16 During the initial evaluation of a cranial or spinal lesion, the degree of vascularity should always be considered. Careful assessment of the preoperative neuroimaging and patient history should highlight the possibility of a vascular lesion and significant intraoperative blood loss. If a vascular lesion is included in the differential diagnosis, the neurosurgeon should consider currently available tools for addressing and facilitating hemostasis prior to bringing the patient to the operating room. The primary tool currently available in the preoperative setting is interventional neuroradiology embolization techniques. With the continued development of better X-ray equipment, catheters, wires, and embolic materials, a growing proportion of cranial and spinal lesions are now amenable to embolization. The majority of preoperative embolization procedures utilize a transarterial approach. However, in select cases, percutaneous embolization techniques may also be employed.1–6 In addition to reduced intraoperative blood loss, the potential benefits of preoperative embolization include (1) preoperative selective control of surgically inaccessible arterial feeders, (2) reduced operative time, (3) increased probability of complete resection, (4) decreased risk of injury to adjacent neural tissue, (5) possibly reduced lesion recurrence rates, and (6) improved definition of abnormal anatomy and overall visualization of the surgical field.7,8 This chapter provides an overview of the techniques utilized for preoperative embolization and the most commonly employed embolic agents, briefly describes the most common cranial and spinal lesions targeted for preoperative embolization, and discusses important complications and risks to be cognizant of in each case. Importantly, preoperative embolization is only an adjunct to the definitive surgical procedure, and therefore, with each individual case, a risk/benefit analysis must be conducted to ensure that the above-mentioned benefits of embolization outweigh the specific and potentially catastrophic risks of each case. The transarterial embolization procedure can be divided into three stages: an access and diagnostic stage, a selective microcatheter stage, and an embolization stage. Prior to obtaining arterial access, the question of anesthesia must be addressed. Embolization procedures performed on an awake or mildly sedated patient enables continuous neurologic monitoring and provocative testing, while avoiding the risks of general anesthesia (GA). On the other hand, the advantages of GA include the elimination of patient discomfort and patient movement during critical diagnostic or embolization portions of the procedure. The main drawback of GA is the inability to perform real-time neurologic exams. However, neurophysiological monitoring such as electroencephalography (EEG), somatosensory evoked potentials (SSEPs), and motor evoke potentials (MEPs) can be used as a substitute for a neurologic exam.9–11 The authors typically perform all intracranial and extracranial head and neck embolization procedures under GA. With spinal embolization procedures, moderate sedation is sufficient except for cases such as metastatic thoracic spine lesions that are complicated by patient movement from respiration or discomfort secondary to the instability pain commonly associated with these lesions. Cannulation of the arterial system typically involves the common femoral artery but may in certain cases instead entail the brachial, radial, or carotid artery. After insertion of an arterial sheath, a variety of diagnostic catheters may be advanced to the appropriate vessel to perform the diagnostic angiogram. The goal of the diagnostic stage is to define the general vascular anatomy of the lesions including the degree of vascularity, arterial supply, and venous drainage. Importantly, the initial diagnostic angiogram should include a preintervention view of the vascular anatomy, which can be used for comparison postintervention. For example, in the case of extracranial head and neck tumors, this includes performing selective internal carotid artery (ICA) angiograms so that if there is concern about a thromboembolic complication, a postintervention ICA angiogram can be analyzed for missing branches or changes in flow. After the diagnostic angiogram is performed, the diagnostic catheter is replaced with a guide catheter. The purpose of the guide catheter is to provide a stable platform for smaller, softer microcatheters to pass through. As a result, these catheters are usually stiffer and have a larger inner diameter than diagnostic catheters. With straightforward anatomy, guide catheters can generally be directly re-advanced into the desired vessel. In the case of tortuous or complex anatomy, the diagnostic catheter that is already in position can be exchanged for the guide catheter over a long exchange wire. In some procedures, the diagnostic catheter can be employed as a guide catheter. In the case of spinal embolizations, the authors commonly use a 5-French Cobra 2 diagnostic catheter (Merit Medical Systems, Inc., South Jordan, UT), which has a tip designed to cannulate transversely oriented vessels such as spinal segmental arteries. The inner diameter is large enough to allow a microcatheter through, and the shape of the Cobra 2 catheter tip helps maintain its position in the segmental artery. The guide catheter is connected to a continuous heparin saline flush via a rotating hemostatic valve to prevent thrombus within the catheter but is not sufficient to affect clotting parameters.12 Systemic anticoagulation with heparin is commonly used to reduce thromboembolic complications.13 For embolization procedures, the authors most commonly administer a dose of heparin 70 IU per kg with a goal of two times the baseline activated clotting time. The next stage of the embolization procedure is the selective microcatheter stage. The goal of this stage is to advance the microcatheter to a position that enables the safe and effective delivery of embolic material to the targeted lesion. The first step of this stage is choosing the appropriate microcatheter. There is a large assortment of microcatheters currently available. The general design of these microcatheters incorporates a stiffer, larger proximal end that tapers into a softer, smaller distal portion. The