8 A thorough understanding of indications for blood replacement is essential for all neurosurgeons. The breadth of neurosurgical procedures, the often critically ill status of the neurosurgical patient population, and the unique perfusion requirements of the brain and spinal cord necessitate special consideration. This chapter discusses the assessment, rationale, indications, complications, and alternatives for blood replacement in neurosurgical patients. Preoperative laboratory data can be helpful in assessing the need for or anticipating the likelihood of blood replacement. A preoperative analysis of hemoglobin and hematocrit levels can indicate the presence of anemia and establish a baseline for future transfusions. A patient with chronic anemia may better tolerate a lower hemoglobin level than a patient experiencing acute blood loss. The finding of unexpected anemia should prompt a determination of the underlying cause before surgery. The platelet count, prothrombin time (PT), and partial thromboplastin time (PTT) provide an evaluation of the substrates for blood clotting. Abnormalities should be investigated and corrected when possible. Finally, deficiencies in any of the above factors or anticipation of significant blood loss can help the surgeon prepare by securing blood products for transfusion (especially the option of autologous donation), requesting blood recovery devices, or modifying the surgical strategy. Given the potentially devastating consequences of bleeding complications in neurosurgical patients, a thorough preoperative assessment of bleeding risk is critical. The most important aspect of the assessment is a detailed bleeding history. The patient should be questioned regarding a bleeding tendency, easy bruising, bleeding problems associated with previous surgeries, and a family history of bleeding pathologies. A patient who is a reliable historian and has no history of bleeding problems is at very low risk for bleeding complications from intrinsic hypocoagulability. Laboratory assessment includes evaluation of the clotting cascade and the effectiveness of platelet function as detailed below. In a neurosurgical context they are useful before, during, and after surgery. The PT evaluates the effectiveness of the extrinsic pathway of coagulation, specifically the tissue factor (TF) and final common pathways. The PT is used to monitor the clotting potential of blood with warfarin therapy, vitamin K deficiency, and the adequacy of liver synthesis of clotting factors. To perform the test, a suspension of tissue thromboplastin (TF + phospholipids) and calcium chloride is added to platelet-poor plasma. The time to the formation of a fibrin clot is the PT. Because different sensitivities of tissue thromboplastin reagents produce variability in PT, the international normalized ratio (INR) was introduced. It is calculated using the following formula: (Patient PT/Mean normal PT)ISI, where ISI is the International Sensitivity Index, a value assigned to the PT reagent when compared with a World Health Organization reference standard. The INR was standardized using plasma from patients on chronic warfarin therapy, which affects only vitamin K–dependent factors (VKDFs) II, VII, IX, and X. The INR will not necessarily be abnormal if other clotting factors are affected. The PTT (or activated PTT) test evaluates the intrinsic pathway, including contact factors (factor XII, high molecular weight kininogen, and prekallikrein) and factors VIII, IX, and XI. Specifically, it evaluates the coagulation of plasma induced by the activation of factor XII with a surface-activating agent. Silica or kaolin is used in the presence of a phospholipid extract of brain lacking tissue factor, which is therefore a partial thromboplastin. PTT is useful for monitoring the effects of heparin therapy and the direct thrombin inhibitors. The laboratory evaluation of blood components should include a platelet count. The normal range is usually defined as 150 to 400 × 109/L; however, a normal platelet count does not guarantee normal platelet function. Some authorities think that minor brain surgery can be performed safely with platelet counts between 60 and 100 × 109/L. Counts between 20 and 60 × 109/L may be associated with excessive bleeding that does not cease with the usual maneuvers or in a timely fashion during surgery. Spontaneous bleeding seldom occurs until platelet counts are less than 20 × 109/L. The cause of thrombocytopenia is important when considering its probable impact on bleeding. The differential diagnosis includes massive transfusion, hemodialysis, platelet sequestration (e.g., from hypersplenism), decreased production from congenital or acquired anemias (e.g., from aplastic anemia, Wiskott-Aldrich syndrome, ionizing radiation, myelosuppressive drug use, nutritional deficiencies), immune destruction (e.g., from idiopathic thrombocytopenic purpura) or nonimmune destruction (e.g., von Willebrand