Biomarker definition
Biomarkers are critical to understand disease pathophysiology, improve diagnostics, and select therapies. Biomarkers are used to determine the presence or absence of a disease, measure response to therapy, or predict likely outcomes. Biomarkers may be useful for patient stratification and to determine target engagement in drug development. Key features of a biomarker include sensitivity (ability to detect true positives), specificity (ability to detect true negatives), and predictive ability (measured by areas under receiver-operating characteristic curves), along with precision, accuracy, stability, and reproducibility. The US Food and Drug Administration (FDA) describes a biomarker as “a defined characteristic that is measured as an indicator of normal biological processes, pathogenic processes, or responses to an exposure or intervention, including therapeutic interventions.” , The FDA delineates four primary biomarker categories: diagnostic, prognostic, predictive, and pharmacodynamic ( Fig. 7.1 ).

Ideal biomarkers are safe, easily accessed, and inexpensive. Although frequently associated with blood measures, they are not limited to biofluids and can include a wide variety of traumatic brain injury (TBI) diagnostics, including imaging (e.g., computed tomography [CT], magnetic resonance imaging[MRI]), neurophysiology (e.g., electroencephalography [EEG], magnetoencephalography [MEG]), cerebrospinal fluid (CSF), saliva, urine, blood, and blood components (e.g., DNA, microRNA [miRNA], exosomal cargo). TBI biomarkers remain largely in the research domain because of the inherent complexities of heterogeneous TBI mechanisms (e.g., blunt, shear, blast) affecting the various central nervous system (CNS) components (e.g., neurons, axons, vascular, astroglial cells) and the protean physiological responses to the acute (primary and secondary cascades) injury, making single biomarker tests at a single time point unlikely. Until recently, no TBI biomarker had been approved by the FDA. This chapter focuses on proteomic, genetic, and epigenetic TBI biomarkers.
Proteomics in acute traumatic brain injury
The majority of TBI biomarker research has focused on proteomics (initially in CSF, then in blood) as diagnostic biomarkers of acute TBI within the first 24 hours after injury. For peripheral detection, either the blood-brain barrier (BBB) has to be compromised with egress of CNS proteins from the breached CNS space or active transport (e.g., cargo proteins within exosomes) by lipophilic components readily exit from the CNS. Candidate acute TBI biomarkers include markers of BBB integrity, neuroinflammation, axonal, , neuronal, astroglial, , , and vascular injury.
The first combination TBI biomarkers to receive FDA approval in acute TBI are plasma glial fibrillary acidic protein (GFAP) and ubiquitin C-terminal hydrolase-L1 (UCH-L1), with the Banyon Brain Trauma Indicator (BTI). , , GFAP is an astroglial structural protein that is released with injury; in acute TBI, serum GFAP levels peak 20 hours after injury. UCH-L1, making up 2% of total soluble brain proteins, is a neuron-specific enzyme involved in ubiquitin turnover. It is detectable as early as 1 hour after TBI and peaks earlier than GFAP at 8 hours and then declines slowly over 48 hours after injury.
In the ALERT-TBI trial with 2081 mild and moderate TBI subjects, GFAP and UCH-L1 levels together reliably differentiated TBI with and without CT-detectable intracranial lesions (sensitivity of 97.5% and specificity of 99.6%), and it is currently FDA approved for point-of-care (POC) testing in serum collected within 12 hours of a TBI. , The test is not approved for the diagnosis of TBI; rather its indication is to identify TBI patients who may have an intracranial lesion that could require surgical intervention. S100B, an abundant astroglial protein, also has a high sensitivity for TBI, with higher levels associated with greater TBI severity and poorer outcomes. Despite widespread use in Europe as a TBI biomarker, its lower specificity in patients with polytrauma caused by extracranial sources and varying levels in young children limits its potential as an acute POC biomarker.
Neurofilament proteins are the major intermediate structural proteins of neuronal axons. Individual intermediate filaments are made up of two of three isoforms—neurofilament light (NF-L), medium (NF-M), and heavy (NF-H) chains—and are associated with large-caliber myelinated axons. Likewise tau is an axonal skeletal protein but is associated with thin, unmyelinated axons. Both (CSF , and blood ) are associated with acute axonal injury, with tau demonstrating less CNS specificity than NF-L in polytrauma cases, likely because of peripheral sources of tau. To date, most studies of NF-L and tau have focused on CSF sources as a diagnostic marker of acute TBI, with CSF NF-L levels the most sensitive of acute axonal injury, including in mild TBI (mTBI) among boxers. Recent studies of plasma tau in acute sports concussion collected within the first 6 hours after injury suggest that higher levels may be prognostic biomarkers of prolonged recovery. Amyloid species—including amyloid precursor protein (APP), amyloid beta 40 (Aβ 40 ), and amyloid beta 42 (Aβ 42 )—are associated with neuronal axons and accumulate as early as 2 to 3 hours after TBI with axonal injury. CSF levels of both Aβ 40 and Aβ 42 are increased in acute, severe TBI but not acute mTBI, making it less useful as a diagnostic biomarker of axonal injury.
