A 55-year-old male smoker with no known past medical history complained to family members of acute onset of severe headache, nausea, and stiff neck before becoming confused and lethargic at home. The patient was transported to the emergency department, where he was found to be confused but able to follow simple commands. A head CT was performed, demonstrating subarachnoid hemorrhage with mild hydrocephalus. CT angiogram reveals a 3 mm anterior communicating artery aneurysm. Upon return from the CT scanner, the patient was noted to be slower with commands, with sluggishly reactive pupils. What neurocritical care interventions are appropriate at this time?
Neuroscience intensive care units (NICUs) specialize in providing care for critically ill neurologic and neurosurgical patients.
NICUs have seen tremendous growth recently in the United States.
Despite this growth, at many US hospitals, ICU patients with primarily neurological conditions are admitted to general medical or surgical ICUs, with the neurology and neurosurgery teams co-managing the patients with the ICU teams.
This chapter provides an overview of neurocritical care and general critical care. Techniques are highlighted that may allow a consulting hospital neurologist to interface more effectively with the rest of the team providing care to critically ill neurology and neurosurgery patients.
There are multiple models for ICU care:
In a closed ICU, a single intensivist or team of intensivists has primary responsibility for the unit.
In an open ICU, multiple providers (eg, surgeons, etc.) admit and manage their patients with or without intensivists consulting.
In a cooperative ICU, some patients will be admitted to the intensivist team, and others may be admitted under multiple providers; typically, the intensivist team will round on or at least consult on all the patients.
The specific type of system and role of a consulting neurologist will vary from hospital to hospital; nevertheless, an understanding of neurologic and general critical care is essential to optimizing patient care in any of these settings.
The fundamental goal of an NICU is to improve the mortality rate and functional status of the most critically ill neurology and neurosurgery patients.
There are two basic reasons for NICU admission: to provide monitoring and to provide interventions that cannot take place elsewhere.
The specifics are institutionally dependent, and an ICU intervention in one facility may be successfully performed in a stepdown unit or on the floor in another.
Examples of monitoring include:
Frequent neurologic examination checks by nursing staff
Continuous arterial blood pressure monitoring
Intracranial pressure monitoring
Multimodality monitoring
Pulmonary artery catheters
Frequent lab draws
Interventions may include:
Mechanical ventilation
Vasopressor support
Antihypertensive infusions
Drainage of cerebrospinal fluid (CSF)
For many disease processes, NICU care has been demonstrated to improve outcomes,1including intracerebral hemorrhage, traumatic brain injury, and subarachnoid hemorrhage (SAH).
Even outside of a dedicated NICU, there are proven benefits of having neurointensivists as consultants.2
Intracranial pressure (ICP) is a common physiologic parameter monitored in the NICU.
ICP is a determinant of the cerebral perfusion pressure (CPP, defined as mean arterial pressure (MAP) minus ICP), which must be maintained to prevent secondary insults caused by ischemia.
Cerebral compliance is fairly limited, meaning that small increases in intracranial volume lead to a rapid rise in ICP and consequently a rapid reduction in CPP. This principle drives the Monro-Kellie doctrine, which states that the total volume of the brain, CSF, and cerebral blood volume must remain constant.
The goal of ICP monitoring is to alert the clinician as this occurs, allowing for medical or surgical therapies to reduce ICP and restore perfusion by either:
Reducing the volume of another intracranial component (brain tissue or CSF)
Increasing the total volume available (ie, craniectomy)
As mentioned, cerebral perfusion pressure (CPP) is defined as the MAP minus the ICP.
CPP may provide additional prognostic information beyond ICP alone.
Both elevations and depressions of CPP have been correlated with worse neurologic outcomes, presumably because the limits of cerebral autoregulation have been exceeded.
The default method of ICP monitoring is by placement of an external ventricular drain (EVD), which has the dual advantage of also allowing for drainage of cerebrospinal fluid.
Alternatives include placement of pressure monitors in the epidural and subdural spaces, and placement of an intraparenchymal pressure monitor, which is preferable from an accuracy standpoint.
Although several are being investigated, there are currently no well-validated noninvasive alternatives for ICP monitoring.
