Radiotherapy has become a part of the standard treatment of high-grade gliomas. Studies have shown that high-dose radiation results in more effective tumor control but at the cost of radionecrosis and other radiation-related side effects. Despite advancing techniques in stereotaxy and precise radiotherapy delivery techniques, studies published for stereotactic radiosurgical treatment of high-grade gliomas have not been unanimous, with large trials showing no survival benefit compared with conventional conformal radiotherapy. New imaging modalities have been studied with the hope to improve accuracy in the planning of radiosurgical treatments. However, further large scale studies are needed to confirm these results.
High-grade gliomas (HGGs) include World Health Organization (WHO) grade 3 anaplastic astrocytoma and grade 4 glioblastoma multiforme (GBM). Although HGG rarely results in distant metastasis, the condition’s seemingly relentless local microproliferation renders its cure impossible (at least in the current technology). Even with the latest imaging and surgical technologies, the exact demarcation of the tumor and its proliferation cannot be determined. This makes the localization of the target an unachievable task. Another unique nature of brain tumor is that the brain is an unforgiving organ that contains many vital structures that many a time HGG involves. The outcome for HGG remains grim despite advancing multimodality treatments, including surgery, chemotherapy, and radiotherapy.
The exact mechanism of radiotherapy is still uncertain. However, the majority supports the notion that double-stranded breaks of the nuclear DNA are the most important cellular effect of radiation. This breakage causes an irreversible loss of reproductive integrity of the cell and eventual cell death. Radiotherapy also uses ionizing radiation to interact with water molecules within the cell, which releases free radicals, whereby causing additional DNA damage.
Soon after the discovery of x-rays by Roentgen in 1895, there were reports that patients with cancers were being successfully treated with radiotherapy.
Frankel and German published one of the earliest reports on radiotherapy for glioblastoma in 1958. The investigators reviewed 219 cases of GBM. Forty-seven patients received radiation doses varying between 2700 and 5900 rads (cGy), and 21 of these patients completed radiotherapy within 60 days after operation. When compared with 62 patients who underwent surgery alone and were alive 60 days after operation, the investigators found that there was a significantly greater percentage of survivors in the irradiated group during the first 12 months. This difference disappeared after the first year. The investigators concluded that routine postoperative radiation effectively prolonged the palliative effects of surgery and proposed a more general usage of radiotherapy. In terms of surgery, they found that a more radical removal offered the best prognosis with regard to operative mortality and survival time.
Radiotherapy is now routinely used as part of the treatment regimen for HGG. Its efficacy and accuracy is continuing to be studied. Problems such as target accuracy and treatment-related complications remain the major evaluation issues. However, with advancing imaging and treatment delivery methods, conclusions and treatment protocols are improving safety and efficacy.
This article reviews the history as well as the recent advances in radiation treatments of HGG.
Definition of the extent of radiation
Ionizing radiations are electromagnetic species that are capable of producing ions as they pass through matter. Photon, out of many types of radiation, is most commonly used for patient treatment. Photons may come in the form of x-rays or gamma rays. These rays are widely available in the hospital setting, produced by affordable linear accelerators (LINACs) or cobalt units. Ionizing heavy particles are also used for radiotherapy. They are generated by larger and expensive cyclotrons and are therefore less available than photons in health care. The most commonly used heavy particle is the proton; however, there is also a large experience with alpha particles generated from helium. More recently, the interest on carbon-generated beam is increasing. The advantage of these expensive particle beams is that they can be sharply stopped as they cross the tumor, therefore depositing the maximum ionizing radiation energy in the tumor itself, without exit dose to normal tissue, as occurring with photons.