disease, sepsis, thrombotic thrombocytopenic purpura, burns), or heparin exposure. When the result is unexpected, the test should be repeated to rule out pseudothrombocytopenia or artifactual thrombocytopenia, which occurs when platelets clump in vitro in the presence of the anticoagulant calcium-chelator ethylenediaminetetraacetic acid (usually a purple-top Vacutainer tube). If this clumping is abolished with the use of a citrate-containing solution (usually a blue-top Vacutainer tube), the diagnosis is confirmed.1 Bleeding time (BT) is typically measured using the Ivy method, in which a sphygmomanometer on the upper arm is used to raise tissue pressure to 40 mm Hg. A standardized 1-mm-deep incision is made in the skin of the anterior forearm. The time it takes for the bleeding to stop is the BT. A normal value is 2 to 9 minutes. The test is still used clinically, but its reliability is considered to be low because its reproducibility is poor. Accordingly, more standardized automated in vitro methods such as the platelet function analyzer have been introduced. In this test, a machine simulates ex vivo bleeding time by drawing whole blood collected in citrate anticoagulant into two collagen-coated cartridges, which stimulate platelets with collagen and epinephrine (CEPI) or adenosine phosphate. The blood interacts with von Willebrand factor to drive clotting that closes an aperture. If the closure time for epinephrine is less than 180 seconds, platelet function is considered to be normal. If aspirin is present, a normal result implies aspirin resistance. If CEPI is longer than 180 seconds and the closure time for collagen and adenosine diphosphate (CADP) is less than 116 seconds, the most likely cause is the use of aspirin or nonsteroidal anti-inflammatory drugs. If the CEPI is longer than 180 seconds and the CADP is longer than 116 seconds, platelet function is abnormal. A markedly elevated result, such as longer than 300 seconds for both tests, suggests the use of a glycoprotein (GP) IIb/IIIa inhibitor. All of these tests can provide a preoperative assessment of the adequacy of the conditions for blood clotting. As blood loss occurs in surgery, the tests can be repeated to detect which clotting components are being depleted to the point where they are no longer effective. The membranes of human red blood cells (RBCs) are estimated to contain more than 300 different antigens, and at least 20 different blood group antigen systems have been identified. In most transfusions, only the ABO and Rh antigens play an important role. Patients are at risk for acquiring antibodies to alleles they do not possess (nonself), leading to potentially serious adverse reactions. Almost all individuals produce antibodies to nonself AB alleles by 1 year of age, regardless of their prior exposure. For the Rh antigens, the D antigen is of primary importance. Patients are classified as positive or negative based on the presence of this antigen; 80 to 85% of the population is positive. Rh-negative patients typically develop antibodies only after a transfusion or pregnancy. Transfusion of Rh-positive blood to a man or postmenopausal women is seldom of consequence. Such transfusion is an option in an emergency situation but should be avoided if possible. Compatibility testing is performed to help avoid a reaction to a transfusion. Testing is performed in the form of screening and crossmatching. The patient’s ABO and Rh type are determined by testing the patient’s blood against serum known to contain antibodies to A, B, and Rh. The results are confirmed by testing the patient’s serum against RBCs with known antigens. Typing can be performed in about 15 minutes. An antibody screen is designed to detect the most common non-ABO reactions by means of an indirect Coombs test. The patient’s serum is mixed with RBCs with known antigens. If the patient has antibodies to these antigens, the antibodies will coat the RBCs and the addition of antiglobulin antibody will cause agglutination of the RBCs. Screens require 45 minutes to perform. They are routinely done on all donor blood and can potentially replace a crossmatch for a recipient. A crossmatch mimics a transfusion in that donor cells are mixed with recipient serum. A crossmatch is able to confirm typing, detect other antibodies, and detect antibodies in low titers not evident on the screen (because they did not agglutinate). Crossmatching provides optimal safety but is reserved for procedures in which a transfusion is anticipated. If the procedure is associated with a transfusion rate of less than 10%, only a type and screen should be performed. A crossmatch- to-transfusion ratio of 2.5:1 is the goal. Ample time and blood should be allocated to allow crossmatching in patients who have undergone prior transfusions, in situations in which multiple transfusions are anticipated, or in patients with known antibodies.2,3 Transfusions are often necessary and can be lifesaving. However, transfusions