Measuring ICP is not without risk:
41% of patients demonstrate procedure-related hemorrhage on imaging, although these hemorrhages are often small and only rarely require intervention.3
Infection occurs in 0–22%4 of patients with EVDs, varying depending on how ventriculostomy-related infection is defined, the duration of device insertion, and possibly the use of antibiotics.
Decisions will often need to be made on a case-by-case basis.
Four common indications for EVD placement include:
Traumatic brain injury (TBI) patients with a GCS of <95
Subarachnoid hemorrhage (SAH) patients with altered mental status and evidence of hydrocephalus on CT scan
Primary intraventricular hemorrhage or intracerebral hemorrhage with intraventricular extension
Patients with obstructive hydrocephalus
Severe TBI patients with small ventricles may be better candidates for an intraparenchymal monitor.
Protocolized treatments based on ICP monitoring versus clinical examination plus imaging were compared in the well-publicized multicenter BEST TRIP randomized trial.6
Patients with severe TBI were included.
Protocolized treatment based on ICP monitoring did not demonstrate a benefit in functional outcomes, mortality, or ICU length of stay.
However, days of treatment directed at improving cerebral physiology (ie, hypertonic fluids, hyperventilation) were lower in the ICP-monitoring group, suggesting improved resource utilization with this protocol.
CPP was not reported.
This study does not necessarily imply that monitoring ICP is never beneficial—merely that treating elevated ICP based on clinical signs versus formal monitoring did not significantly change outcomes.
Direct measurement of ICP in the setting of a focal lesion (hemispheric stroke or hemorrhage, etc.) is generally done only in rare situations, as a global ICP number may be high or low independent of the focal mass effect of the lesion on brainstem structures. Such situations are generally managed by following the progression of a patient’s neurologic examination.
The role of ICP monitoring for patients with hypoxic-ischemic injury or hepatic encephalopathy is not yet well-defined, and no standardized recommendation can be made in such situations.
Normal ICP is less than 20 mmHg, and levels above this have the potential to compromise cerebral perfusion.
However, the patient’s MAP and CPP must be considered as well.
Prolonged elevations are much more likely to cause significant damage than transient ones.
Isolated ICP elevations should not necessarily be reflexively treated, but rather considering as part of an entire clinical picture, including the degree of loss of autoregulation from existing injury, systemic physiologic variables, additional monitoring information, and the patient’s neurologic examination.
Information regarding cerebral compliance can be obtained from the ICP waveform, which has three distinct peaks (Figure 21.1): 7
P1, representing transmission of systolic blood pressure to the ventricles via the choroid plexus
P2, thought to represent the compliance of the brain tissue
P3, representing the closing of the aortic valve.
P2 becomes higher as cerebral compliance decreases, and a P2 elevation higher than P1 represents a significant decrease from normal compliance.7
Many patients with brain injuries may have “plateau waves,” transient spikes of extremely elevated ICP that last for a few minutes and resolve spontaneously.
The presence, height, and frequency of plateau waves do not necessarily indicate a worse prognosis and these waves do not necessarily require treatment.
Prolonged plateau waves may still impair cerebral perfusion and result in ischemia.
Elevating the head of the bed:
Decreases ICP by promoting venous drainage since, as previously discussed, small changes in intracranial volume result in significant changes in ICP,
In some cases, may decrease CPP as well.
Hyperventilation
Reduces ICP by inducing cerebral vasoconstriction and thereby reducing cerebral blood volume.
May cause ischemia8 due to this vasoconstriction, and has been shown to reduce brain tissue oxygenation in certain situations.9
May cause deleterious effects on neurologic outcomes in patients with traumatic brain injuries when utilized for prolonged durations.10
Has diminishing beneficial effects on ICP over time, as renal buffering of the respiratory alkalosis occurs.11
Current Brain Trauma Foundation guidelines:12
Strongly discourage hyperventilation below a PaCO2 of 25 mmHg.
Discourage prophylactic moderate hyperventilation (PaCO2 31–35 mmHg).
Overall, hyperventilation to limit ICP should be:
Moderate only, with a goal PaCO2 of 32–40 mmHg
Thought of as a temporizing measure12 while more definitive medical or surgical therapy is pursued
Fever avoidance
Fever is a common consequence of a variety of neurologic injuries, including stroke, SAH, TBI, and hypoxic-ischemic injury.