stiffer, proximal portion enables the catheter to be advanced forward while the more flexible, distal portion enables the catheter to navigate small, distal vessels with decreased risk of vessel rupture or dissection. Microcatheters are braided, which prevents kinking of the catheter when it traverses sharp turns. A hydrophilic coating around the catheter reduces friction between the microcatheter and the guide catheter, and between the microcatheter and the vessel wall. There is some evidence that the hydrophilic coating may reduce the risk of thrombus formation along the catheter.14 The general technique of advancing microcatheters is to do so coaxially over a microwire, with the less traumatic wire in front. A special subset of microcatheters that can be advanced in a different manner is the flow-directed microcatheter. The Magic microcatheter (Balt Extrusion, Montmorency, France) and the Marathon microcatheter (ev3, Plymouth, MN) are flow-directed catheters that have extremely soft, small distal tips. With these catheters, the microwire is retracted into the lumen of the catheter while the soft distal tip is carried forward and directed by blood flow, enabling the catheter to be navigated into extremely small vessels with a very low risk of vessel injury. Microcatheter tips may be pre-shaped out of the box or may be custom shaped with steam to facilitate navigation. Lastly, prior to selection of a microcatheter for an embolization procedure, the compatibility with specific embolic materials must be taken into account. The liquid embolic Onyx (ev3, Plymouth, MN) is dissolved in dimethyl sulfoxide (DMSO), and therefore only DMSO-compatible microcatheters such as the Marathon microcatheter can be used. Additionally, particle embolics can aggregate within very small catheters, leading to occlusion and even catheter rupture. Each microcatheter requires a microwire for navigation, and, similar to the large assortment of microcatheters, there likewise is a large selection of microwires commercially available. Microwire characteristics include size, softness, deformation, steerability, trackability, torque control, and fluoroscopic visibility, which are mainly dependent on the composition of the core wire. Microwires are easily custom-shaped, which is vital for navigating around tight bends and into small distal branches. Biplane fluoroscopy is utilized for navigation cranially, whereas single-plane fluoroscopy is usually sufficient for spinal lesions. To facilitate navigation, the digital roadmap technique is commonly used. With this technique, a mask image of the vascular anatomy is first obtained and then superimposed on real-time fluoroscopic images. The roadmap image is useless if there is patient movement or change in position of the X-ray tube and image intensifier. For that reason, the roadmap technique is not well suited for navigation in the thoracic or lumbar spine because of respirations and bowel peristalsis. Three-dimensional (3D) roadmap techniques have been developed that utilize the reconstructed 3D image of a rotational angiogram as the roadmap.15 The 3D roadmap technique enables the X-ray equipment to be changed to different projections without necessitating acquisition of a new roadmap. Similar to the standard roadmap technique, no patient movement can occur during the acquisition or use of the 3D roadmap. After reaching the desired microcatheter position, a selective angiogram is performed. Ideally, this position is one in which arterial supply is flowing only to the lesion and from which the selected embolic material can reach the target. In addition to confirming microcatheter position, some other goals of the selective angiogram include ruling out flow to normal brain vessels or a spinal cord artery, and the presence of extra- to intracranial anastomoses in the case of head and neck embolizations. The time needed for interpretation of the selective angiogram is dependent on the target lesion. For example, the evaluation of an intracranial arteriovenous malformation (AVM) requires far greater time than that needed for an L5 renal cell carcinoma metastasis. At this point, provocative neurologic testing can be performed using amobarbital and lidocaine injection. Typically, provocative testing is performed on awake patients and aims to elucidate whether there is blood flow to normal brain, spinal cord, or cranial nerves from the established microcatheter position. It has been demonstrated that provocative testing utilizing EEG can predict neurologic complications after AVM embolization.16 An important pitfall of provocative testing is that false negatives can occur as injected drug may be preferentially shunted to high-flow lesions and away from normal neural tissue.10,17 Therefore, some authorities argue that detailed analysis of the selective angiogram is as effective as provocative testing in ruling out dangerous anastomoses or filling of normal branches. There is a broad range of embolic materials currently available for use. During the planning phase of a preoperative embolization procedure, selection of the embolic agent is critical, as it guides the choice of microcatheter and targeted microcatheter position. In selecting an embolic agent, lesion characteristics such as type, location, and rate of flow need to be considered. Embolic agent characteristics that play a factor in the decision include ease of use, cost, and microcatheter compatibility. The discussion in this chapter focus on three categories of embolic agents: particles, liquid embolics, and coils. However, an even wider range of embolic materials has been used in the past and is currently in development.18 Particle embolics are the most commonly used preoperative embolic agents because of the relatively low cost and ease of use. Particle embolics are most effective with lesions that have a capillary bed, such as tumors. One advantage of particles is the ability to deliver the agents from a more proximal microcatheter position because blood flow carries the particles to the tumor capillary bed. The smaller the particle, the more distal it can travel. In the past, particle embolics were used for preoperative embolizations of AVMs, but liquid embolics have largely replaced them because of the increased risk and more frequent hemorrhagic complications associated with particles.19,20 