should be performed judiciously. Adverse events are common, serious, and potentially life-threatening, and the inappropriate use of transfusions is associated with increased rates of morbidity and mortality. Therefore, surgeons should be familiar with the indications and potential consequences, and the potential for adverse reactions should be anticipated. Primary complications are immunity and infection related and include, but are not limited to, acute and delayed hemolytic reactions, transmission of infectious diseases, and development of immune-compromise and coagulopathy. Acute hemolytic reactions are caused by ABO incompatibility and occur in 1 in 38,000 transfusions. They are typically due to misidentification and result in acute intravascular hemolysis. Patients present with fever, chills, nausea, tachycardia, hypotension, hemoglobinuria, and diffuse surgical oozing. Hemolytic reactions can lead to disseminated intravascular coagulation, shock, and renal failure and are fatal in 1 in 100,000 transfusions. The severity of the reaction often depends on the amount of blood transfused. Barring an emergency, blood products should always be started and infused slowly. Delayed hemolytic reactions occur 2 to 21 days after transfusion, when antibodies develop against less common antigens found in blood products. These reactions are mild and can result in extravascular hemolysis. Nonhemolytic immune complications include fever, urticarial reactions, anaphylaxis, noncardiogenic pulmonary edema, and graft versus host disease. Anaphylaxis occurs in 1 in 150,000 transfusions, most commonly in immunoglobulin A (IgA)-deficient patients. Transmission of infectious disease is the other major potential complication of blood transfusion. The advent of routine testing for hepatitis and human immunodeficiency virus (HIV) has markedly reduced the transmission rate of these viruses through blood products. The incidence of transfusion-acquired hepatitis C is reported to range from 1 in 60,000 to 1 in 1,900,000 and that for HIV to be 1 in 1,900,000. Cytomegalovirus (CMV) and Epstein-Barr viruses can also be transmitted through this route. Because CMV is capable of mounting a severe infection in immunocompromised patients, these patients should be transfused with CMV-negative units or leukocyte-reduced units. Bacterial contamination is another potential complication of transfusion and is the second leading cause of transfusion-related deaths. Bacterial contamination is present in 1 in 2,000 platelets and in 1 in 7,000 packed RBCs. Sepsis occurs in 1 in 25,000 platelets and 1 in 250,000 packed RBCs. Prevention measures should include appropriate storage of blood and transfusion within 4 hours of removing the units from storage to ensure the correct temperature. If slower transfusion is desired, the unit should be split. Blood transfusions are also believed to cause immunosuppression by an unknown mechanism and therefore may increase the risk of serious infection. Massive transfusions are associated with a coagulopathic state, typically from platelet dilution. The patients at highest risk of transfusion-related complications are those who have undergone prior transfusions, the immunocompromised, and those receiving multiple transfusions. A patient who has received previous transfusions is at higher risk for hemolytic, febrile, and urticarial reactions. More time and blood should be allocated to pretesting to ensure appropriate crossmatching. Febrile reactions can be reduced by using leukocyte-reduced packed RBCs. Leukocyte-reduced cells can help lower the risk of infection, and irradiated cells can help prevent graft versus host disease, particularly in immunocompromised patients who are at higher risk. Massive blood transfusion is also associated with unique risks, including coagulopathy, hypocalcemia, and hypothermia. In patients receiving one or two times their circulating blood volume, preparation should be made to transfuse platelets and fresh frozen plasma (FFP), and all blood products and fluids should be warm.2,3 Maintaining proper fluid balance is critical during anesthesia. Preoperative fluid deficit, maintenance of fluid requirement, and replacement of ongoing fluid losses should be considered. Maintenance of fluid requirement is typically estimated as 100 mL/kg/day for the first 10 kg of body weight, 50 mL/kg/day for the next 10 kg, and an additional 20 mL/kg/day for each kg over 20 kg. Surgical patients enter surgery with a fluid deficit from their preoperative fast, and this deficit must be replaced. For the average 70-kg adult, fasting for 8 hours amounts to an 880 mL loss in fluid. Once surgery has begun, fluid loss is accelerated due to surgical blood loss, wound exposure, and tissue trauma. Most neurosurgical procedures are associated with only a small amount of evaporative fluid loss; however, evaporative loss can become significant during long spinal procedures involving large wounds