A large meta-analysis found fever across multiple neurologic conditions to be correlated with worse outcomes, including:13
Increased mortality
Worsened neurologic outcomes on a variety of scales
Increased ICU length of stay
Increased hospital length of stay
Medications such as acetaminophen and nonsteroidal anti-inflammatory medications are less likely to be effective in fever control in brain-injured patients due to impairment of the normal thermoregulatory mechanisms.14
Other methods of fever control include surface cooling and intravascular cooling.
These methods may trigger shivering that requires medical treatment to allow for optimal cooling. Pharmacologic options include:
Buspirone
Magnesium
Meperidine
Dexmedetomidine
Sedatives
Neuromuscular blockade agents
Given that fever is one of the common signs of infection, surveillance for infection in patients undergoing therapeutic normothermia is necessary.
There is currently no evidence-based or standardized approach to such surveillance.
Prospective data linking fever reduction to improvements in outcome are limited.
One study in patients with a variety of cerebrovascular diseases found that endovascular cooling resulted in better fever control without significant complications, but did not improve outcomes.15
Further outcomes data are needed.
Osmotic therapy
Osmotic therapy decreases ICP by removing water from the brain tissue.
The brain is 80% water, making its volume much more responsive than that of other tissues to changes in water content.
Causes water to freely diffuse down a concentration gradient that is artificially created between the brain and the vasculature.
Requires agents with a high reflection coefficient (ie, one to which the blood–brain barrier is largely or completely impermeable).
Requires an intact blood–brain barrier:
Osmotic therapy removes water primarily from the portion of the brain that is not damaged, as the solute crosses into the parenchyma in damaged tissue.
Osmotic therapy may have limited effect on vasogenic edema surrounding a mass lesion.
Requires maintenance of hyperosmolarity to prevent rebound edema.
Choice of agent:
Mannitol and hypertonic saline are two frequently used agents.
Studies comparing the effects of hypertonic saline versus mannitol on ICP have yielded conflicting results,16,17 but the effect size has generally not been significant enough to prefer one over the other.
Patients who show insufficient response to one agent may benefit from having the other added.
Mannitol:
May be administered peripherally
Is an osmotic diuretic and may result in hypotension
May cause renal failure
Repeated dosing of mannitol should be based on the osmolar gap.18
The osmolar gap is defined as measured osmolarity – (2 * [Na] + [Glucose]/18 + BUN/2.8 + [Ethanol]/3.7)
Mannitol may be safely redosed when the osmolar gap is less than or equal to 10
Hypertonic saline:
Should generally be administered through central access outside of immediately life-threatening emergencies
Causes intravascular volume expansion and may result in CHF
May have immunomodulatory properties,19 although the clinical significance of this effect is unclear.20
Common formulations include 1.5%, 3%, and 23%.
23% should be reserved for immediately life-threatening ICP crises, and is usually administered as a bolus over 5–15 minutes.
This bolus dose may be repeated rapidly if needed to treat a life-threatening ICP crisis.
Weaker concentrations may be used as continuous infusions to maintain hyperosmolality.
Serum sodium level should be maintained at no more than 160.
Avoiding internal jugular catheterization, if a patient needs a central venous catheter:
Limits the possibility of obstructing outflow, particularly in the event of a catheter-associated deep venous thrombosis.
Studies regarding the effect of catheter site location on ICP have not been performed, but absent other significant considerations, the common NICU practice of preferring the subclavian site for neurologically injured patients should be followed.
In most patients, EVDs should be left open for drainage rather than being clamped.
Exceptions include patients with unsecured aneurysmal SAH (where drainage may theoretically cause rebleeding) and posterior fossa lesions (where drainage may cause upward herniation). In these patients, drainage should only be performed for sustained elevations of ICP.
In most patients, the drain should initially be placed 10 or 15 cm above the external auditory meatus.
Overly aggressive drainage may cause subdural hemorrhages/hygromas or low-pressure headaches.
The drain should be clamped for any maneuvers involving patient repositioning to avoid sudden overdrainage.
