Preoperative surgical evaluation
The initial evaluation of a patient with suspected glioblastoma includes history and physical examination, anatomic imaging, and symptom management with seizure medications and corticosteroids. Clinical presentation of glioblastoma is highly variable, depending on tumor size, location, and the amount of peritumoral edema. Glioblastomas are rarely discovered incidentally (3.8% of patients). Seizures and neurocognitive changes are the most common presenting symptom, occurring in more than 70% of patients. Blunted affect, changes in personality, and neurocognitive dysfunction may be more prevalent in patients with frontal tumors. Speech and language deficits occur in 58% of patients from tumors affecting cortical or subcortical language pathways in the dominant hemisphere. Additional symptoms include headaches, memory loss, sensory dysfunction, and visual field deficits.
Initial imaging of patients with suspected glioblastoma begins with brain MRI with and without enhancement. T1-weighted images with and without gadolinium enhancement are critical, because they allow the estimation of anatomic localization, tumor necrosis, vascularity, and amount of mass effect. T2-weighted, fluid-attenuated inversion recovery (FLAIR) images and diffusion-weighted MRI sequences show the extent of surrounding vasogenic edema. Glioblastomas are typically avidly enhancing on FLAIR signals outside of tumor boundaries, showing the degree of surrounding peritumoral edema. Structural and functional imaging, such as functional MRI (fMRI), diffusion tensor imaging (DTI), and magnetoencephalography (MEG), may be useful when evaluating tumors within presumed functional areas.
Corticosteroids are commonly used to control tumor-associated vasogenic edema. Dexamethasone is commonly used, with doses ranging between 2 and 24 mg daily. Corticosteroids may improve focal neurologic deficits and level of consciousness, and relieve headaches. Major side effects of corticosteroids include depression, osteoporosis, and immunosuppression; therefore, they should be administered only if necessary and at the lowest dose possible. Recent data suggest that higher doses of corticosteroids may lead to resistance to alkylating chemotherapy such as temozolomide. Patients presenting with seizures should be started on an anticonvulsant while balancing side-effect profiles and drug-to-drug interactions. Phenytoin was historically the first-line antiepileptic agent; however, levetiracetam is now commonly used because of its low toxicity, lack of need to monitor serum levels, and minor side-effect profile.
Preoperative planning
Management of intrinsic brain tumors begins with surgery intended to establish the diagnosis and maximal safe resection. In order to minimize perioperative risk, careful planning should occur before the patient is taken to the operating room. High-quality contrast MRI scans with and without enhancement are vital for developing the optimal operative plan. Preoperative imaging provides knowledge about glioblastoma location as well as its relationship to vascular structures and potential functional areas. Structural MRI and fMRI can be reconstructed to create three-dimensional models to establish a safe surgical corridor during surgery. fMRI, DTI tractography, and MEG MRI provide valuable information regarding the interface of tumor tissue with adjacent functional cortical and subcortical pathways ( Fig. 12.1 ). fMRI produces the blood oxygenation level–dependent (BOLD) signal, which marks cortical regions associated with task performance (motor, expressive language, receptive language). DTI tractography establishes white matter tracts surrounding the glioblastoma and can be helpful in determining whether functional pathways are infiltrated or displaced by tumor mass effect. DTI of white matter pathways is particularly important for language pathways, including corticospinal tract, superior longitudinal fasciculus, arcuate fasciculus, uncinate fasciculus, inferior orbitofrontal fasciculus, and optic pathways. Preoperative clinical evaluation should include baseline language and sensorimotor assessment performed 24 to 48 hours before surgery. Many glioblastomas are within or adjacent to potential functional areas, making it difficult to balance achieving an excellent extent of resection with minimizing postoperative deficits. Preoperative planning must therefore take into consideration the range of available approaches, including awake language and motor brain mapping craniotomy, asleep motor mapping craniotomy, image-guided resection, and use of the intraoperative MRI (iMRI).

Intraoperative standards of resection
Over the past 2 decades, numerous studies have enhanced the understanding of the impact of extent of resection on overall and progression-free survival in patients with glioblastoma. The decision to offer maximal safe resection depends on factors such as performance status, patient age, and tumor location and size. There are several intraoperative tools and strategies that have been developed to both enhance safety and improve the extent of tumor resection. These options include neuronavigation, intraoperative brain mapping, use of iMRI, and fluorescence-guided surgical resection.