Establishment of radiation as part of the multimodality treatment of HGG s
Although radiation therapy has been used in treating primary brain tumors since the early 1900s, there was no scientific evidence that it was efficacious and safe. In 1979, Walker and colleagues published their report on the analysis of dose-effect relationship for malignant gliomas based on the experience of the Brain Tumor Study Group. They compared the median survival in patients who did not undergo radiotherapy (18 weeks) with that in those who were irradiated using radiation doses of 45 Gy or less (13.5 weeks), 50 Gy (28 weeks), and 60 Gy (42 weeks). The investigators found an increase of 1.3 times in median life span associated with the higher dose between 5000- and 6000-rads (cGy) groups. They concluded that radiotherapy had a significant influence on the survival of patients with malignant glioma, and a clear-cut dose-effect relationship was found. At around the same time, the Scandinavian Glioblastoma Study Group also published the results of a multicenter randomized trial on adjuvant irradiation for operated HGGs, disclosing that 45-Gy whole-brain irradiation increased median survival from 5.2 to 10.0 months. To investigate the relationship between radiation dosage and survival, Salazar and colleagues compared groups of postoperative patients with GBM who were given radiation doses of 50, 60, and 75 Gy. The investigators found patients’ survival to be 30, 42, and 56 weeks among the groups given 50, 60, and 75 Gy, respectively. The increase in median survival was only significant between the extremes and not between the intermediate dose, leading to the conclusion that higher radiation doses (75 Gy) did not significantly alter overall survival and could increase risk of radiation necrosis. Still in the 1990s, a randomized trial by the Medical Research Council also demonstrated an improvement in median survival from 9 to12 months when 60 Gy was compared with 45 Gy. Both this and the Brain Tumor Cooperative Group trials led to the conclusion that 60 Gy is the ideal dose for adjuvant postoperative radiotherapy for HGGs.
In a systematic review of radiotherapy for newly diagnosed malignant glioma, 6 randomized trials detected a significant survival benefit favoring postoperative radiotherapy compared with no radiotherapy. Another randomized trial detected a small improvement in survival with 60 Gy in 30 fractions over 45 Gy in 20 fractions. It was concluded that postoperative external beam radiotherapy (EBRT) is recommended as standard therapy for patients with malignant glioma. The high dose volume should incorporate the enhancing tumor plus a limited margin (eg, 2 cm) for planning target volume (PTV), and the total dose delivered should be in the range of 50 to 60 Gy in fraction sizes of 1.8 to 2.0 Gy.
In 2005, a joint European Organization for Research and Treatment of Cancer (EORTC) and National Cancer Institute of Canada (NCIC) randomized trial found that concomitant and adjuvant temozolomide significantly increased the median survival of patients with GBM from 12.1 to 14.6 months and more than doubled the 2-year survival (26.5% vs 10.4%). The current standard treatment after resection or biopsy of GBM is fractionated focal radiotherapy (60 Gy, 30–33 fractions of 1.8–2 Gy, or equivalent doses per fractionations) with concomitant chemotherapy with temozolomide (dose of 75 mg/m 2 ) daily (7 days per week) followed by 5-day cycles every 4 weeks to complete. The result of the 5-year analysis of this trial was published in 2009. The investigators found that benefits of adjuvant temozolomide with radiotherapy lasted throughout 5 years of follow-up in all clinical prognostic subgroups, including patients aged 60 to 70 years.
Other chemotherapeutic agents have also been tried along with radiation for the treatment of HGGs. In a retrospective study examining the effect of reirradiation with bevacizumab for recurrent HGGs, patients who were previously treated with standard radiotherapy received bevacizumab (10 mg/kg intravenous) on day 1 and day 15 during administration of 36 Gy in 18 fractions. It was noted that the overall survival was significantly better in patients receiving bevacizumab than in those receiving no additional substance or temozolomide. The conclusion was that reirradiation with bevacizumab is feasible and effective for recurrent HGGs.