EVDs should be gradually weaned in patients whose tolerance of discontinuation is unclear:
At all stages of weaning, patients should be monitored for signs of worsening hydrocephalus, including confusion, lethargy, worsening headaches, worsening ability to deviate eyes upward, or more sluggish pupillary responses.
The initial steps should be to raise the drain by 5 cm daily while following CSF output.
If 20 cm is tolerated and CSF output is decreasing, consider clamping the drain and opening only for sustained ICP elevations.
If clamping is tolerated for 24 hours, a head CT should be obtained to exclude worsening hydrocephalus, and if none is found, the EVD may be discontinued.
The timing of EVD weaning should be dependent on the underlying disease process:
In SAH, weaning should generally not take place until vasospasm has resolved (as treatment with vasodilators may raise ICP).
In ischemic stroke, cerebral edema typically reaches a maximum 3–5 days after infarction.
In intracerebral hemorrhage, swelling may last as long as 8–10 days.
In both SAH and intracerebral hemorrhage patients, the persistent presence of intraventricular hemorrhage should be factored into decision-making as well, as its presence predisposes to persistent hydrocephalus.
EVDs for posterior fossa decompressions should be left in place until swelling has subsided and the fourth ventricle is clearly open.
Surgical decompression
If a patient has a surgical mass lesion (ie, an epidural hematoma), surgical decompression may be required. In such cases, medical therapy to limit intracranial pressure should be considered a temporizing measure only while pending definitive surgical management.
Surgical decompression removes part of the skull, mitigating the limitations imposed by the Monroe-Kellie doctrine as the cerebral contents are no longer confined to a fixed space. Evacuation of mass lesions, such as epidural hematomas, may also take place.
Surgical decompression may be considered in several conditions:21
Traumatic brain injury
Surgical treatment is the clear standard of care for TBI patients with mass lesions such as epidural hematomas exhibiting significant mass effect, and such patients should proceed to operative intervention if consistent with goals of care.
Bifrontal craniectomy has been recommended for patients with diffuse post-traumatic cerebral edema and elevated ICP refractory to medical management.22
There is some controversy surrounding this recommendation, as it may decrease mortality without improving neurologic outcomes.21
In the DECRA trial, a well-publicized randomized multicenter trial of craniectomy versus medical therapy for refractory ICP elevations in diffuse post-traumatic cerebral edema:23
Craniectomy did not change long-term neurologic outcomes.
Craniectomy was associated with lower ICP, fewer days of mechanical ventilation, and fewer ICU days.
Of note, patients with surgical mass lesions were excluded.
Many of the patients who were screened did not meet enrollment criteria because their ICP elevations were successfully managed with medical therapy.
Aneurysmal SAH
Several studies have suggested that decompressive craniectomy may benefit a select group of more severe aneurysmal SAH patients.24,25
Specific indications and timing of surgical management require further clarification.
Steroids
When given to patients with vasogenic cerebral edema from intracranial tumors, steroids may improve focal neurologic signs, mental status, and decrease elevated ICP.9
Steroids have demonstrated either no effect or a detrimental effect in other conditions resulting in elevated ICP, including TBI and intracerebral hemorrhage.9
Hypothermia
One study of therapeutic hypothermia in TBI patients found no improvement in neurologic outcomes but reductions in ICP in the treatment group.26
Due to its potential complications, hypothermia should not be routinely used to lower ICP, but may be considered on a case-by-case basis pending further study.
Sedation and paralysis
ICP elevations caused by agitation, posturing, or coughing may be minimized by adding sedative or paralytic medications.9
These interventions should only be used when absolutely necessary, as they result in loss of a reliable neurologic examination.
These medications also may cause complications including myopathy and polyneuropathy.
Barbiturate coma
Induction of a barbiturate coma is associated with significant complications, including hypotension and increased infection rate, and complicates management by preventing effective neurologic examinations. Therefore, barbiturate coma induction should be avoided if possible.