Neuronavigation
Functional and anatomic image guidance (neuronavigation) is an important part of both preoperative planning and intraoperative tumor resection. It is used to plan size and location of the craniotomy, and to estimate extent of resection during glioblastoma resection. Image guidance allows a generalized assessment of vascular and functional pathway displacement in relation to the tumor. Functional neuronavigation refers to the integration of DTI tractography, transcranial magnet stimulation, MEG, or fMRI for the identification of cortical and subcortical areas of functional significance ( Fig. 12.2 ). Intraoperative functional neuronavigation can therefore display plots of functional areas and their relationships to the tumor using preoperative gadolinium-enhanced MRI. However, these studies are tend to be inaccurate because of distortion caused by mass effect, individual anatomic variability, or functional reorganization caused by cortical and subcortical plasticity. fMRI has a sensitivity and specificity of 91% and 64% for identification of Broca area, 93% and 18% for identification of Wernicke area, and close to 100% for identification of the primary motor cortex. Uncoupling of the BOLD signal in highly vascular tumors such as glioblastoma makes interpretation of fMRI results difficult. Resting state coherence measured with MEG is a noninvasive measure of functional connectivity of the brain. Glioblastomas with decreased resting state connectivity have a low risk of postoperative neurologic deficits, whereas those with increased resting state connectivity are associated with higher risk of postoperative neurologic deficits. It is advantageous to preoperatively and intraoperatively visualize functional pathways and their spatial relationships to a glioblastoma.

Awake Craniotomy and Intraoperative Brain Mapping
Direct cortical and subcortical stimulation mapping allows the identification of language, sensorimotor, and cognitive functions during surgery. Proper patient selection is critical and patients are considered for an awake craniotomy if they have a supratentorial intrinsic brain tumor located within or adjacent to regions presumed to have language or sensorimotor function on preoperative imaging. Proper patient selection is critical to maximizing perioperative safety. Given the limitations of structural and functional imaging, glioblastomas presumed to be within functional sites on preoperative anatomic imaging are never an absolute contraindication to attempting intraoperative mapping.
Several factors are associated with increased perioperative risk during awake brain tumor surgery. Absolute contraindications to an awake craniotomy include an uncontrolled persistent cough, severe dysphasia (ie, >25% naming errors despite a trial of preoperative dexamethasone and mannitol), large glioblastomas with mass effect resulting in more than 2 cm of midline shift, or hemiplegia with less than antigravity motor function. In the remainder of circumstances there are solutions and strategies to these obstacles. Patients with severely impaired preoperative language or motor function (>25% naming errors and <2 of 5 motor function) may be treated with high-dose corticosteroids (dexamethasone, 4–8 mg intravenously every 6 hours) and/or osmotic diuretics (mannitol 20%, 30 g every 6 hours for 48–72 hours) followed by reassessment of preoperative function. Obese patients, including individuals with body mass index greater than 35, may be treated with a laryngeal mask airway to prevent hypercapnia. Patients with generalized anxiety or severe untreated psychiatric history should be treated with antidepressant and mood-stabilizing medications before surgery. All seizures should be well controlled before an awake craniotomy, given the risk of intraoperative stimulation-induced seizures during intraoperative mapping. Intraoperative seizures may be treated with topical iced Ringer solution applied to the cortex. In addition, intravenous (IV) propofol, diazepam, or lorazepam should be ready for rapid administration as needed. Nausea should be treated with antiemetic medications such as ondansetron hydrochloride or scopolamine.
Preoperative evaluation before an awake craniotomy for glioblastoma includes functional and anatomic imaging, baseline language and sensorimotor testing, neuroanesthesia evaluation, and patient counseling and education. Baseline language assessments are performed by surgical neurophysiology or speech pathology 24 to 48 hours before surgery for tasks including picture naming, responsive naming, or reading, depending on tumor location.
Specialized neuroanesthesia is critical for completion of a safe awake craniotomy, because it ensures clear communication between neurosurgeon, anesthesiologist, speech pathologist, and other members of the mapping team. Surgery commences with the appropriate patient monitoring and premedicating with midazolam, fentanyl, or dexmedetomidine before positioning. Sedation is achieved with propofol (up to 100 μg/kg/min) or dexmedetomidine (up to 1 μg/kg/min) and remifentanil (0.07–2.0 μg/kg/h). Sedation begins during Foley catheter insertion and Mayfield head holder pin placement. A scalp block using a mixture of 1% lidocaine with 1:100,000 epinephrine and 0.5% bupivacaine is applied by the surgical team. Optimal head positioning usually involves a small amount of neck extension (for airway patency), which allows ease of operation, patient comfort, and optimal airway patency. A dedicated IV line is filled with a 1-mg/kg bolus of propofol if needed for suppression of an intraoperative grand mal seizure. Topical ice-cold Ringer lactate solution is available on the surgical field at all times for seizure suppression. After skin incision and removal of the bone flap, all sedatives are held or reduced before dural opening.