Particles are not radiopaque and therefore must be suspended in dilute contrast to allow for fluoroscopic visualization during injection through the microcatheter.21 The most common particle utilized is the standard polyvinyl alcohol (PVA) particle. PVA particles are irregular in shape and have a tendency to aggregate, which leads to a more proximal occlusion. To minimize the risk of catheter occlusion secondary to particle aggregation, the appropriate-sized microcatheter must be selected and the particle-contrast mixture should not be overly concentrated. Spherical particles such as Bead Block (Terumo Medical Corp., Somerset, NJ), and Embospheres (Merit Medical Systems, Inc., South Jordan, UT) have been designed to have a more consistent shape.22 Similarly sized spherical particles tend to travel more distally than standard PVA particles.23 Smaller particles are more likely to penetrate the tumor capillary bed and produce a more complete devascularization.24 On the other hand, a potential risk of spherical embolic particles or smaller PVA particles (< 150 µm) is passing through fine, distal extra- to intracranial anastomoses during head and neck embolizations, or traveling more distally than intended, which may be associated with an increased risk of postembolization hemorrhage.23,25,26 The authors typically use standard PVA particles (150–250 µm or larger in size) in preoperative embolization cases of extra-axial intracranial tumors such as meningiomas, extracranial head and neck tumors, and spinal lesions. With all particles, there is a high probability of recanalization, as the produced occlusion is partly the result of thrombus that is formed around the particles and eventually breaks down. However, when particles are used as a preoperative embolic agent, recanalization is not an important concern because surgical resection is typically undertaken before significant recanalization can occur. Liquid embolic agents have recently been developed that can be easily injected in the liquid state through the microcatheter but polymerize on reaching the bloodstream. The two main liquid embolics that are currently in use are cyanoacrylates (“glue”) and Onyx. Of the cyanoacrylates, the most commonly used is n-butyl cyanoacrylate (NBCA) (Trufill, Codman & Shurtleff, Inc., Raynham, MA). When exposed to an anionic media such as blood, cyanoacrylates rapidly polymerize. The occlusion produced by NBCA is believed to be permanent,27–29 but there is some evidence that recanalization of embolized vessels can occur.30,31 Meticulous technique must be practiced during preparation and loading of the NBCA to avoid inadvertent polymerization and catheter occlusion. To prevent polymerization in the microcatheter, the catheter is first flushed with 5% dextrose solution. The polymerization time of NBCA can be prolonged by mixing it with ethiodized oil, which is an oil-based contrast agent or glacial acetic acid. Ethiodized oil will also increase the viscosity of the mixture, whereas glacial acetic acid has no effect on the viscosity. Fluoroscopic visualization is further enhanced with the addition of tantalum powder to the mixture. Delivery of the agent can be very tightly controlled, especially if “wedge-flow” is obtained with the microcatheter. In this position the microcatheter tip is occlusive in the distal vessel so that the flow of the delivered agent is directly dependent on each push of the syringe.32 A variety of microcatheters can be used with NBCA, including small, flow-directed catheters such as the Magic microcatheter. Another advantage of NBCA is the ability to push the embolic agent from a more proximal location because the polymerization time of the glue can be adjusted by changing the ratio of NBCA/ethiodized oil to match the characteristics of the lesion. The major disadvantages of NBCA are a relatively short activation time and the risk of catheter retention due to its strong adhesive properties. In the authors’ practice, the assistant removes the microcatheter immediately after the NBCA injection has ceased in a tightly coordinated manner to minimize the risk of catheter retention. NBCA was initially Food and Drug Administration (FDA) approved for preoperative embolization of AVMs, but it also has been utilized for embolization of other intracranial lesions.33,34 The second liquid embolic, Onyx, is composed of ethylene-vinyl copolymer (EVOH), which is dissolved in DMSO. EVOH precipitates when the solution contacts the aqueous bloodstream and the DMSO disperses. Tantalum powder is added to EVOH and DMSO to enable fluoroscopic visualization. EVOH transforms into a nonadhesive spongy material when it precipitates. Onyx is commercially available with varying percentages of EVOH, which affects the rate of precipitation and distal penetration. Several advantages of Onyx over NBCA include greater ease of use, relatively long activation time, and decreased risk of catheter retention, although this complication may still occur if there is significant reflux. Furthermore, Onyx-occluded vessels are softer than NBCA-glued vessels, which facilitates manipulation during surgery,35 and Onyx penetrates smaller, more distal vessels than NBCA.27 It is believed that Onyx produces less of an inflammatory response as compared with NBCA,36,37 but vascular and perivascular inflammation has been detected in Onyx-embolized, AVM surgical specimens.27 Unlike NBCA, the Onyx injection can be stopped and restarted, which allows the Onyx to be redirected to different vascular compartments of the lesion38 and allows for angiograms during pauses in the injection. Furthermore, if “wedge-flow” is not obtained, an Onyx plug can be formed at the tip of the catheter, which prevents reflux of the agent and confers greater control with each push of the syringe.39,40 The main disadvantages of Onyx are the high cost and the limited set of microcatheters that are DMSO-compatible. DMSO can be toxic to the endothelium if injected too rapidly,38,41 and it produces an unpleasant odor as it is metabolized. There has been some debate about the durability of the occlusion produced by Onxy; in a histopathological study by Natarajan et al,27 recanalization was observed in 18% of resected AVM specimens. Similar to NBCA, Onyx was initially FDA approved for the embolization of AVMs, but its use has expanded to other