CASE 21-1 (continued)
The patient is intubated for airway protection and undergoes mild hyperventilation while mannitol is being administered. Neurosurgery is consulted and places an EVD, with a high initial opening pressure. After initial CSF drainage, his ICP falls to normal range, and his EVD is clamped. He is admitted to a neuroscience ICU for further management. Upon arrival, he develops convulsive status epilepticus, requiring lorazepam, levetiracetam, and ultimately a benzodiazepine infusion. His rhythmic motor activity ceases, but he is no longer following commands despite a normal ICP. What monitoring should be considered in this patient?
Continuous EEG monitoring should be considered for any patient with generalized or complex partial seizures who does not recover to their neurologic baseline (to identify ongoing seizure activity causing persistent altered mental status).
Any patients who are intubated for status epilepticus should have continuous EEG monitoring in order to titrate antiepileptic medications and sedative infusions.
EEG monitoring has also been used in a variety of other conditions:
Patients with acute neurologic illnesses:
Many patients with neurologic insults develop seizures due to their acute insult.
This includes acute ischemic stroke, SAH, intracerebral hemorrhage, TBI, and postoperative neurosurgical patients.
These seizures may be subclinical, and, in many cases, have been shown to be correlated with worse prognosis.
EEG monitoring should be considered for all patients with these conditions and an otherwise unexplained deterioration in mental status, as their seizures may be subclinical (eg, electrographic only).
Detection of such events occurs more frequently with continuous EEG monitoring than routine EEG due to its intermittent nature.27
Delay to diagnosis of nonclinical seizures in ICU patients results in increased mortality.28
SAH patients
EEG changes occur within seconds of changes in cerebral blood flow.29,30
Multiple retrospective studies have demonstrated the ability of EEG monitoring with quantitative techniques to detect ischemia caused by vasospasm in SAH.31–33
In some cases this occurs prior to clinical deterioration.31,32
Alpha/delta ratios,33defined as the ratio of power in the alpha versus delta ranges, and alpha variability32 have both been found to have good sensitivity for vasospasm in SAH.
Post-cardiac arrest patients
Continuous EEG monitoring has become the standard of care for post-cardiac arrest patients undergoing therapeutic hypothermia.
Such patients are at high risk for seizure and often undergoing continuous neuromuscular blockade to prevent shivering, resulting in a complete loss of their clinical examination.
Although not 100% sensitive or 100% specific, EEG monitoring may yield significant prognostic information, even when performed during therapeutic hypothermia and particularly when performed after rewarming:34
A nonreactive background EEG has been shown to be incompatible with neurologic recovery.
Burst-suppression patterns, generalized periodic epileptiform discharges, focal or generalized seizures, and status epilepticus are all associated with a poor prognosis.
Excessive beta or theta slowing was associated with improved neurologic prognosis.
Interpreting continuous EEG information
There is a spectrum of EEG findings and intermediate patterns between normal and frank seizure.
The significance of these intermediate patterns is an area of active research.
Transcranial Doppler (TCD) ultrasound
Cheap, easily obtainable, dynamic, and may be obtained at the bedside
Limited in that it primarily provides information about the proximal vessels
Often used to detect vasospasm in subarachnoid hemorrhages prior to clinical deterioration.35,36
Jugular venous oxygen saturation (SjvO2) monitoring
Provides a continuous measure of global brain oxygen delivery relative to demand
Operates on the principle that lower oxygen delivery or high demand increases the oxygen extraction coefficient and decrease the SjvO2
Requires placement of the catheter in the superior part of the jugular bulb to avoid mixing of intracranial venous blood with return from the facial veins
Has been studied primarily in TBI and SAH
Near-infrared spectroscopy (NIRS)
Transmits infrared light through the skull and uses differences in reflectance between oxyhemoglobin and deoxyhemoglobin to determine cerebral oxygenation
Has the advantages of being continuous and noninvasive
Multiple variables such as hemoglobin concentration, skull thickness, and alterations in CSF may limit the accuracy of results.
Brain tissue oxygentation (PbtO2 monitoring)
A probe placed directly into the brain parenchyma provides measurement of the partial pressure of oxygen in that brain tissue.
Only information regarding the local tissue surrounding the probe is provided.
Studies correlating tissue oxygenation with clinical outcomes have yielded varying results, and further study is needed prior to routine clinical use.
The recent BOOST 2 study demonstrated that protocol-based treatment of low PbtO2 in TBI patients resulted in a significant decrease in the amount of time spent below a PbtO2 of 20 mmHg (which has been shown to correlate with worse outcomes).