Establishment of radiation as part of the multimodality treatment of HGG s
Although radiation therapy has been used in treating primary brain tumors since the early 1900s, there was no scientific evidence that it was efficacious and safe. In 1979, Walker and colleagues published their report on the analysis of dose-effect relationship for malignant gliomas based on the experience of the Brain Tumor Study Group. They compared the median survival in patients who did not undergo radiotherapy (18 weeks) with that in those who were irradiated using radiation doses of 45 Gy or less (13.5 weeks), 50 Gy (28 weeks), and 60 Gy (42 weeks). The investigators found an increase of 1.3 times in median life span associated with the higher dose between 5000- and 6000-rads (cGy) groups. They concluded that radiotherapy had a significant influence on the survival of patients with malignant glioma, and a clear-cut dose-effect relationship was found. At around the same time, the Scandinavian Glioblastoma Study Group also published the results of a multicenter randomized trial on adjuvant irradiation for operated HGGs, disclosing that 45-Gy whole-brain irradiation increased median survival from 5.2 to 10.0 months. To investigate the relationship between radiation dosage and survival, Salazar and colleagues compared groups of postoperative patients with GBM who were given radiation doses of 50, 60, and 75 Gy. The investigators found patients’ survival to be 30, 42, and 56 weeks among the groups given 50, 60, and 75 Gy, respectively. The increase in median survival was only significant between the extremes and not between the intermediate dose, leading to the conclusion that higher radiation doses (75 Gy) did not significantly alter overall survival and could increase risk of radiation necrosis. Still in the 1990s, a randomized trial by the Medical Research Council also demonstrated an improvement in median survival from 9 to12 months when 60 Gy was compared with 45 Gy. Both this and the Brain Tumor Cooperative Group trials led to the conclusion that 60 Gy is the ideal dose for adjuvant postoperative radiotherapy for HGGs.
In a systematic review of radiotherapy for newly diagnosed malignant glioma, 6 randomized trials detected a significant survival benefit favoring postoperative radiotherapy compared with no radiotherapy. Another randomized trial detected a small improvement in survival with 60 Gy in 30 fractions over 45 Gy in 20 fractions. It was concluded that postoperative external beam radiotherapy (EBRT) is recommended as standard therapy for patients with malignant glioma. The high dose volume should incorporate the enhancing tumor plus a limited margin (eg, 2 cm) for planning target volume (PTV), and the total dose delivered should be in the range of 50 to 60 Gy in fraction sizes of 1.8 to 2.0 Gy.
In 2005, a joint European Organization for Research and Treatment of Cancer (EORTC) and National Cancer Institute of Canada (NCIC) randomized trial found that concomitant and adjuvant temozolomide significantly increased the median survival of patients with GBM from 12.1 to 14.6 months and more than doubled the 2-year survival (26.5% vs 10.4%). The current standard treatment after resection or biopsy of GBM is fractionated focal radiotherapy (60 Gy, 30–33 fractions of 1.8–2 Gy, or equivalent doses per fractionations) with concomitant chemotherapy with temozolomide (dose of 75 mg/m 2 ) daily (7 days per week) followed by 5-day cycles every 4 weeks to complete. The result of the 5-year analysis of this trial was published in 2009. The investigators found that benefits of adjuvant temozolomide with radiotherapy lasted throughout 5 years of follow-up in all clinical prognostic subgroups, including patients aged 60 to 70 years.
Other chemotherapeutic agents have also been tried along with radiation for the treatment of HGGs. In a retrospective study examining the effect of reirradiation with bevacizumab for recurrent HGGs, patients who were previously treated with standard radiotherapy received bevacizumab (10 mg/kg intravenous) on day 1 and day 15 during administration of 36 Gy in 18 fractions. It was noted that the overall survival was significantly better in patients receiving bevacizumab than in those receiving no additional substance or temozolomide. The conclusion was that reirradiation with bevacizumab is feasible and effective for recurrent HGGs.
Importance of surgery for radiation treatment
Surgery is usually performed for either tissue diagnosis or tumor debulking purposes. In a review of the literature of both HGGs and low-grade gliomas, Sanai and Berger showed that more extensive tumor resection is associated with longer life expectancy. Increasing the extent of resection was also found to be associated with improved survival independent of age, degree of disability and WHO grade, or subsequent treatment modalities used in both primary and repeat resection of malignant gliomas. However, for tumors that are located in eloquent areas of the brain or for patients with poor premorbid status, a biopsy of the tumor may be more appropriate.
