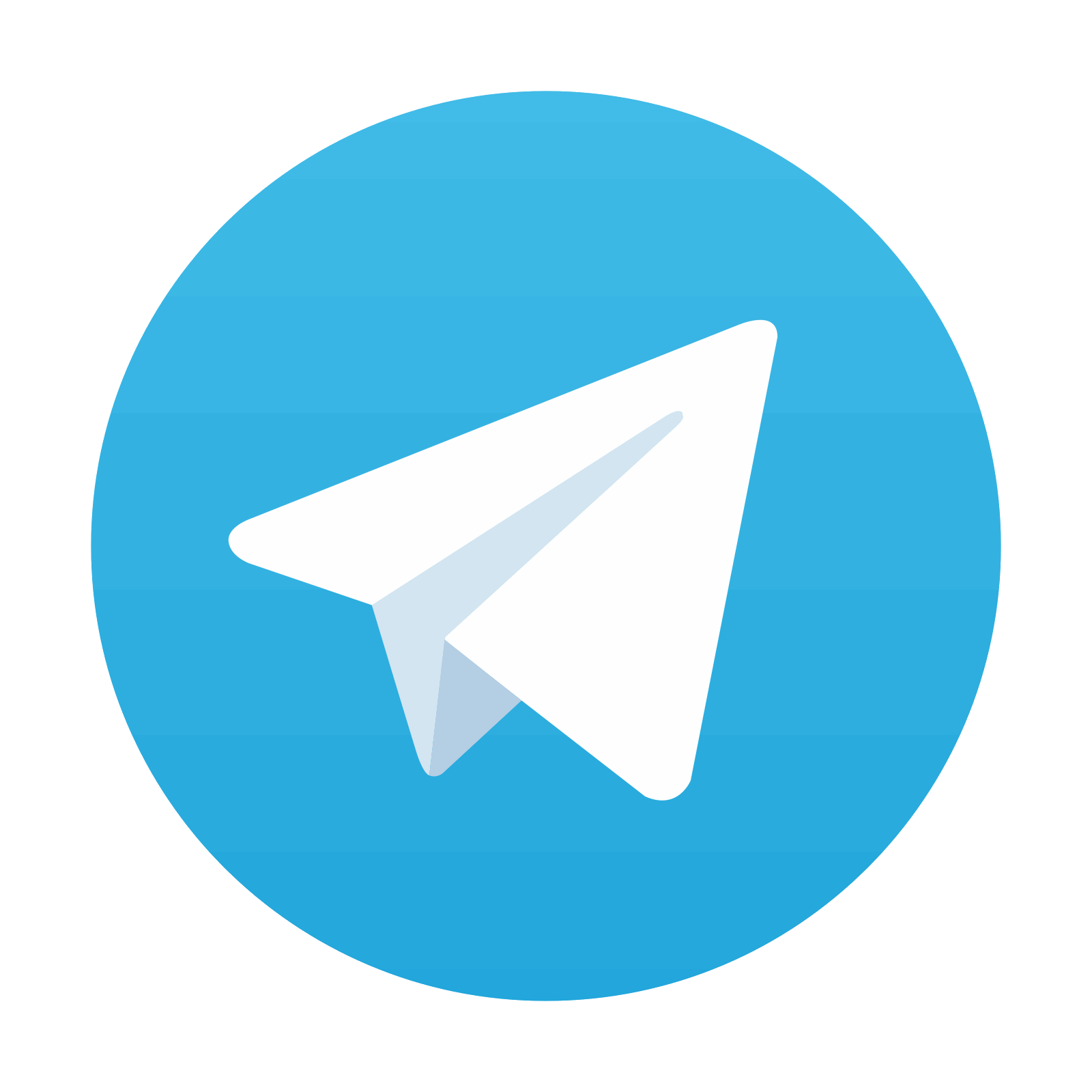
Stay updated, free articles. Join our Telegram channel

Full access? Get Clinical Tree
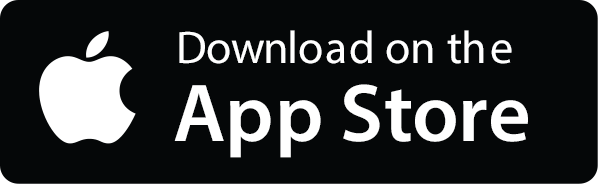
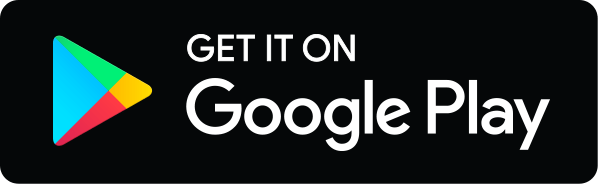