This study demonstrated a trend toward decreased mortality and improved neurologic outcome, and future studies with larger sample size are planned.
Cerebral microdialysis
A microdialysis catheter is placed into brain tissue, and molecules from the brain tissue diffuse down their concentration gradients; the dialysate is then recovered and analyzed.
Changes in concentrations of lactate, pyruvate, and glycerol provide information regarding aerobic versus anaerobic cell metabolism as well as cell death.
Limitations of this technique are that it is invasive, it provides information regarding local brain tissue only, and the information provided is delayed until the dialysate is recovered.
Overall, as all the aforementioned methods have their unique strengths and unique limitations and none provides a comprehensive evaluation of all possible aspects of cerebral perfusion and cellular metabolism, multiple sources of information must be integrated into a complete clinical picture to support treatment recommendations for specific patients.
CASE 21-1 (continued)
The patient undergoes continuous EEG monitoring, revealing ongoing nonconvulsive status epilepticus. Additional antiepileptics are added, resulting in control of his seizure and gradual improvement in his mental status. As his mental status improves, he becomes increasingly agitated, resulting in difficulty with ventilation and intermittent ICP elevations. What medications should be considered?
At times, the patient may become agitated and require sedative medication for comfort or to prevent patients from removing necessary medical equipment.
Sedatives may also be needed to perform procedures.
Sedative use has been shown to increase delirium.
Dose and duration of sedative use should be minimized.
Daily interruptions of sedation have been shown to decrease ventilator days and length of stay,37 as well as mortality,38 and should generally be performed in the absence of contraindications.
Sedation interruptions also allow physical therapy and better return to functional status.
Recent studies have challenged the paradigm of continuous infusions of sedative medications and have found intermittent as-needed bolus dosing to be associated with less ventilator days and shorter ICU and hospital lengths of stay.
In general, lighter levels of sedation should be targeted to improve patient outcomes.39
When possible, identifying and treating a specific cause for agitation, ie, pain, is preferable to sedative use.
The most commonly used sedatives in the ICU are benzodiazepines and propofol.
Current recommendations favor propofol over benzodiazapenes,39 based on a meta-analysis demonstrating reduced ICU lengths of stay with nonbenzodiazepine sedatives.
Benzodiazepines
Midazolam and lorazepam are two of the most commonly used
Target the GABAA receptors, resulting in sedation, anxiolysis, and amnesia
Also have anticonvulsant properties
Are metabolized by the liver and may have prolonged effects in patients with hepatic dysfunction
Several benzodiazepines (midazolam, diazepam) have active metabolites that are renally cleared
Lorazepam uses propylene glycol as a diluent, potentially resulted in toxicity with large, prolonged, or repeated dosing.
Propofol
Binds to multiple neuronal receptors, including GABAA, and nicotinic and muscarinic receptors
Has a similar effect profile to benzodiazepines, although causes less amnesia
Has quicker offset than many benzodiazepine sedatives, allowing for easier interruption for neurologic examinations
Has the potential to result in hypotension and respiratory depression
May result in propofol infusion syndrome, particularly with prolonged use
Causes metabolic acidosis, hypertriglyceridemia, and hypotension
Carries up to a 33% mortality rate
Treatment is discontinuation of propofol and supportive care
Dexmedetomidine
Binds to central alpha2 receptors
Patients are more easily aroused when sedated with dexmedetomidine than other agents
Has the potential to cause hypotension and bradycardia
Less potential for respiratory depression than other agents
May reduce opioid requirements
Many ICU patients experience pain, both peri-procedurally and with routine ICU care.
Opiate pain medication should be used sparingly to limit interference with neurologic examination, depression of mental status, and delirium.
All intravenous opiates are equally effective when titrated to similar pain intensity endpoints.
Opiates may result in respiratory suppression as well as hypotension.
Hypotension is histamine-mediated and not amenable to treatment with reversal agents.
Synthetic opiates such as fentanyl may be preferred if hypotension is a concern.
Loss of pain-induced sympathetic stimulation may still result in hypotension when these agents are administered.