cranial lesions.4,42,43 Microcoils are platinum coils of varying sizes and shapes that can be advanced into a targeted vessel through a microcatheter. In the case of preoperative embolizations, coils are typically utilized as a supplement to other embolic agents by further decreasing flow from an arterial feeder and reducing the rate of recanalization after particle embolization of the tumor capillary bed. Additionally, during extracranial embolizations, microcoils can be deployed to redirect particle embolics to a targeted lesion when the microcatheter cannot be advanced distally or selectively enough to protect normal territory or a dangerous anastomosis. Pushable coils are platinum coils that have interweaving thrombogenic fibers composed of nylon, polyester, or other synthetic substance. The coils require larger-diameter microcatheters and are advanced with a coil pusher. The most common class of coils used in interventional neuroradiology is the detachable platinum coil, which was initially designed and still primarily used for the embolization of intracranial aneurysms.44,45 The prototype coil is the Guglielmi detachable coil (GDC; Boston Scientific Corp., Natick, MA). Detachable coils remain attached to the coil pusher until electrically or mechanically released by the operator and therefore may be repositioned as needed or removed altogether. A significant disadvantage of detachable platinum coils is the high cost. In addition to transarterial embolization, percutaneous embolization techniques have been described as a method to devascularize lesions preoperatively. The most common embolic agents utilized with this technique include the liquid embolics NBCA and Onyx. These techniques have been described for head and neck tumors such as paragangliomas, juvenile nasal angiofibromas, and hemangiopericytomas of the calvarium.1–5,46 Schirmer et al6 described the percutaneous injection of NBCA in a series of five patients with highly vascular, renal cell carcinoma spinal metastases as a complement to transarterial embolization. Percutaneous direct tumor embolization can still carry the risk of ischemic complications, as the embolic material may reflux into branches supplying cranial nerves, branches with extra- to intracranial anastomoses, or reflux directly into the intracranial circulation.47–49 To reduce the risk of emboli traveling into the intracranial circulation, angiography is performed in conjunction with the direct head and neck tumor puncture, which helps guide the degree of embolization, and balloon catheters can be placed in the ICA or vertebral artery as a protective measure. The optimal timing of surgery for tumor resection after preoperative embolization has been debated. One group of neurointerventionists and neurosurgeons has recommended delayed surgery, typically 1 to 2 weeks following embolization. The argument is that the delay allows for embolization-induced tumor necrosis and resulting softening of the tumor, with one study, by Kai et al,50 concluding that maximum tumor resectability was achieved 7 to 9 days after embolization. On the other hand, other authors have recommended early surgical resection following preoperative embolization.51,52 The disadvantage of delayed surgery is the risk of tumor edema and hemorrhage that may occur during the days following embolization.26,52,53 However, at least 24 hours should elapse after embolization for the best hemostatic effect as demonstrated by Chun et al54 in a series of embolized meningiomas. Dexamethasone is usually administered periprocedurally to reduce embolization-induced tumor edema. Another potential disadvantage of delayed surgery is the risk of misgrading tumors. For example, meningiomas may be overgraded because of embolization–induced necrosis and reactive changes.55–57 The authors typically perform preoperative cranial and spinal tumor embolization 24 to 48 hours prior to the scheduled surgery. In the case of AVMs, surgical resection is usually performed 3 to 30 days after the last stage of embolization. One of the most common tumors for which preoperative embolization is performed is meningiomas. These lesions, arising from arachnoid granulation cells, are one of the most common primary central nervous system (CNS) tumors.58 Frequently observed locations include the parasagittal and falcine region, cerebral convexities, sphenoid wing, parasellar region, olfactory groove, and tentorium. Multiple meningiomas are present in about 8% of patients.59 As the tumors arise from the arachnoid, meningeal branches of the external carotid artery (ECA) are usually the initial blood supply, though the tumors may recruit pial vessels. The middle meningeal artery (MMA) most commonly supplies tumors overlying the cerebral convexities, parasagittal region, and sphenoid wing. The supply may be from bilateral MMA when the tumor location is parasagittal or crosses the midline. In the case of olfactory groove meningiomas, feeders usually arise from dural branches of the ICA that include the ethmoidal branches of the ophthalmic artery. Tentorial or clival meningiomas may receive supply from cavernous ICA branches such as the artery of Bernasconi and Cassinari.60 Posteromedial posterior fossa meningiomas usually receive supply from meningeal branches of the vertebral artery, whereas more lateral lesions may receive supply from the occipital artery or the ascending pharyngeal artery (APA). In general, the target of embolization procedures is the ECA tumor feeders because of the increased stroke risk associated with the embolization of ICA feeders. There is variability in the degree of vascularity and therefore the intensity of the tumor blush. A “spokewheel” pattern of intratumoral vessels may be observed. The diagnostic angiogram also provides important information regarding the patency of dural sinuses such as the superior sagittal sinus especially in the case of parasagittal and falcine meningiomas. For large, highly vascular lesions, preoperative embolization may reduce intraoperative blood loss. However, there have been only a handful of comparative studies demonstrating this benefit.61–63 Dean et al62 published a retrospective analysis of 18 embolized patients with meningiomas that were matched with 18 nonembolized patients. The authors found that patients with preoperatively embolized tumors had