Radiosurgical Management of Arteriovenous Malformations
Pearls
Arteriovenous malformation (AVM) radiosurgery has its greatest role in the management of subcortical AVMs less than 3 cm in maximum diameter.
Dose selection for AVM radiosurgery is mainly based on AVM volume and location, but the margin dose should rarely be below 16 Gy.
The main limitation of AVM radiosurgery is hemorrhage during the latency interval before complete obliteration.
If radiosurgery is performed for AVMs greater than 15 mL in volume, consideration should be given to volume or dose staging of the target.
Successful arteriovenous malformation (AVM) radiosurgery achieves complete AVM nidus obliteration, which leads to elimination of further hemorrhage risk.1 – 3 While this goal is being achieved, there should be limited morbidity and no mortality from hemorrhage or radiation-induced brain injury. When these outcomes can be achieved with a high likelihood, a strong case can be made for radiosurgery. If clinical or angiographic factors argue against the achievement of these goals, then other strategies should be considered instead. Physicians who make an argument for radiosurgery cite one or more of the following factors: (1) radiosurgery is an effective therapy for the management of deep-brain AVMs; (2) radiosurgery is an effective therapy for residual AVMs after subtotal resection; (3) radiosurgery is worthwhile in attempting to lower management risks for AVMs in functional brain locations; (4) because embolization does not cure most AVMs, additional therapy such as radiosurgery may be required; (5) microsurgical resection may not be the best choice for some patients because of their general health; and (6) radiosurgery entails lower costs. Because radiosurgery is the first and only biologic AVM therapy, it represents the basis for future cellular approaches to vascular malformation diseases. For this reason, the future of radiosurgery may be impacted positively by the development of other biologic strategies such as brain protection or endothelial sensitization.
♦ Decision Making
Those who make an argument against the use of radiosurgery cite the following factors: (1) radiosurgery does not always work, especially when only partial AVM obliteration is achieved4; (2) brain hemorrhage may occur during the time it takes for radiosurgery to work; (3) radiation-related morbidity may cause functional neurologic deficits; (4) there may be long-term problems after brain irradiation5; and (5) resection may be a more cost-effective treatment over the long term.6 Although all these factors can be debated, most neurosurgeons agree that the role of radiosurgery is greatest for patients with small-volume, deep-brain AVMs.2 It has a lesser role for patients with larger and surgically accessible AVMs. In between these two extremes there exists much debate. The role of radiosurgery for patients with small but accessible AVMs is growing steadily.7 For patients with largevolume yet deeply located AVMs, multimodality management often is required. Thus, whether radiosurgery should be considered in the management of an individual patient depends on the factors of AVM volume, brain location, prior hemorrhage history, patient age, and surgical resectability. These factors have been studied in detail by different groups as predictors of successful AVM outcomes or as reasons for radiosurgery failure.3 , 8 , 9 The role of AVM obliteration and radiosurgical morbidity in decision making is addressed in further detail later in this chapter.
♦ How Does Radiosurgery Work?
Radiosurgery is effective because single-fraction irradiation causes significant injury to the endothelial cells of blood vessels that compose the AVM.10 – 12 Stereotactic definition of the AVM target ensures that these radiobiologic effects are limited to the malformation. Conformal radiosurgery allows irradiation of only a small volume of surrounding normal tissue in the region of radiation dose fall-off.2 Dose-prescription formulas are used to help select an appropriate radiation dose depending on imaging and clinical factors.13
The immediate effect of radiosurgery is to damage the endothelial cells of the AVM vessels. Release of tissue-specific cytokines common to other forms of radiation-induced injury is likely to mediate such acute effects. Inflammatory cells mediate tissue repair in response to irradiation. Later, chronic inflammation consists of the ingrowth of granulation tissue that contains fibroblasts and new capillaries. These events may explain the delayed imaging changes sometimes observed after radiosurgery. Szeifert et al14 identified the presence of actin-producing fibroblasts, so-called myofibroblasts, that are hypothesized to exert contractile properties and facilitate AVM obliteration. Contrast-enhanced magnetic resonance imaging (MRI) studies at this late stage after obliteration commonly show enhancement of the obliterated AVM. This finding does not indicate a “patent” AVM, but rather we believe it is a marker for the newly formed capillary network within the scarred AVM tissue remnant. Several reports have noted the rare, late finding of cyst formation at the AVM site, which probably represents expansion of the extracellular fluid space within the fibrosis.5 , 15 Radiosurgery may impact on seizure control through irradiation of epileptogenic tissue or through correction of abnormal hemodynamic conditions.16
♦ Results of Stereotactic Radiosurgery
Clinical Experience
At the University of Pittsburgh, 1129 AVM patients had gamma knife radiosurgery during a 20-year period. The mean patient age was 36 years (range 2–82). Prior intracranial hemorrhage was reported in 38% of patients (n = 424), headaches in 38%, and seizures in 28%. The wide variety of clinical presentations ensures discussion of the different treatment options in all patients. All referred vascular malformation cases are discussed at a weekly multidisciplinary conference. Intravascular embolization was performed in 206 patients (18%) before radiosurgery; 127 patients (11%) had already undergone one or more surgical procedures prior to radiosurgery. For some of these patients, the goal of surgery had been AVM resection, whereas for others the goal was hematoma removal. The median AVM volume was 3.4 mL (range 0.03–58 mL). The 50% isodose was used as the margin isodose in 81% of patients. Only 0.7% of patients were treated below the 50% isodose.