Hypotension should be treated with intravenous fluids and/or vasopressors and should not necessitate withholding analgesia.
Gabapentin or carbamazepine should be used for neuropathic pain.
Neuromuscular blocking agents prevent transmission across the neuromuscular synapse, preventing muscle contraction.
Succinylcholine is a depolarizing neuromuscular blocker that binds to the postsynaptic acetylcholine receptor to depolarization and muscle contraction and then prevents further contraction.
The remaining neuromuscular blocking agents, such as rocuronium and vecuronium, block the acetylcholine receptor, preventing depolarization and contraction.
Adult skeletal muscle retains the ability to produce fetal acetylcholine receptors. These receptors are upregulated with prolonged use of neuromuscular blocking agents, resulting in tachyphylaxis.40
Neuromuscular blockers may be used in acute respiratory distress syndrome (ARDS) to facilitate mechanical ventilation by eliminating the effects of the chest wall musculature on a patient’s already decreased lung compliance.
Neuromuscular blockers may be used for similar reasons in ventilated patients with refractory status asthmaticus.
Neuromuscular blockade may be considered in patients with elevated ICP to prevent worsening intracranial hypertension with coughing and gagging in response to suctioning and other routine ICU care. These agents should only be used for this purpose when other therapies have been exhausted.
Neuromuscular blockade agents may often be used to limit or prevent shivering in patients undergoing therapeutic hypothermia or targeted temperature management.
At least one study found that neuromuscular blockade after cardiac arrest was associated with increased survival.41
Neuromuscular blockade should not be used to control seizure activity, as it prevents the motor activity but does not affect the underlying neural activity.
Neuromuscular blockade interferes with neurologic assessment.
Prolonged duration of effect may occur in patients with hepatic or renal dysfunction.
Neuromuscular blockers may contribute to critical illness polyneuropathy and myopathy.42
CASE 21-1 (continued)
The patient is placed on intermittent as-needed bolus dosing of fentanyl and midazolam to control pain and agitation while limiting his total medication dosing. At conventional angiography, he is confirmed to have a 3-mm ruptured anterior communicating artery aneurysm, which is successfully coiled. His EVD is opened at 20 cm above the tragus. His blood pressure after angiography is 122/76. What cardiovascular monitoring and medications may be used in the NICU, and which are indicated for this specific patient?
An important role of the neurointensivist is optimizing a patient’s cardiovascular status to limit secondary insults with respect to neurologic dysfunction.
Neurologic injuries may alter the traditional hemodynamic management principles often otherwise followed, such as maintaining cerebral perfusion pressure in polytrauma patients with traumatic brain injuries,43 for whom blood pressure goals may otherwise be less stringent.
A thorough understanding of cardiovascular physiology and treatment options facilitates management recommendations for neurologically injured patients.
Depending on the underlying disease process, interventions may be targeted toward optimizing perfusion, controlling arterial hypertension, and/or limiting the chances of further bleeding.
End-organ perfusion depends on vascular resistance and cardiac output, which is affected by:
Heart rate
Preload
Contractility
The brain is extremely sensitive to variation in blood flow and is equipped with a variety of autoregulatory mechanisms to maintain constant blood flow despite significant variations in mean arterial pressure. Of note:
Limits of autoregulation vary significantly in healthy individuals.
Autoregulatory mechanisms can become disrupted by a variety of neurologic and nonneurologic disease states.
When the safety net of autoregulation is no longer intact, precise control over a patient’s cardiovascular status to maintain perfusion becomes even more important.
Managing neurologic injuries associated with a cardiomyopathy is even more challenging.
This occurs commonly in patients with SAH, although case reports exist in a variety of other neurologic conditions as well.