Modern techniques of radiation for HGG s based on modern imaging
Gliomas have no capsule and infiltrate the brain, particularly along the white matter tracts diffusely. This makes definition of the tumor extent extremely difficult with the conventional radiologic technology. Without a definite margin, it is virtually impossible to plan a radiation treatment targeting only the tumor and sparing normal tissue. The current practice is to irradiate the tumor-involved tissue based on T2-weighted MRI along with an additional 2-cm margin. This practice is based on studies showing that most tumors recur within a 2-cm margin.
However, modern MRI sequences promise to shed light on this difficult issue. Diffusion tensor imaging (DTI) is a modification of diffusion-weighted imaging that is sensitive to the preferential diffusion of brain water along axonal fibers and hence is useful in demonstrating white matter tract anatomy and can detect subtle changes in diseased white matter tracts ( Fig. 1 ). Thus, many studies have been performed to investigate the usefulness of DTI in demonstrating the extent of HGGs. One of these studies compared DTI with T2-weighted 3-T MRI. The investigators found that subtle white matter disruption is identified using DTI beyond what was seen on T2-weighted images of patients with HGGs. This DTI white matter disruption was not apparent in metastatic lesions or low-grade gliomas. Correlative studies of DTI abnormalities and histologic confirmation have been conducted to determine the accuracy of DTI in detecting tumor infiltration by HGG. One study found a sensitivity of 96% and a specificity of 85%.
In an attempt to put DTI planning to test, Jena and colleagues compared standard planning with individualized planning based on DTI findings. Standard plans were generated using a clinical target volume (CTV) margin of 2.5-cm added to the gross tumor volume (GTV) and were compared with DTI-based plans in which the CTV was generated by adding a 1-cm margin to the tumor-involved margin. The investigators found that DTI could reduce the size of the PTV by a mean of 35% and resulted in an escalated dose (mean, 67 Gy; range, 64–74 Gy), with normal tissue complication probability (NTCP) matching that of conventional treatment plans. Findings from studies that showed most tumors recurred in the high-dose treatment volume rather than at the margin seem to suggest that local tumor recurrence may not be caused by inadequate treatment volume or margin but rather insufficient dose to the central tumor. Thus the investigators conclude that DTI can be used to individualize radiotherapy target volume with reduction of CTV, yielding modest dose escalation without increasing the NTCP.
l -(methyl-11C) methionine–labeled positron emission tomography (MET-PET) has been shown to have higher specificity and sensitivity in tumor delineation than MRI. l -(methyl-11C) methionine (MET) is a natural amino acid avidly taken up by glioma cells, with only a low uptake in normal brain tissue. Comparative analysis between computed tomography (CT), MRI, MET-PET, and stereotactic biopsies has shown that MET-PET has greater accuracy in defining the extent of gliomas than CT and MRI. In a study comparing MET-PET and MRI for GTV definition for radiotherapy planning of HGG, it was found that the size and location of residual MET uptake differ considerably from that found on postoperative MRI. Given the known high accuracy of MET-PET for detection of tumor tissue, these findings suggest that MET-PET may significantly improve the definition of target volumes in patients with HGGs. It was also proposed that using MET-PET/MRI fusion imaging and combining the biological characterization of the tissue with accurate presentation of the anatomy, a more precise delineation of the target volume for radiotherapy planning could be achieved. An important disadvantage of MET includes the low physical half-life of about 20 minutes, requiring an on-site cyclotron.
As further evidence to support the usage of MET-PET in radiotherapy planning for HGG, one study looked at the usage of MET-PET/CT/MRI fusion to determine the GTV for stereotactic fractionated radiotherapy for reirradiation of recurrent HGGs. In this prospective nonrandomized single-institution trial using stereotactic fractionated radiotherapy (SFRT) reirradiation plus temozolomide, MET-PET or iodine I 123 α-methyl tyrosine single-photon computed emission tomography (SPECT)/CT/MRI fusion and CT/T1 plus gadolinium-enhanced MRI integrated radiation treatment plans were compared. Six fractions of 5 Gy were administered in 6 days. Temozolomide, 200 mg/m 2 body surface per day, was administered in 1 to 2 cycles before SFRT and 4 to 5 cycles after SFRT. The median survival time for patients with treatment planning based on PET(SPECT)/CT/MRI was significantly longer than those having radiation planning using anatomic imaging (CT/MRI) alone, 9 months versus 5 months, respectively. It was concluded that biological imaging–optimized SFRT plus temozolomide in recurrent HGGs was feasible and safe and led to a significantly longer survival.