and extensive tissue trauma. Fluid status and blood loss must be assessed and corrected constantly. Vital signs, urine output, estimated blood loss, and laboratory data must be monitored closely. Unfortunately, the volume of blood loss can be difficult to estimate given that considerable blood can be lost under surgical drapes and in sponges and due to calculation errors incurred by the contribution of irrigation fluid. Volume replacement should precede the development of hypotension and tachycardia, two physiological signs of the body’s response to volume depletion. A drop in urine output is a useful sign of hypovolemia. An adult should maintain a urine output of 0.5 to 1.0 mL/kg/hour. The base deficit can also indicate the volume status and adequacy of replacement. Serial evaluations of the hematocrit can be followed to assess blood loss. However, because the hematocrit level reflects the ratio of blood cells to plasma rather than blood loss, results can be affected by fluid shift and intravenous replacement. Therefore, clinical status and the estimated blood loss should take precedence. The timing and indications for colloid solutions (e.g., serum albumin) are not well established. Administration of colloid solutions is usually considered after 3 to 4 L of crystalloid solution have been administered with an inadequate hemodynamic response. Colloid solutions are typically administered in a 3–4:1 ratio to blood loss. Colloid solutions correct fluid deficits more rapidly than crystalloid solutions, have an intravascular half-life of 3 to 6 hours, and are less frequently associated with significant tissue edema. The effective osmoles in colloidal fluids are larger and therefore are less likely to leach into the interstitial space, which is believed to be the reason for the reduction in edema. Tissue edema can impair oxygen delivery and tissue healing. Because prevention of tissue edema and poor oxygenation is critical in neurosurgery, particularly in the case of head trauma, some neurosurgeons favor colloid use for fluid replacement. Despite its desirable features, colloid use is limited by its cost and potential complications. Colloids are derived from either plasma proteins or synthetic glucose polymers in an isotonic solution. Blood-derived colloids include albumin and plasma protein fraction and carry a risk of viral transmission and a hypotensive allergic reaction. Synthetic colloids include gelatins (not available in the United States) and dextrose starches. Dextrans are associated with antiplatelet effects, renal failure, and mild-to-severe anaphylactic reactions. Hetastarch is a highly effective plasma expander that is rarely associated with anaphylactic reactions or negative effects on coagulation status.3,4 Whole blood is seldom administered for replacement, except in massive transfusion protocols. Instead, individual blood components are administered depending on the perceived deficit the patient is suffering. This section reviews general protocols and indications for blood product transfusion and then presents concerns specific to cranial and spinal surgery. The RBCs are used to increase oxygen-carrying capacity during blood loss and profound anemia in the setting of impaired oxygen delivery. One unit of packed RBCs (pRBCs) has a hematocrit of 55 to 80% and a volume of 250 mL. In a 70-kg male, a single unit can be expected to raise hemoglobin by 1 g/dL and the hematocrit by 3%. This amount is usually physiologically inconsequential; therefore, at least two units of pRBCS are typically administered at a time.5 Given the considerable risks of both over- and undertransfusion, considerable effort has been exerted to determine the appropriate indications for transfusion. This effort led to the development of a Task Force on Blood Component Therapy by the American Society of Anesthesiologists. The Task Force concluded that transfusion should not be dictated by a single hemoglobin level; rather, it should be based on the individual patient’s risk of developing complications related to inadequate oxygenation. In most instances, the Task Force found that transfusion was indicated for a hemoglobin level < 6 g/dL and was rarely needed for hemoglobin levels > 10 g/dL. Carson et al6 published a meta-analysis of six studies comparing liberal and restrictive transfusion trials. They analyzed complete transfusion outcome and 30-day mortality rates in 1,568 patients. The liberal transfusion group had a mean transfusion trigger of 9.7 mg/dL of hemoglobin and received 4.4 units of RBCs. The conservative group had a mean transfusion trigger of 7.5 mg/dL of hemoglobin and received an average of 2.3 units of RBCs. There were 120 (15.2%) deaths in the liberal group compared with 94 (12%) in the conservative group, suggesting that the incidence of adverse events is lower with restrictive transfusions than with liberal transfusion. The incidence of cardiac events was not affected.7 The type of neurosurgical