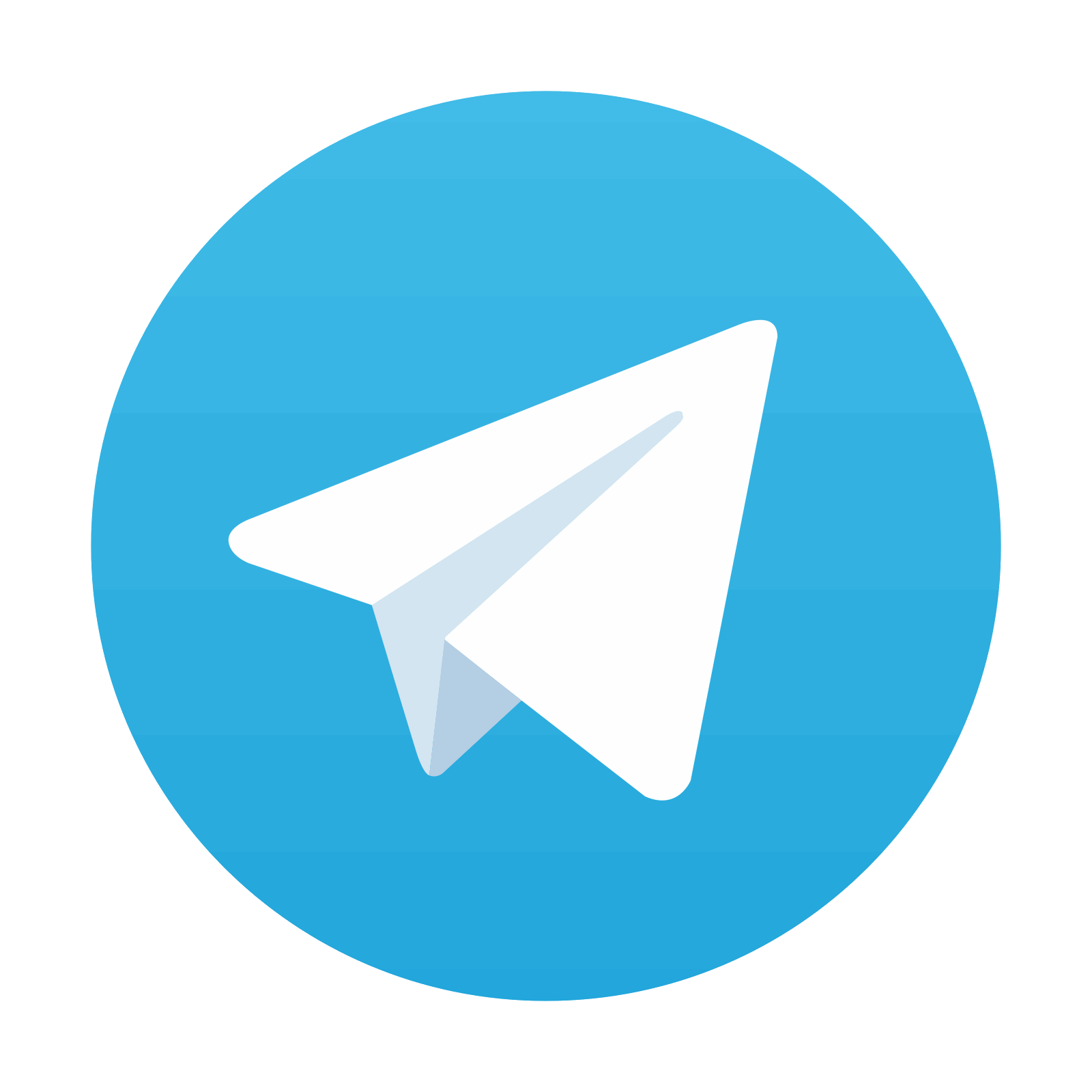
Stay updated, free articles. Join our Telegram channel

Full access? Get Clinical Tree
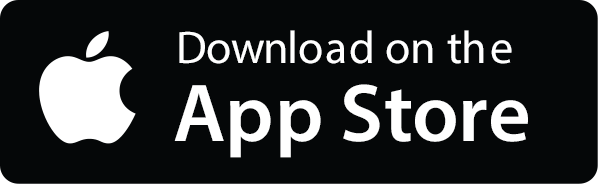
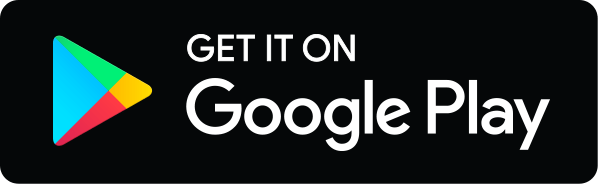