HGGs are generally considered to be radioresistant because of the hypoxic nature of the cells. Molecular oxygen is known to be a powerful modifier of cell sensitivity to radiation. The biological effect of ionizing radiation has been reported to be increased by about 3-fold when irradiation is performed under well-oxygenated conditions. Hyperbaric oxygenation (HBO) is used to assist in the repair of radiation-induced damage, because it is thought that high oxygen tension promotes neovascularization in tissues of irradiated patients. A recent phase 2 trial investigating the long-term results of radiotherapy administered immediately after HBO with multiagent chemotherapy in adults with HGGs was published. Patients with histologically confirmed malignant gliomas were irradiated daily with 2-Gy fractions for 5 consecutive days per week up to a total dose of 60 Gy. Each fraction was administered immediately after HBO. The chemotherapeutic agents used were procarbazine, nimustine, and vincristine; these were given during and after radiotherapy. The median overall survivals in patients with GBM and grade 3 glioma were 17.2 and 113.4 months, respectively. No patient developed neutropenic fever, intracranial hemorrhage, or serious nonhematologic or late toxicities. It was concluded that the use of radiotherapy immediately after HBO with chemotherapy for HGGs was safe, with virtually no late toxicities, and seemed effective.
High linear energy transfer–charged particle therapy with carbon ions has been investigated in the treatment of HGGs because of its better dose localization in the tumor volume and greater biological effectiveness. Because carbon ions have inverted dose profile and high local dose deposition within the Bragg peak, precise dose application and sparing of normal tissue are possible. A phase 1/2 clinical trial for patients with malignant gliomas treated with combined radiotherapy, chemotherapy, and carbon ion radiotherapy was published in 2007. Patients with confirmed HGGs were treated with 50 Gy in 25 fractions for 5 weeks of radiotherapy followed by carbon ion radiotherapy in 8 fractions for 2weeks. Nimustine hydrochloride at a dose of 100 mg/m 2 was given with radiotherapy. Carbon ion dose was increased from 16.8 to 24.8 Gy equivalent in 10% incremental steps. The median survival times for grade 3 gliomas and GBM were 35 months and 17 months, respectively. The median progression-free survival and median survival time showed 4 and 7 months for the low-dose group, 7 and 19 months for middle-dose group, and 14 and 26 months for high-dose group. It was thus concluded that carbon ion radiation seems to be effective in the treatment of HGGs and that dose escalation of carbon ions has a statistical significance in the overall survival and progression-free survival of HGGs. This and other studies have led to a randomized phase 1/2 trial evaluating the effects of carbon ion radiotherapy versus fractionated stereotactic radiotherapy (FSRT) in recurrent or progressive gliomas. In phase 1 of the carbon ion radiotherapy versus fractionated steretotactic radiotherapy in patients with recurrent or progressive gliomas (CINDERELLA) trial, the recommended dose of carbon ion radiotherapy was determined in a dose escalation scheme. In phase 2, the recommended dose was evaluated in the experimental arm compared with the standard arm, FSRT with a total dose of 36 Gy in single doses of 2 Gy. The primary end point of phase 1 was toxicity. For the randomized phase 2, the primary end point was survival after reirradiation at 12 months and the secondary end point was progression-free survival.