Intraoperative mapping originally involved large craniotomies with the goal of finding positive language and motor sites. The overall goal was to perform a focused exposure encompassing the lesion plus a 2-cm margin. Craniotomies are now focused to open only the dura overlying the lesion with a reliance on negative mapping, which avoids cortical injury to the surrounding brain. A dural block can be placed using a 30-gauge needle with 1% lidocaine to infiltrate the region around the middle meningeal artery. Following removal of the bone flap, sedating medications are discontinued or greatly reduced to ensure that the patient is cooperative before dural opening. If the brain is tense, the patient is asked to take additional controlled breaths, mannitol is given, and the head of the bed raised.
Cortical stimulation depolarizes a focal area of brain, which excites local neurons via diffusion of current using both orthodromic and antidromic propagation. Bipolar stimulation using a 2-mm tip with 5 mm of separation allows for local diffusion and more precise mapping. Mapping begins with a stimulation current of 1.5 to 2 mA using a constant-current generator that delivers 1.25-millisecond biphasic square waves in trains of 2 to 4 seconds at 50 or 60 Hz. Numerical markers are placed on the surgical field spaced 1 cm apart ( Fig. 12.3 ). Electrocorticography is used to monitor for subclinical seizure activity and detect after-discharge potentials, which improves mapping accuracy. All language tasks are repeated at least 3 times per cortical site, and a positive site is defined as the inability to count, name objects, or read words during stimulation at least 66% of times. Language mapping seeks to identify sites responsible for speech arrest, anomia, and alexia with stimulation testing. Speech arrest is defined as discontinuation in number counting without simultaneous motor response. Dysarthria can be distinguished from speech arrest by an absence of involuntary muscle contractions affecting speech.

Intraoperative MRI
MRI-guided surgery has been shown to enhance the extent of glioblastoma resection and therefore patient survival. Neuronavigation becomes less reliable during surgery. Therefore the rationale for the use of iMRI combined with conventional neuronavigation-guided resection is the avoidance of brain shift caused by cerebrospinal fluid loss and tissue edema. Numerous studies have attempted to quantify the effect of iMRI on extent of glioblastoma resection. Both iMRI and awake craniotomy are tools to maximize extent of resection while limiting neurologic deficits; however, there have been no head-to-head comparison studies. Both approaches have been proved safe and effective, but patient selection is critical. Awake brain mapping surgery allows real-time language and sensorimotor mapping and monitoring. However, patients with relative or absolute contraindications might be at increased risk. The primary advantage of iMRI is the ability to acquire updated anatomic and structural imaging throughout the procedure. Patients with cardiac comorbidities might be at increased risk during iMRI surgery given interference between telemetry and the operating room MRI equipment. It is becoming increasingly customary to use awake brain mapping within an MRI operating suite. Extent of tumor resection must be measured against perioperative risks, quality of life, and long-term neurologic disability.
Fluorescence-guided Surgery Using 5-Aminolevulinic Acid
It has been well established that most glioblastoma recurrences occur within a centimeter of the tumor resection cavity. In addition, most neurosurgeons overestimate the degree of resection they are able to achieve and do not have access to iMRI. 5-Aminolevulinic acid (5-ALA) is an inexpensive nonfluorescent amino acid precursor that produces an accumulation of fluorescent protoporphyrin IX in glioblastoma cells. Intracellular protoporphyrin IX level peaks 6 hours after administration and remains increased for 12 hours. Protoporphyrin IX has an absorption band strongest in the 380 ± 420 nm spectrum, emitting red fluorescence at 635 nm and 704 nm in the brain. A long-pass filter mounted to the surgical microscope allows tumor visualization as the operator switches between white and violet light. The use of 5-ALA as an adjuvant to glioblastoma surgery improves extent of resection. A phase III clinical trial of patients with suspected glioblastoma receiving surgery using either conventional white light microsurgery or fluorescence-guided surgery assisted with 5-ALA found that 65% of patients treated with 5-ALA received a gross total resection compared with 36% of patients treated with conventional white light microsurgery. Study patients therefore experienced a 50% improvement in 6-month progression-free survival (41.0% vs 21.1%). Complete removal of all fluorescent tissue (also known as a 5-ALA complete resection) results in improved overall survival without an increase in postoperative neurologic deficits.