statistically significantly decreased intraoperative blood loss and transfusion requirements. Another study compared the results at two different neurosurgical centers. One group of neurosurgeons regularly employed preoperative embolization and the other group did not. Intraoperative blood loss was significantly reduced when a greater than 90% devascularization was achieved during embolization.61 The most commonly employed embolic material is particle embolics such as PVA, but liquid embolics such NBCA and Onyx have also been used.33,42,64,65 Importantly, with all ECA embolizations, an understanding of possible extra- to intracranial anastomoses and cranial nerve supply must be attained. An ischemic neurologic complication rate of around 3%66 has been reported and can occur secondary to emboli traveling to unrecognized extra- to intracranial anastomoses or reflux of material into branches with anastomoses or supply to normal neural tissue. Hemorrhagic complications may also occur and are likely secondary to the increased fragility of tumor vessels after tumor necrosis or the result of venous outflow obstruction due to particles traveling too distal.25 Intracranial hemangiopericytomas or meningeal hemangiopericytomas are relatively uncommon tumors, occurring at a frequency of 2.4% of meningiomas.67 The lesions are thought to arise from pericytes, which are the contractile cells surrounding capillaries. The lesions are found most commonly in the supratentorial compartment but do occur in the posterior fossa. The lesions tend to be highly vascularized with prominent vessels often visualized on magnetic resonance imaging (MRI).68 The diagnostic angiogram usually exhibits a prolonged, intense, heterogeneous tumor blush with “corkscrew” vessels.69,70 There is often a mixed dural–pial vascular supply to the tumors.70 Because of the significant vascularity and dual vascular supply, significant intraoperative blood loss is often observed.67,71–73 For that reason, preoperative embolization is advocated. In a small series by Fountas et al,72 the average blood loss from embolized tumors was 508 mL versus 1,160 mL for nonembolized tumors. Targeted tumor feeders include both ICA and ECA branches that can be super-selectively catheterized. The use of embolic materials such as PVA particles, detachable coils, and NBCA have been reported in the literature.33,74,75 Given the rarity of these lesions, no large comparative study has been published definitively demonstrating the hemostatic benefit of preoperative embolization. However, most authors strongly recommend preoperative embolization if possible.72,74,76 Hemangioblastomas comprise a small percentage of all primary CNS neoplasms but comprise 7 to 12% of primary posterior fossa tumors,77 and are the most common primary intra-axial posterior fossa tumor in adults. The classic description of hemangioblastoma is a cystic mass with a mural nodule, though a considerable percentage of the tumors are solid.78 The majority of the lesions are sporadic but about 10 to 20% are associated with von Hippel–Lindau disease. The most common location is the cerebellum, with a much smaller proportion in the medulla and in the intramedullary compartment of the spinal cord.79 In addition to the cyst and mural nodule, serpentine flow voids are often visualized on MRI.78 On diagnostic angiogram, a small highly vascular mural nodule is typically observed with a prolonged vascular stain.80 Because of the significant vascularity, massive intraoperative blood loss is a recognized danger during surgical resection.81 To improve intraoperative hemostasis, preoperative embolization has been recommended by many authors for resection of both cranial and spinal lesions.53,81–84 However, because of the small number of cases, no systematic comparative analysis of the benefits of preoperative embolization has been published. The literature to date has primarily described the use of particle embolics for preoperative embolization.23,53,82,84 Intra-procedure and postprocedure tumor hemorrhage after particle embolization is a significant documented complication.23,85 In addition to tumor hemorrhage, tumor edema is possible,53,82 and ischemic complications may occur from inadvertent occlusion of branches that fill normal cerebellar brain tissue.86 With the development of liquid embolics such as NBCA and Onyx, more effective and safer preoperative embolizations may possibly be undertaken.4,33,87,88 Choroid plexus tumors include choroid plexus papillomas and carcinomas, of which papillomas are twice as common as carcinomas.89–91 The tumors may occur at any age, but 70% of the patients are younger than 2 years old.92 Choroid plexus tumors are rare, but in children younger than 2 years old they may account for up to 12% of intracranial tumors.93,94 In pediatric cases, the tumors are usually located in the lateral ventricle,89 but in adults the lesions are typically found in the fourth ventricle.95 The lesions may have significant vascularity, and surgical mortality attributed to intraoperative blood loss may be as high as 12%.96 In the past, preoperative embolization was hampered by the lack of microcatheters capable of cannulating the small choroidal vessels that feed the tumors.91,97 With the development of small, flow-directed microcatheters such the Magic and Marathon microcatheters, cannulation of these vessels is now possible.34,94 Various embolic materials have been utilized for embolization, including PVA particles, Onyx, and NBCA.34,94,98 At this time, no large comparative study has been published quantitating the benefit of preoperative embolization because of the limited number of cases. Paragangliomas or glomus tumors of the head and neck arise from chemoreceptor cells of the paraganglia or glomus bodies. The most common locations are the carotid body, middle ear, jugular fossa, vagus nerve, and larynx.99 The lesions are slow-growing and locally invasive, leading to bony destruction and infiltration of adjacent structures. The classic description on MRI is a “salt-and-pepper” appearance on T1 or T2 sequences, which represent high velocity flow voids within the tumor.100 Diagnostic angiogram demonstrates a dense vascular blush, with frequent ECA feeders from branches of the APA and occipital artery as well as the MMA. In some instances, supply may also arise from the