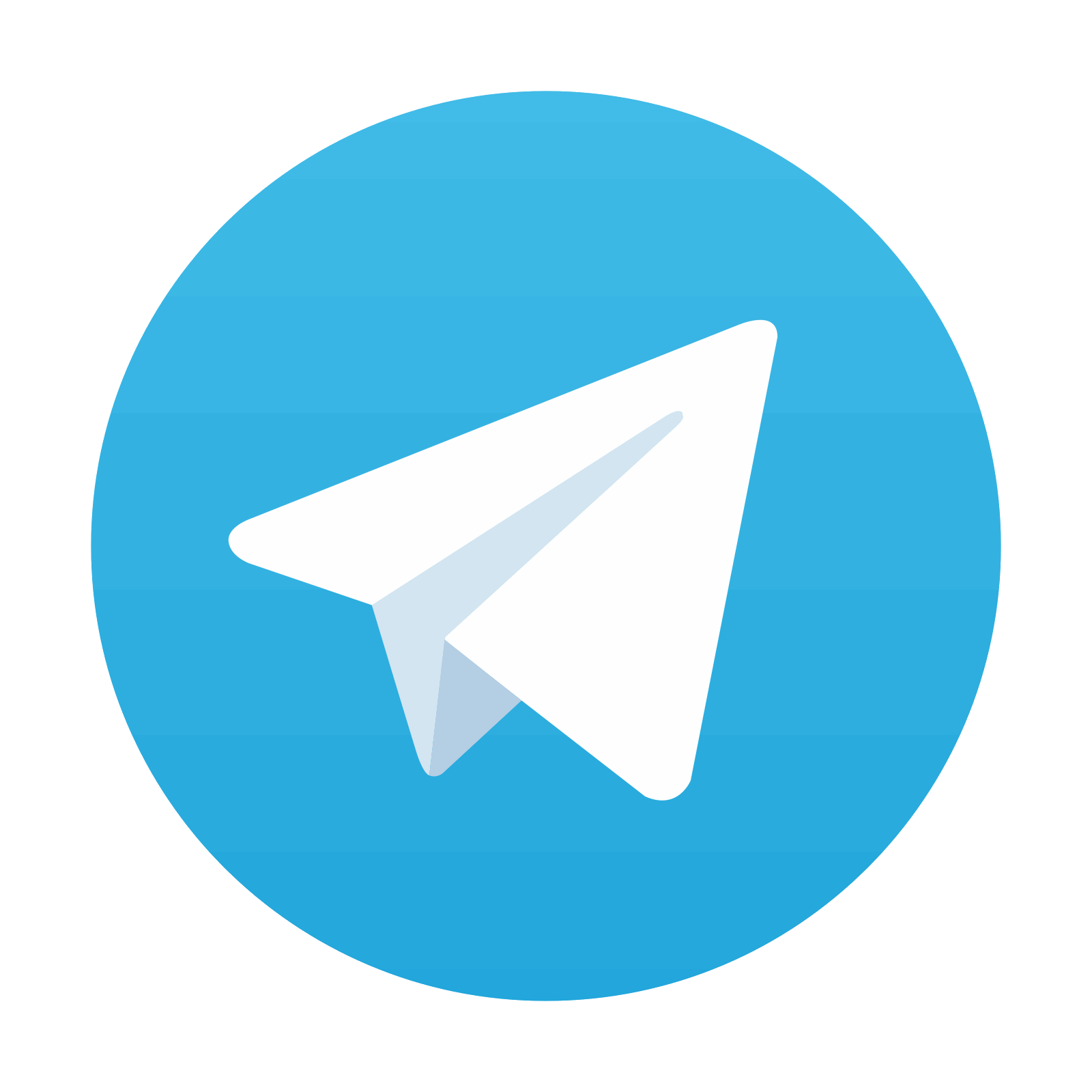
Stay updated, free articles. Join our Telegram channel

Full access? Get Clinical Tree
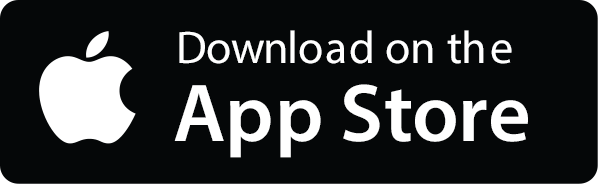
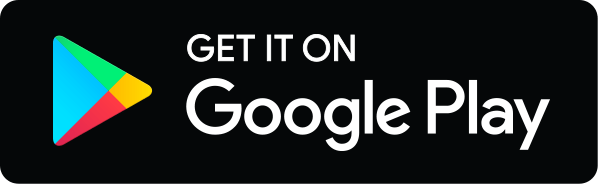