pathology and type of patient may affect the decision to transfuse. In patients with subarachnoid hemorrhage, a target hematocrit of 30 mg/dL is often accepted as a good balance between dilute enough to promote flow through optimum rheology and concentrated enough to deliver adequate oxygen to the brain. In contrast, the hematocrit level of a spine surgery patient with a history of coronary ischemia and whose hematocrit level is borderline immediately after surgery may need to be kept higher to prevent a drift into a clinically significant anemia. Platelets are transfused prophylactically to prevent bleeding or for therapeutic replacement in thrombocytopenic patients with active bleeding. Platelets come as pheresis-collected single donor units containing 3 to 6 × 1011 platelets each. They can also be collected from the whole blood of six different donors containing an average of 7.5 × 1010 platelets in each pack. Single donor platelets are preferred, because they are associated with a lower rate of disease transmission and less potential to drive an allergic response. Transfusion of one single donor unit of platelets is expected to raise the platelet count by 50,000/mm3. This response, however, is variable. Laboratory values should be followed to assess the actual amount of platelet correction. Increased platelet consumption, active thrombosis, destruction by platelet antibodies, and splenic sequestration can blunt the expected platelet response. Importantly, the cause of thrombocytopenia should be considered when deciding to transfuse. In platelet-consumptive conditions, the available platelets are typically younger, larger, and better functioning, thereby reducing the need for transfusion. Patients with platelet hypoplasia and comorbid conditions are more likely to require a transfusion. The Task Force recommended prophylactic platelet transfusion for surgical patients or patients with microvascular bleeding with platelets < 50,000/mm3, and for high-risk surgical patients with or without microvascular bleeding, with platelets between 50,000 and 100,000/mm3. The Task Force does not recommend routine prophylactic transfusion for thrombocytopenia related to platelet destruction, for minor procedures or surgeries associated with insignificant blood loss, or for surgical patients with platelet counts > 100,000/mm3. They also noted that platelet transfusion may be necessary for patients with normal platelet counts but known platelet dysfunction and microvascular bleeding.8 One unit of FFP contains 200 to 250 mL of volume, 1 unit of each coagulation factor, and 2 mg of fibrinogen per milliliter. A unit of FFP has an INR of 0.9 to 1.2 and correction with FFP should not be expected to lower a patient’s INR below 1.2 to 1.3. FFP takes 20 to 40 minutes to thaw, and this process cannot be accelerated. The Task Force recommends transfusion of FFP for urgent reversal of warfarin, for correction of known factor deficiencies when specific factors are unavailable, for correction of microvascular bleeding with PT or PTT over 1.5 times normal, and for patients with microvascular bleeding who have received a massive transfusion (≥ 12 units PRBC).8 Given the brain’s sensitivity to oxygen deprivation and the potentially devastating consequences of uncontrolled bleeding in neurosurgical patients, blood replacement in cranial procedures requires special consideration. Weiskopf et al9 found that cognitive functioning is compromised at hemoglobin values less than 6 mg/dL. This study was performed in healthy volunteers; many believe that the injured brain is susceptible to anemia at even higher hemoglobin levels. Animal and human studies have shown worse outcomes in anemic patients who suffer a traumatic brain injury.10 However, transfusion has not been shown to improve their outcomes.11 Unfortunately, no adequate studies are available to guide transfusion triggers in the neurosurgical population. However, it is reasonable to believe that transfusion strategies applicable to other patients may not be most appropriate for the neurosurgical population. Further research is needed to determine the optimum hemoglobin target for a transfusion of RBCs in patients with central nervous system (CNS) pathology. Platelet and factor replacement is also unique in neurosurgical patients, given the high risk of bleeding complications and coagulopathic state that often accompanies CNS disease. Patients undergoing neurosurgery or those suffering from intracranial hemorrhage usually receive transfusions when their platelet count is less than 100,000/mm3. Platelets in patients with a brain injury may not function normally. Ongoing microvascular bleeding despite normal platelet counts should prompt consideration of transfusion. Spinal surgery can often be extensive and result in considerable blood loss. Anticipation of these factors provides the opportunity to use transfusion-conservation strategies, such as cell saver and normovolemic