The Spetzler-Martin grading system was used to classify all AVMs according to size, critical location, and venous drainage. The most commonly referred patient was one with a small-volume, deeply located AVM (grade III, n = 380, 34%). The smallest category of patients had an AVM that was small, superficial, and noncritical in location (grade I, n = 27, 3.0%). In such patients we first recommend a resection unless the patient has a medical contraindication or refuses that recommendation. Eleven percent of patients (n = 121) had a grade VI AVM. The AVM was located totally within the parenchyma of the brainstem or thalamus. The mean dose delivered to the AVM margin was 18 Gy, and the mean maximum dose 34 Gy. Although there is no such thing as an “inoperable” AVM, we consider an AVM to be associated with an acceptably high risk for resection when it is located completely within the parenchyma of the brainstem, thalamus, or basal ganglia. In our series, 53 patients had brainstem AVMs, 97 had thalamic AVMs, and 68 had basal ganglia malformations.
When radiosurgery did not lead to complete AVM obliteration, we further discussion the merits of repeat radiosurgery or resection. At our center 118 patients underwent repeat radiosurgery for persistent AVM nidus after at least 3 years had elapsed since the first procedure. If, after 3 to 4 years, a residual AVM nidus with early venous drainage remains, then a second radiosurgical procedure should be performed.17 We do not recommend additional management for patients who harbor only an early draining vein, as this feature resolves over an additional observation interval. In addition, we know of no patient who sustained a later hemorrhage when only an early draining vein was present. Some patients’ angiograms show some abnormal-appearing vessels in the region of the irradiated AVM, without early venous drainage. This fine vascular blush may indicate the neocapillary network within the scarred malformation. Such findings also require no additional therapy.
Repeat radiosurgery is associated with a 70% probability of obliteration.4 , 18 At the second procedure, only the small remnant needs to be irradiated, usually at a dose higher than the first dose delivered (especially if the initial AVM was large and the remnant is small, depending on location).
♦ How Arteriovenous Malformation Obliteration Affects Decision Making
Whether an AVM can be successfully obliterated depends on whether proper stereotactic nidus definition can be performed followed by delivery of an adequate radiosurgery dose.19 A complete analysis of 197 AVM patients with up to 3-year angiographic follow-up showed an overall complete obliteration rate of 72% after a single procedure. These results were stratified by volume. In 20 of 197 patients (10%) the targeted AVM nidus failed to obliterate totally. The most important reason for lack of complete obliteration was incomplete targeting.18 An additional 35 patients (18%) had a residual AVM that was not included in the original treatment volume. Many of these patients then underwent a second radiosurgery procedure. Important obliteration factors were identified in this study: incomplete imaging-definition of the AVM, reappearance of AVM after initial compression by hematoma, and recanalization of a previously embolized nidus. We and others advocate the use of multimodality imaging (MRI, magnetic resonance angiography [MRA], and conventional stereotactic angiography) to obtain the best results.20 For the smallest AVM (less than 1.3 mL), 90% of patients had complete obliteration (45 of 50), and 98% had obliteration of the target (49 of 50). For AVMs between 1.4 and 3 mL, 41 of 49 patients had complete obliteration (84%) and 47 of 49 had obliteration of the included target (96%). These data indicate that the radiosurgical dose will achieve our goal with a high likelihood if we can accurately tailor it to the entire lesion margin (Fig. 28.1).