Biomarkers of BBB disruption, neuroinflammation, and vascular injury are candidate biomarkers that may aid our understanding of TBI pathophysiology. Studies in acute severe TBI have shown BBB disruption with increased CSF to serum albumin ratios and neuroinflammatory responses (interleukin [IL]-1β, IL-6, IL-8, IL-10, TNF alpha) but no similar changes in studies of boxers with mTBI or military personnel with blast exposures, suggesting these are less sensitive as diagnostic TBI biomarkers. Candidate vascular biomarkers include vascular endothelial growth factor, von Willebrand factor, and angiopoietin-1. TBI biomarker discovery projects hypothesize that candidate biomarker analyses from large TBI longitudinal studies that encompass multiple mechanisms and time since injury, partnered with robust data sets and statistical modeling, will advance the field to actuate TBI diagnostic biomarker panels from POC to chronic TBI outcomes.
Proteomics in chronic traumatic brain injury
Chronic TBI biomarker research focuses on advancing our understanding of the mechanisms responsible for ongoing symptoms and deficits. Clinically, one cannot identify the 10% to 15% of mTBI patients who will remain symptomatic 1 year postinjury or optimal timing for a safe return to play after sports concussions. Candidate prognostic biomarkers of TBI early outcomes include S100B, tau and phosphorylated tau (p-tau), NF-L, αII-spectrin breakdown product (SNTF), and neuroinflammatory markers (IL-6, IL-8, IL-10 and TNF-α). The most promising and sensitive to date are plasma measures of tau, NF-L, and GFAP.
There are few studies of TBI biomarkers in the chronic stage, 6 months after injury. The first studied active duty service members who sustained deployment TBIs and were tested 12 or more months later. They had elevated levels of plasma tau in those with repetitive (≥3) TBI, and tau elevations correlated with increased postconcussive symptoms. , Rubenstein reported elevated plasma tau, p-tau, and p-tau:tau ratio in a small cohort of severe TBI patients 6 to 8 months after injury. Exosomes, extracellular vesicles thought to be important in cell-to-cell signaling, have a lipid membrane that easily crosses the BBB and are abundant in the peripheral circulation. Their cargo reflects cytoplasmic metabolic status from the cell of origin and is enriched with peptides, enzymes, and RNA (including miRNA). Recent prognostic biomarker studies of chronic TBI have focused on exosomal proteins of central origin. Several studies have reported elevated levels of exosomal tau, p-tau, NF-L, GFAP, and IL-6 years to decades after TBI, with biomarker levels corresponding to postconcussive and affective symptoms and cognitive impairment. ,
Genetics of traumatic brain injury
Evidence is mounting that genetic variation may modulate response to TBI, including TBI recovery and outcomes. The polymorphism with the most support is the ε4 allele of the apolipoprotein E ( APOE ) gene, which encodes for lipoproteins that transport cholesterol in the peripheral circulation. A metaanalysis suggests that the ε4 allele is associated with poorer outcomes after TBI, including a 10-fold risk of dementia and greater cognitive decline 30 years after TBI. Further, boxers with the ε4 allele who had participated in many bouts were more likely to develop chronic traumatic encephalopathy (CTE) than those who had only boxed a few fights. Several other common genetic variants have been associated with chronic effects of neurotrauma (e.g., DRD2, COMT, BDNF, DAT1, MAO-A ), but their association with neurodegenerative disorders after TBI remains inconclusive.
Recent advances in large-scale genome-wide association studies have helped characterize genetic vulnerability as prognostic biomarkers for a wide range of chronic neurological disorders. Polygenic risk scores (PRS) derived from TBI-related outcomes (e.g., dementia, CTE, posttraumatic headache) could pave the way for a more robust understanding of how genes moderate TBI recovery and may in the future be useful to help predict treatment response.