hemodilution. Close attention should be paid to blood loss during surgery, pursuing meticulous hemostasis wherever possible. As larger cases progress, regular review of the status of blood loss with the anesthesia team, as well as communicating future anticipated blood loss, can facilitate proactive strategic replacement. Finally, for cases of extreme blood loss involving an entire volume of blood or more, the option of staged surgery is a reasonable consideration. Intraoperative cell saver, or autologous blood salvage, is often used in surgeries when a large volume of blood loss and the need for blood transfusion are anticipated. The most appropriate use in neurosurgery is in clean non-oncological cases where considerable blood loss is common (e.g., large spine surgeries), rather than in cases where large volume blood loss is possible but infrequent (i.e., aneurysm repair). Cell saver circumvents the risk of adverse effects from an allogenic blood transfusion and may be accepted by patients who refuse exogenous transfusions due to religious beliefs. Three types of cell savers are available: RBC savers, direct transfusion, and ultrafiltration of whole blood. Cell processors or RBC savers centrifuge intraoperatively salvaged blood. RBCs are washed and separated, whereas platelets, plasma proteins, clotting factors, and by-products such as cytokines, anaphylatoxins, and additional waste products are removed. In a direct transfusion, blood is transferred through an extracorporeal circuit, typically during cardiopulmonary bypass surgeries, collected, and then re-infused. This blood tends to be more dilute (hemoglobin 6–9 g/dL) and contains cytokines and other waste products that have been implicated in organ dysfunction and edema. The third type, ultrafiltration of whole blood, removes excess noncellular plasma water, solutes, particulate matter, and waste substances, and re-infuses whole blood. It includes platelets, clotting factors, and plasma proteins and typically retains a normal level of hemoglobin. During blood recovery, many commonly used hemostatic agents should be avoided to prevent clotting within the recovery circuit. Studies are needed to compare the efficacy of various cell salvage techniques in replacing intraoperative blood loss in neurosurgical patients.12–14 Normovolemic hemodilution is based on the principle that reducing the concentration of RBCs leads to a reduction in the total number of RBCs lost during large volume blood loss and that cardiac output remains normal when intravascular volume is maintained. The process begins with the controlled removal of one to three units of whole blood, depending on the level of the preoperative hematocrit, to target a hematocrit of 28%. The blood volume removed is replaced with three to four times the volume of crystalloid or colloid solution. The blood is then transfused when needed. A study involving adolescents undergoing correction of scoliosis showed a reduction of allogeneic transfusion from 79% to 37% using normovolemic hemodilution. Another study in patients undergoing lumbar fusion showed an allogeneic transfusion rate of 23.5%, whereas a group in a comparable study had a rate of 40%. Given the aforementioned concerns with allogeneic transfusion, such a drastic reduction could have a profound impact on patient outcomes.15 Again, this kind of conservation strategy makes the most sense in procedures such as spine surgery to correct a deformity, where significant blood loss is almost certain. Some patients will not accept blood product transfusion because of their religious beliefs. In North America, the most common group encountered is the Jehovah’s Witnesses, who refuse blood transfusions on the premise that several biblical passages state that blood cannot be eaten as “nourishment.” As a group, Jehovah’s Witnesses typically will not accept whole blood, RBCs, platelets, plasma, or stored autologous blood. Acceptance of the following products varies on an individual basis: plasma proteins (albumin, cryoprecipitate, immunoglobulin), stem cells, autologous blood preserved in a cell saver, clotting factors, bone marrow transplants, organ transplants, epidural blood patches, and extracorporeal circulation (dialysis, plasmapheresis, cardiac bypass machines). Correcting anticoagulation or controlling acute bleeding is a challenge in these patients, and physicians must be aware of and use strategies to minimize perioperative blood loss, to optimize hemodynamics, and to increase blood production. The strategies used can be applied to all neurosurgical patients. Perioperative blood loss can be limited by conducting phlebotomy only when necessary, by using pediatric sample tubes (can decrease blood volume drawn 40–45% compared with conventional tubes), by increasing point-of-care testing, and by using the appropriate medication to prevent bleeding (e.g., desmopressin and aprotinin). The neurosurgeon should strongly consider optimizing RBC