ICA or vertebral artery. Given the vascularity of the lesions, many authorities advocate preoperative embolization of major ECA feeders prior to surgical resection.101–103 The most commonly used embolic material is PVA particles. Tikkakoski et al104 described their experience with preoperative embolization of neck paragangliomas in 20 patients. In nine preoperatively embolized patients, the intraoperative blood loss averaged 588 mL versus an average of 1374 mL in 11 nonembolized patients. In the case of carotid body tumors, there is some evidence that preoperative embolization does not significantly alter intraoperative blood loss, and therefore some authors do not recommend embolization in these cases.102,105,106 However, analysis of nationwide inpatient data for treated carotid body glomus tumors demonstrated that preoperative embolization was associated with fewer blood product transfusions.107 In addition to transarterial embolization techniques, direct tumor embolization of paragangliomas has been performed with success as described in the previous section. The most commonly used embolic materials include PVA particles and liquid embolics such as NBCA and Onyx.1,3–5,33,46,103,104 Possible complications of embolization include ischemic stroke and cranial nerve palsies secondary to branch occlusion or tumor edema.48,103,108 The occurrence of cranial nerve palsies is likely due to the fact that commonly targeted ECA branches such as the neuromeningeal trunk of the APA provide arterial supply to the lower cranial nerves.102 The risk of permanent cranial nerve palsy is reduced with the use of reabsorbable embolic materials or particles larger than 150 µm. Lastly, extra- to intracranial anastomoses must be ruled out to prevent an inadvertent shower of emboli into the ICA or vertebral artery. Arteriovenous malformations are rare congenital CNS lesions that are part of the spectrum of intracranial vascular malformations. These lesions are high-flow shunts between intracranial arterial feeders and enlarged deep or superficial veins, connected by a nidus of abnormal, malformed vessels. Gliotic, hemosiderin-stained brain parenchyma is found around the AVM nidus. The risk of an initial hemorrhage is 2 to 4% per year.109–114 The role of embolization in the treatment of AVMs has evolved over time for these complex lesions. As a stand-alone curative procedure, endovascular embolization at this time is only appropriate for a small subset of AVMs.115 Currently, endovascular embolization is combined with microsurgical resection or radiosurgery in a multimodality treatment approach.116–119 The primary target of AVM embolization is the nidus, though features such as flow-related and intranidal aneurysms warrant special attention during the preoperative embolization.120 For AVMs, preoperative embolization may reduce intraoperative blood loss by occluding deep, surgically difficult-to-reach arterial feeders, reducing nidus size, and reducing flow and degree of arteriovenous (AV) shunting. Other advantages of preoperative embolization include reduced operative time and decreased risk of perioperative rupture of intranidal aneurysms or flow-related aneurysms.121 The diagnostic stage and selective microcatheter stage of the preoperative AVM embolization require careful examination. The angiographic analysis of the arterial feeders includes defining true terminal feeders from en passage vessels or pseudoterminal vessels, arteriopathy such as stenoses or ectasias, and flow-related aneurysms. Analysis of the AVM nidus includes defining the size, shape, location, rate of flow, ectasias, and intranidal aneurysms. Lastly, angiographic analysis of the venous drainage includes understanding the deep or superficial pattern, the number of draining veins, the specific veins and territories, the dural sinuses involved, stenoses, occlusions, and varices.122 The PVA particles have been largely replaced by the liquid embolics NBCA and Onyx, which were given FDA approval for AVM embolization in 2000 and 2005, respectively. After PVA embolization, there was a higher rate of postresection hemorrhage compared with patients who underwent preoperative embolization with NBCA.19 Despite the above-stated theoretical advantages of preoperative AVM embolization, there are few comparative studies definitively demonstrating decreased intraoperative blood loss after preoperative AVM embolization. Pasqualin et al123 found that there was reduced intraoperative bleeding in patients with preoperatively embolized AVMs when the lesion was greater than 20 mL in volume. The embolic agents utilized in the study were Silastic sponge and polyfilament polyethylene thread since the study was performed prior to the routine use of liquid embolics. Jafar et al124 demonstrated in a group of 33 patients who underwent surgery alone or combined with NBCA, intraoperative blood loss was similar despite the fact that the AVMs in the embolized group were significantly larger and were of a higher Spetzler-Martin grade. Intraoperative blood loss has not been shown to be significantly different between NBCA-embolized AVMs versus PVA-embolized AVMs,19 or between NBCA-embolized AVMs versus Onyx-embolized AVMs.37 Multistage embolizations can be performed prior to surgical resection. The authors typically wait 2 to 4 weeks between each stage of embolization. Complications associated with AVM embolizations are not insignificant and include periprocedural hemorrhage, ischemic events, and death. Several recent series have reported mortality rates between 1% and 2.6% and permanent disabling complication rates between 1.6% and 11%.125–129 Complications related to the embolization procedure may be limited by careful evaluation of the superselective angiogram, use of less traumatic flow-directed catheters, use of liquid embolics, tight periprocedural blood pressure management, and staged embolizations that may reduce the incidence of periprocedural hemorrhage secondary to normal perfusion pressure breakthrough.130 Hypervascular metastatic disease of the spine most often involves renal cell, thyroid, breast, prostate, lung, and hepatocellular carcinomas as well as melanoma. Metastatic tumors present with metastases to the spine in 30 to 70% of cases.131 Of the above-mentioned tumors, renal cell carcinoma (RCC) and thyroid carcinoma are the most