Epigenetics of traumatic brain injury
Epigenetics refers to factors both inheritable and noninheritable that modify gene activity by a variety of mechanisms that include DNA hyper- and hypomethylation, histone modification (e.g., acetylation), and miRNA regulation of protein translation. , DNA methylation modulates gene expression through the inhibition or reduction of transcriptional proteins binding to DNA. In TBI, DNA methylation has the potential to affect variability in injury susceptibility, intensity of the acute pathophysiological response to injury, rate and extent of recovery, and the emergence of posttraumatic neurodegeneration. Recent studies have investigated its potential to influence outcomes of chronic psychological and related neurologic disorders, including dementia, TBI, and posttraumatic stress disorder. This is a rapidly advancing field in biomarker research, particularly in chronic TBI outcomes.
miRNAs are short lengths of single-stranded RNA (17–25 nucleotides) and are thought to be important in regulating posttranscriptional gene expression . They can be found in several peripheral sources, such as blood, saliva, and urine, with the majority contained within exosomes. There is intense interest in the potential of miRNA expression as a possible acute, chronic, and/or remote TBI diagnostic and prognostic biomarker. There are increasing reports of miRNAs as novel biomarkers for the diagnosis and prognosis of sports concussion and mild , and severe TBI. , Both blood and salivary miRNAs are easily measured, physiologically relevant, and candidate diagnostic and prognostic TBI biomarkers, but these remain a research endeavor at this time.
Review questions
- 1.
Current evidence suggests an acute traumatic brain injury (TBI) diagnostic biomarker panel could include which of the following trios:
- a.
Apolipoprotein E (APOE) genotyping, diffuse tensor imaging (DTI) sequence on magnetic resonance imaging (MRI), plasma tau
- b.
Computed tomography (CT) scan findings, salivary microRNA (miRNA) expression, urinary catecholamine levels
- c.
Cerebrospinal fluid (CSF) neurofilament light (N-FL) level, serum cholesterol level, plasma ubiquitin C-terminal hydrolase-L1 (UCHL-1) level
- d.
Plasma glial fibrillary acidic protein (GFAP) level, exosomal interleukin (IL)-6 level, susceptibility weighted imaging (SWI) sequence on MRI
- a.
- 2.
Recently, a, the US Food and Drug Administration (FDA) approved a TBI serum test for GFAP and ubiquitin C-terminal hydrolase-L1 with a sensitivity of 97.5% for detecting a patient with evidence of TBI on head CT. Given this information, which of the following is true?
- a.
A positive test means the patient has a 97.5% chance having evidence of injury on head CT.
- b.
A negative test result means that the patient has a 2.5% chance of having no evidence of injury on head CT.
- c.
A TBI patient with evidence of injury on head CT has a 2.5% chance of testing negative.
- d.
A TBI patient with no evidence of injury on head CT has a 2.5% chance of testing positive.
- a.
- 3.
GFAP may be a superior biomarker for TBI than S100B because of which of the following?
- a.
S100B is less stable than GFAP.
- b.
S100B has lower specificity than GFAP.
- c.
S100B does not cross the blood-brain barrier after TBI.
- d.
S100B is not expressed in younger children.
- a.
Answers on page 386.
Access the full list of questions and answers online.
Available on ExpertConsult.com
- 4.
Recent data suggest that the biomarkers tau and NF-L isolated from _____ are useful to detect TBI and correlate with prognosis?
- a.
Serum
- b.
CSF
- c.
urine
- d.
whole blood
- a.
- 5.
Serum tau is a useful chronic TBI biomarker because
- a.
elevations in serum tau predict findings on head CT.
- b.
serum tau correlates with the APO-ε4 genotype.
- c.
increased serum tau in chronic TBI patients correlates with postconcussive symptoms.
- d.
serum tau levels can differentiate chronic TBI patients from patients with dementia.
- a.
- 6.
Which of the following is false regarding exosomes?
- a.
Exosomal phosphorylated tau correlates with postconcussive symptoms in chronic TBI patients.
- b.
Exosomes are nanovesicles derived from cell and membrane released by all cells.
- c.
Exosomes are the primary source of miRNA in the blood.
- d.
Exosomes contain chromosomes that are useful for identifying genetic biomarker information.
- a.
- 7.
An example of a candidate TBI prognostic biomarker is
- a.
the presence of the APOE-ε4 allele because it is associated with worse cognitive outcomes after TBI.
- b.
the presence of the APOE-ε4 allele because it can be used to predict how an individual will respond to TBI therapies.
- c.
the presence of the APOE-ε4 allele because it can measure the direct effect of a therapeutic action on TBI outcomes.
- d.
the presence of the APOE-ε4 allele because it can help establish the TBI diagnosis.
- a.
- 8.
Potential epigenetic biomarkers include all of the following except
- a.
DNA methylation.
- b.
RNA methylation.
- c.
DNA mutation.
- d.
histone acetylation.
- a.