mass and production before surgery via nutritional supplementation with vitamin B12, folate, and iron (intravenous route acts much more quickly), and a nutritional consultation should be considered.16,17 Erythropoietic stimulating agents such as erythropoietin may help, depending on the acuity of blood loss. Some studies have shown a decrease in transfusion requirements when erythropoietic stimulating agents are administered in the acute setting.18,19 However, these agents are not a replacement for transfusion, because there is still a delay of several weeks between the administration of erythropoietin and the production of new RBCs.20 Careful preoperative planning involves possible angiographic embolization, staged operations, and selection of minimally invasive procedures. Plans for careful dissections and vigilant coagulation of all bleeding, regardless of how minor, are crucial for these patients.21 Hemodynamic parameters may be maximized by increasing oxygen content, by optimizing cardiac output, and by lowering the patients’ metabolic rate. Blood substitutes such as hemoglobin-based oxygen carriers have been studied as an alternative to blood transfusion. No blood substitute has been approved by the US Food and Drug Administration in the setting of acute bleeding. The three drugs (HemAssist, PolyHeme, and Hemopure) that have reached phase 3 trials did not decrease transfusion requirements during surgery and were associated with significant adverse events, including increased risk of myocardial infarction and death.22–24 Infusion with crystalloid solutions to maintain circulating volume remains the standard of treatment in these patients when they experience decreased cardiac output and decreased blood volume during surgery.16 Management of intraoperative blood replacement requires careful assessment of the patient’s preoperative, operative, and postoperative hemodynamic and coagulation status. When appropriate, blood product transfusion should be used judiciously, with consideration of the potentially devastating complications that can ensue. Care should be taken to investigate and address the cause of all blood component deficiencies. Adequate fluid status must be maintained during surgery. Colloid solutions restore volume deficits more rapidly than do crystalloids and are less likely to induce tissue edema. It is, however, more costly, and it is associated with complications of its own. When crystalloid solutions are used, normal saline is preferred, because its isotonic fluid balance and higher sodium content help prevent cerebral edema and further brain injury. Finally, in surgeries where high volume blood loss is foreseen (e.g., extensive spinal operations) or barriers to adequate blood and volume replacement are anticipated (e.g., Jehovah’s Witnesses), various blood conservation measures must be used. These measures include cell saver, normovolemic hemodilution, nutritional and medical optimization of RBC production, logistical considerations to decrease iatrogenic blood loss, and careful surgical planning to minimize blood loss. KEY POINTS • The INR and PTT are used clinically to assess coagulation function and follow anticoagulant therapies. • A normal platelet count does not guarantee normal platelet function. • Platelet counts < 100,000 × 109 should alert the physician to potential challenges with intraoperative control of bleeding. • A normal value for bleeding time is 2 to 9 minutes. • Aspirin, nonsteroidal inflammatory agents, and clopidogrel alter platelet function. • Blood typing and blood crossmatch processes facilitate a low transfusion risk for patients. • However, transfusion-related complications may include acute and subacute hemolytic reactions, anaphylaxis, transmission of infectious disease, bacterial contamination, immunosuppression, febrile reactions, graft versus host disease, hypocalcemia, hypothermia, and coagulopathy. • Intraoperative assessment of blood volume requires determination of a patient’s preoperative volume status, monitoring of physiological parameters, and monitoring of the blood losses during surgery. • The intraoperative hematocrit may not be a reliable measure of blood loss if large volumes of crystalloid or colloid solution have been used. • A single unit of pRBCs will raise the hemoglobin by about 1 g/dL and the hematocrit by 3%. • Preoperative, intraoperative, and postoperative transfusion triggers should be determined for patients. • A single donor unit of platelets transfused will raise the platelet count by about 50,000/mm3. • One unit of FFP has a volume of 200 to 250 mL and an INR of 0.9 to 1.2. • Cell savers have a role in elective, nontumor surgery when a large volume of blood loss is expected. • Normovolemic hemodilution can be a useful preoperative strategy when a large volume of blood loss is expected. • Nutritional and medical optimization of RBC production may have a role when planning elective surgery in high-risk patients.