commonly associated with highly vascularized vertebral body lesions,132 and the majority of the literature demonstrating the benefits of preoperative embolization has focused on RCC.133–138 As a result of the significant vascularity, surgical resection of these metastatic spinal lesions is hampered by the extensive intraoperative blood loss, which results in increased morbidity and mortality.139–141 Preoperative embolization has been strongly advocated as a critical component of the treatment paradigm for hypervascular metastatic spine disease.132,134,136,137,141,142 A study by Berkefeld et al,139 which predominantly involved patients with RCC, reported average intraoperative blood loss in nonembolized patients of 4,350 mL versus 1,800 mL in patients who underwent PVA particle embolization. The investigators also combined coils with PVA particles in select cases, but the coils did not appear to confer additional hemostatic benefit. In another small study of 17 patients with preoperatively embolized RCC spinal metastases and 10 matched controls, the hemostatic benefit of PVA particle embolization was also demonstrated.135 In fact, for two of the control patients, the resection was aborted because of life-threatening hemorrhage. In a recent report by Wilson et al138 of 100 spinal preoperative embolizations for both primary and metastatic tumors, RCC accounted for 38% of the cases. The authors found that RCC was associated with significantly increased intraoperative blood loss as compared with the other pathologies. A benefit from preoperative embolization was confirmed in that the average Intraoperative blood loss for partially or nonembolized RCC tumors was 3,460 mL versus 1,821 mL for completely embolized RCC tumors.138 Additionally, adjunctive percutaneous embolization techniques have been described for the embolization of RCC spinal metastases as mentioned previously. Camille et al143 were the first to report on the use of preoperative embolization of spine and pelvic thyroid carcinoma metastases. However, no large series or comparative study has been published to date demonstrating the efficacy of preoperative embolization for thyroid carcinoma metastases. A major possible neurologic complication related to the embolization procedure is spinal cord ischemia secondary to occlusion of a radiculomedullary branch that fills a spinal cord artery. This can be avoided through careful examination of the segmental artery angiogram.139 In the case of cervical spine tumors, the interventionist must be vigilant for possible anastomoses between the carotid, vertebral, and costocervical, and thyrocervical trunks of the subclavian artery, which can result in cerebral emboli and infarction.138,144,145 Preoperative embolization has been utilized in the treatment of primary bone tumors such as hemangiomas, osteoblastomas, aneurysmal bone cysts, and giant cell tumors. Hemangiomas are common benign lesions of the vertebral body that can produce spinal cord compression from mass effect or pathological compression fracture.146 Preoperative embolization has been recommended by various authors because of the potential for significant intraoperative blood loss.147–149 Symptomatic spinal hemangiomas may benefit from transarterial preoperative embolization with particle embolics or Onyx prior to undergoing surgical resection.150,151 Osteoblastomas account for about 3% of benign and 1% of all primary bone tumors.152 Surgical resection is the treatment of choice153; however, complete surgical excision is usually impeded by excessive intraoperative bleeding. In a small series by Trübenbach et al,154 preoperative embolization with particle embolics was accomplished in three cases of cervical spine osteoblastoma with no reported complications. The authors did not specify the exact degree of blood loss in these cases. Aneurysmal bone cysts are expansile bone lesions that are characterized by a reactive proliferation of connective tissue and multiple blood filled cavities.155 Preoperative embolization with PVA particles is utilized for these highly vascular lesions in an attempt to prevent excessive intraoperative bleeding.147,155,156 However, aneurysmal bone cysts usually lack a true major feeding vessel and are supplied instead by a network of small vessels, which makes preoperative embolization unfeasible in some cases.147 As is the case for embolizations of metastatic spine lesions, avoidance of neurologic complications involves careful examination of the preintervention diagnostic angiogram to identify the presence of any radiculomedullary branches that fill spinal cord arteries and the presence of any anastomoses to the carotid or vertebral arteries in the case of cervical spine lesions. There is a variety of hypervascular benign and malignant lesions in the craniospinal axis for which surgical resection may be significantly hampered by excessive intraoperative blood loss. The extensive blood loss that may occur in these cases can lead to significant morbidity and mortality. The development of new technologies and materials such as digital 3D roadmapping, flow-directed microcatheters, and liquid embolic agents has allowed neurointerventionists to address more and more of these hypervascular lesions prior to attempted surgical resection. Embolization procedures do carry risks, and those risks must be carefully weighed against the morbidity and mortality associated with surgical resection without preoperative embolization. However, all practicing neurosurgeons should be aware of this growing armamentarium now available for attaining preoperative preemptive surgical hemostasis of hypervascular lesions. • Careful attention should be paid to the preoperative imaging to determine if the lesion to be removed would be appropriate for endovascular treatment to reduce blood loss at surgery. • The use of microcatheters for selective embolization facilitates safety with preoperative embolization. • Agents for tumor and vascular malformation embolization include particles, liquid embolics, and coils. • Onyx, a liquid embolic agent, has been a revolutionary improvement in the endovascular armamentarium. • For AVMs, liquid embolic agents have superseded the use of particles for embolization. • Timing of surgery should be carefully planned when using particles to avoid recanalization of the vascular supply.