References
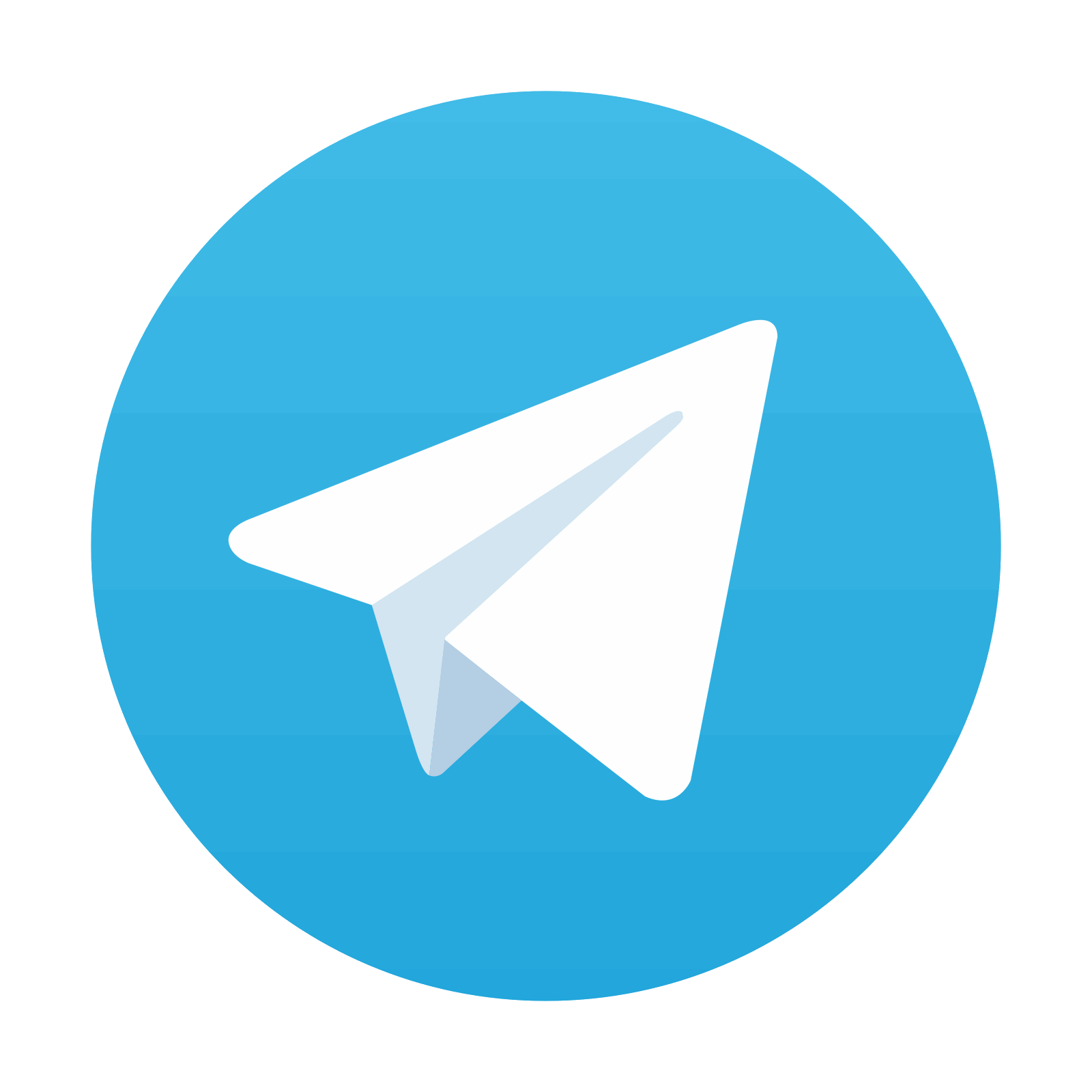
Stay updated, free articles. Join our Telegram channel

Full access? Get Clinical Tree
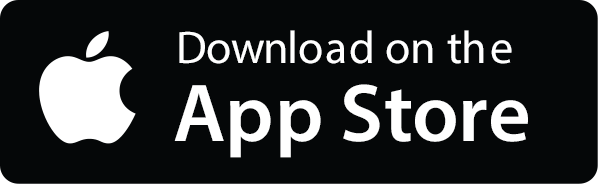
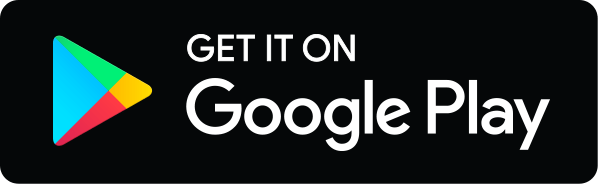