Principles of Blood and Volume Replacement
Preoperative Assessment
Laboratory Evaluation of Coagulation
Prothrombin Time and the International Normalized Ratio
Partial Thromboplastin Time
Platelet Count
Tests of Platelet Function
Blood Typing and Crossmatching
Transfusion-Related Complications
Intraoperative Assessment of Blood Status and Volume Replacement
Blood Factor Replacement
Packed Red Blood Cells
Platelets
Fresh Frozen Plasma
Cranial Surgery
Spinal Surgery
Intraoperative Cell Salvage
Normovolemic Hemodilution
Blood Replacement in the Jehovah’s Witness
Conclusion
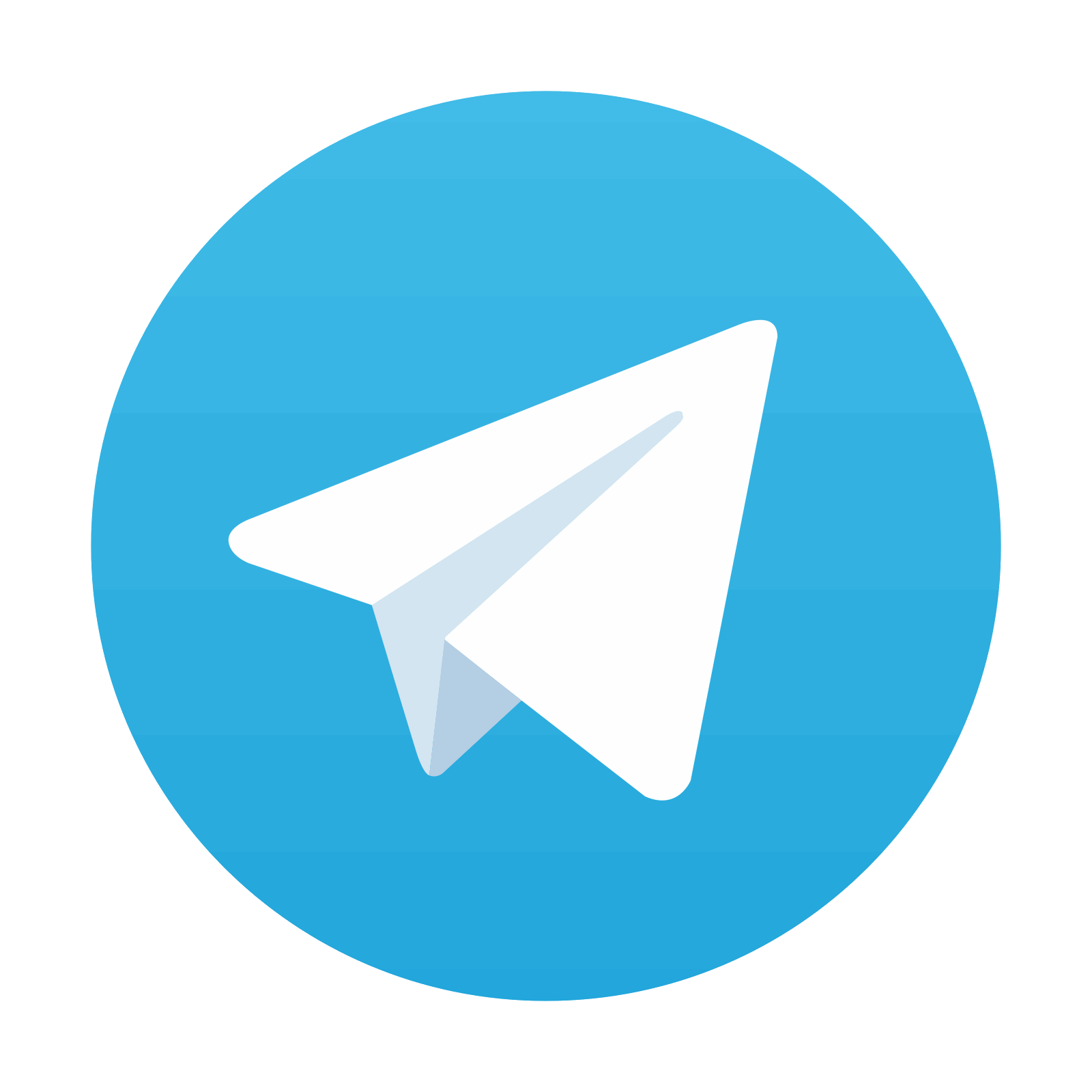