Preoperative Non-Hematologic Adjuvant Methods for Preventing Blood Loss
Embolization Techniques
Catheters, Wires, and Technique
Arterial Access
Catheters (Overview)
Microcatheters and Wires
Angiography Technique
Embolic Agents
Particle Embolics
Liquid Embolic Agents
Microcoils
Direct Tumor Puncture Embolization
Timing of Surgery
Common Preoperatively Embolized Lesions
Cranial, Head, and Neck Lesions
Meningiomas
Hemangiopericytomas
Hemangioblastomas
Choroid Plexus Tumors
Paraganagliomas
Arteriovenous Malformations
Spinal Lesions
Metastatic Spine Disease
Primary Spine Bony Lesions
Conclusion
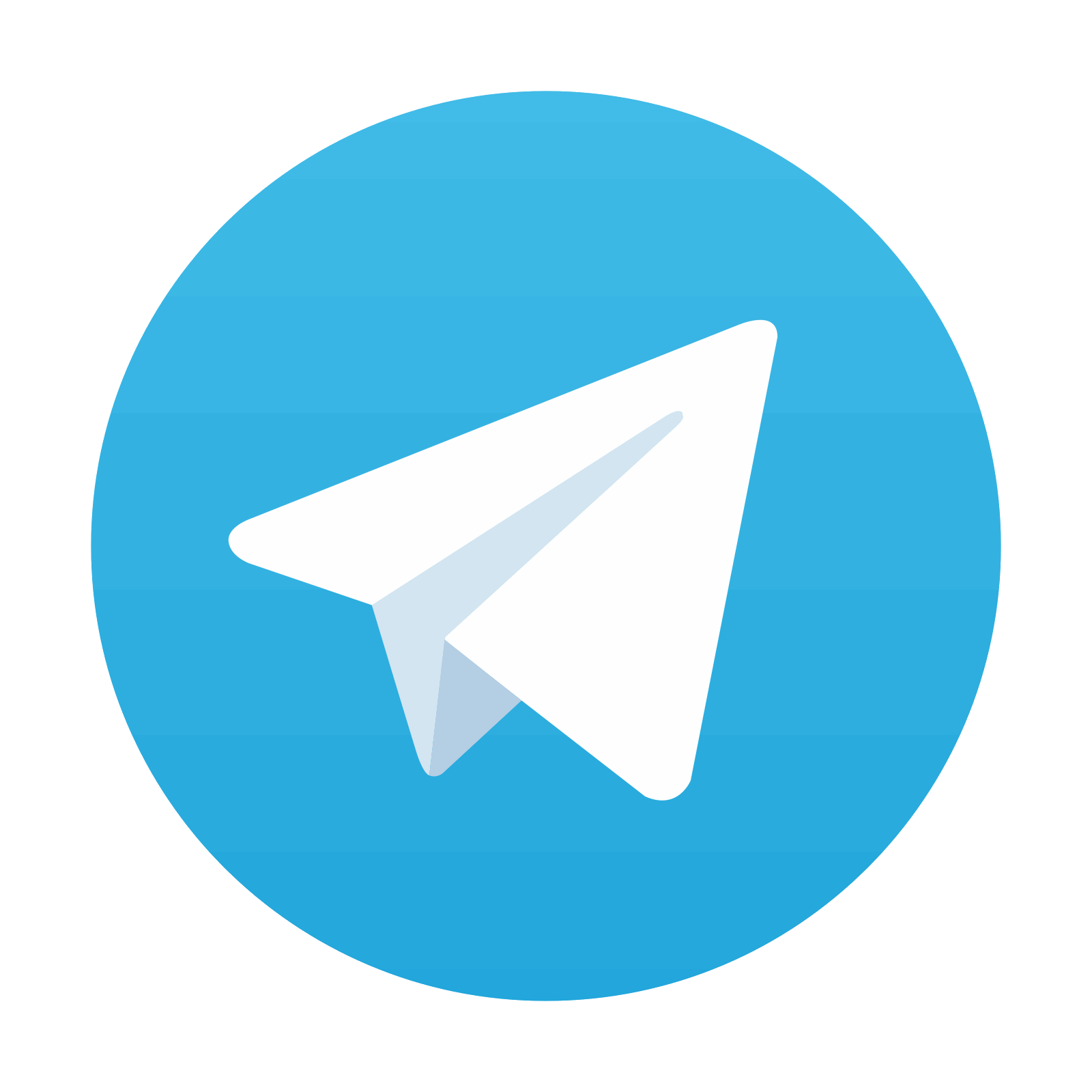