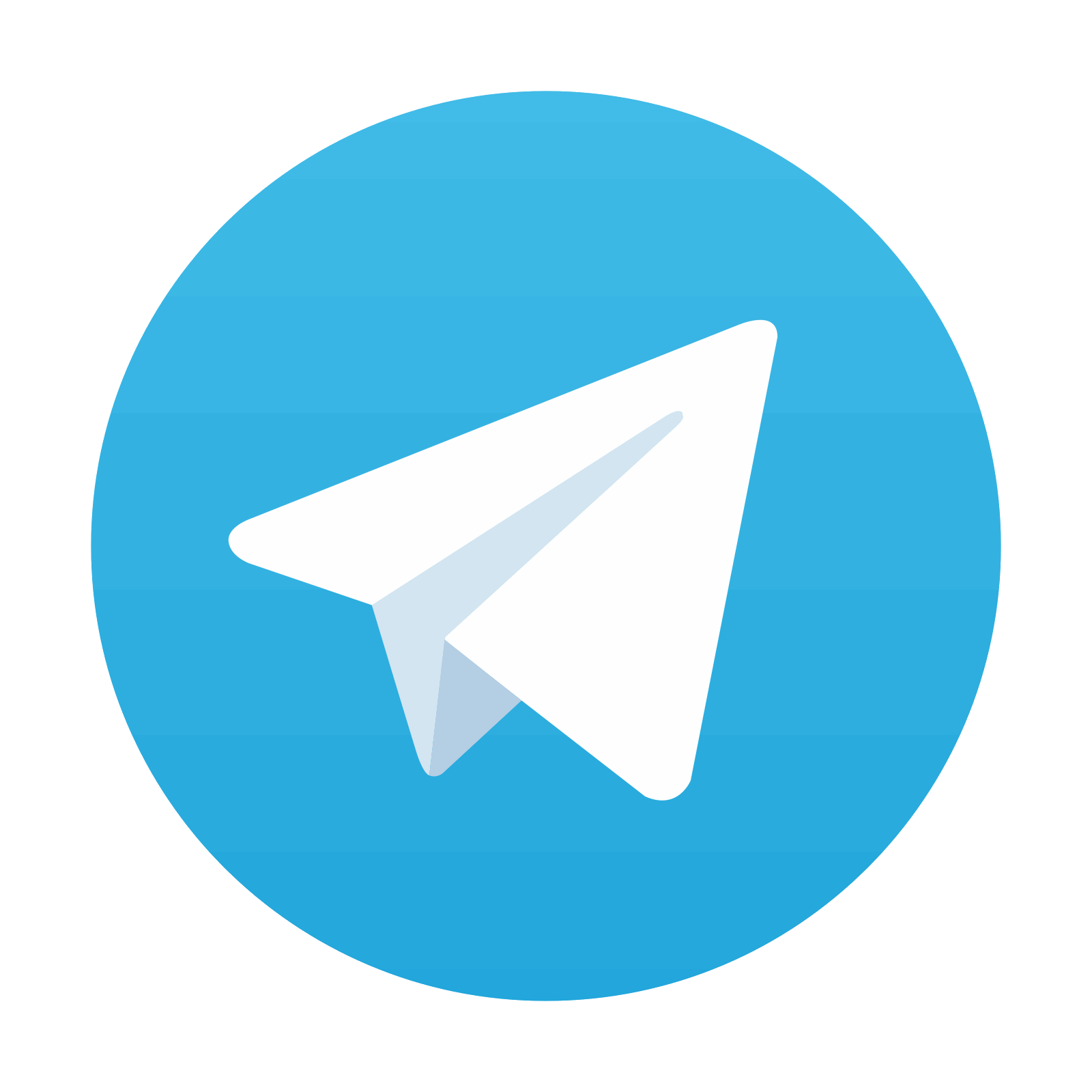
Stay updated, free articles. Join our Telegram channel

Full access? Get Clinical Tree
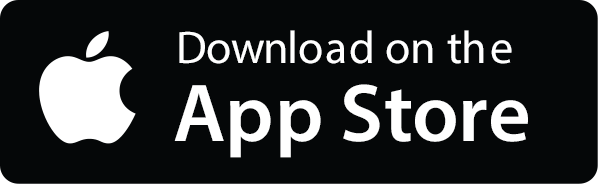
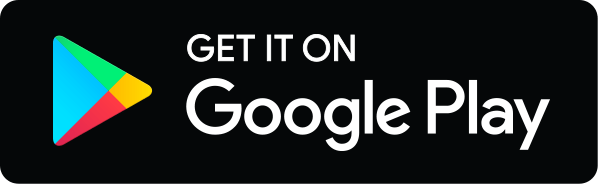