In a separate analysis of our data, we reported a multivariate analysis of AVM obliteration as related to dose and volume.19 A clear dose response up to 25 Gy was identified. We concluded that large AVMs have low obliteration rates because of the combination of lower treatment doses used and the greater problems encountered with target definition. An analysis of 95 patients with thalamic or basal ganglia AVMs found similar obliteration rates when stratified by volume; overall, 80% of patients were cured after a single procedure. Thus, AVM volume not only means that more tissue exists to undergo obliteration, but that there may be additional challenges in stereotactic targeting.
Liscak et al21 reported that AVM obliteration was achieved in 222 (67%) of 330 patients after the initial stereotactic radiosurgery (SRS) and in 47 (69%) of 68 patients who underwent repeat SRS. Final angiography verified complete obliteration by 12 to 96 months (median, 25 months). Smaller volume AVMs and the application of a higher margin dose resulted in a higher chance of obliteration. The risk of rebleeding after SRS was 2.1% annually until full obliteration. The risk of permanent morbidity after the initial and repeat SRS were 2.7% and 2.9%, respectively.21 Subtotal obliteration of AVMs after SRS implies a complete angiographic disappearance of the AVM nidus but persistence of an early filling draining vein, indicating that residual shunting is still present; hence, per definition there is still a patent AVM, and the risk of bleeding is not eliminated. Yen et al22 reported that there was no case of bleeding after the diagnosis of subtotally obliterated 159 AVMs (16 of 159 subtotally obliterated AVM patients underwent repeat SRS) at mean follow-up of 3.9 years after diagnosis of a subtotally obliterated AVM. The fact that none of the patients with subtotally obliterated AVMs suffered a rupture is not compatible with the assumption of an unchanged risk of hemorrhage for these lesions, and implies that the protection from rebleeding in patients with subtotal obliteration may be significant. Subtotal obliteration does not seem to be a stage of an ongoing obliteration. At least in some cases it represents an end point of this process, with no subsequent obliteration occurring. This observation requires further confirmation by open-ended follow-up imaging.
The optimal timing of gamma knife surgery (GKS) after AVM hemorrhage depends on identification of the target tissue. If a hematoma obscures identification of the nidus, then several months should elapse for blood to resorb. Maruyama et al23 retrospectively studied 211 patients with AVMs who presented with hemorrhage and underwent GKS as the initial treatment. Patients were categorized into three groups according to the interval between the time of first hemorrhage and GKS, as follows: group 1, 0 to 3 months (70 patients); group 2, >3 to 6 months (62 patients); and group 3, >6 months (79 patients). After a median follow-up of 6.3 years, the rates of obliteration, hemorrhage after treatment, and complication were not significantly different between the three groups even though the patients with a longer interval before GKS (group 3) had more AVMs in eloquent areas and neurologic deficits. However, the numbers of patients with preoperative hemorrhage in the interval before GKS was significantly higher in group 3 (1, 3, and 20 patients in groups 1, 2, and 3, respectively). These results were similar in the analyses of 127 patients presenting with intracerebral hemorrhage (ICH). The authors concluded that no benefit was detected in waiting for hematoma absorption before performing GKS after a hemorrhage from an AVM. Because of higher hemorrhagic risk from performing GKS more than 6 months after hemorrhage, the authors recommend performing GKS within 6 months after hemorrhage ( Fig. 28.2 ).23
Pikus et al24 argued that the high rate of complete microsurgical resection in their 72-patient AVM series (99%) with an 8% rate of new permanent neurologic deficits, supported their belief that resection was better than radiosurgery for small AVMs. However, only three of their patients (4%) had AVMs in the basal ganglia, thalamus, or brainstem. Porter et al6 constructed a decision analysis model based on obliteration estimates and morbidity rates for resection and radiosurgery. They concluded that resection conferred a clinical benefit because of early protection from hemorrhage. Radiosurgery became a superior treatment if the surgical morbidity rate exceeded 12%. They did not factor the use of second stage or repeat radiosurgery into their model, choosing to leave patients with subtotally obliterated AVMs unprotected for the rest of their expected life. This outcome is rare because most patients achieve complete obliteration but may require more than one procedure. Thus, how obliteration data are used and the brain locations from which they are obtained are important factors in deciding when to use the different techniques.
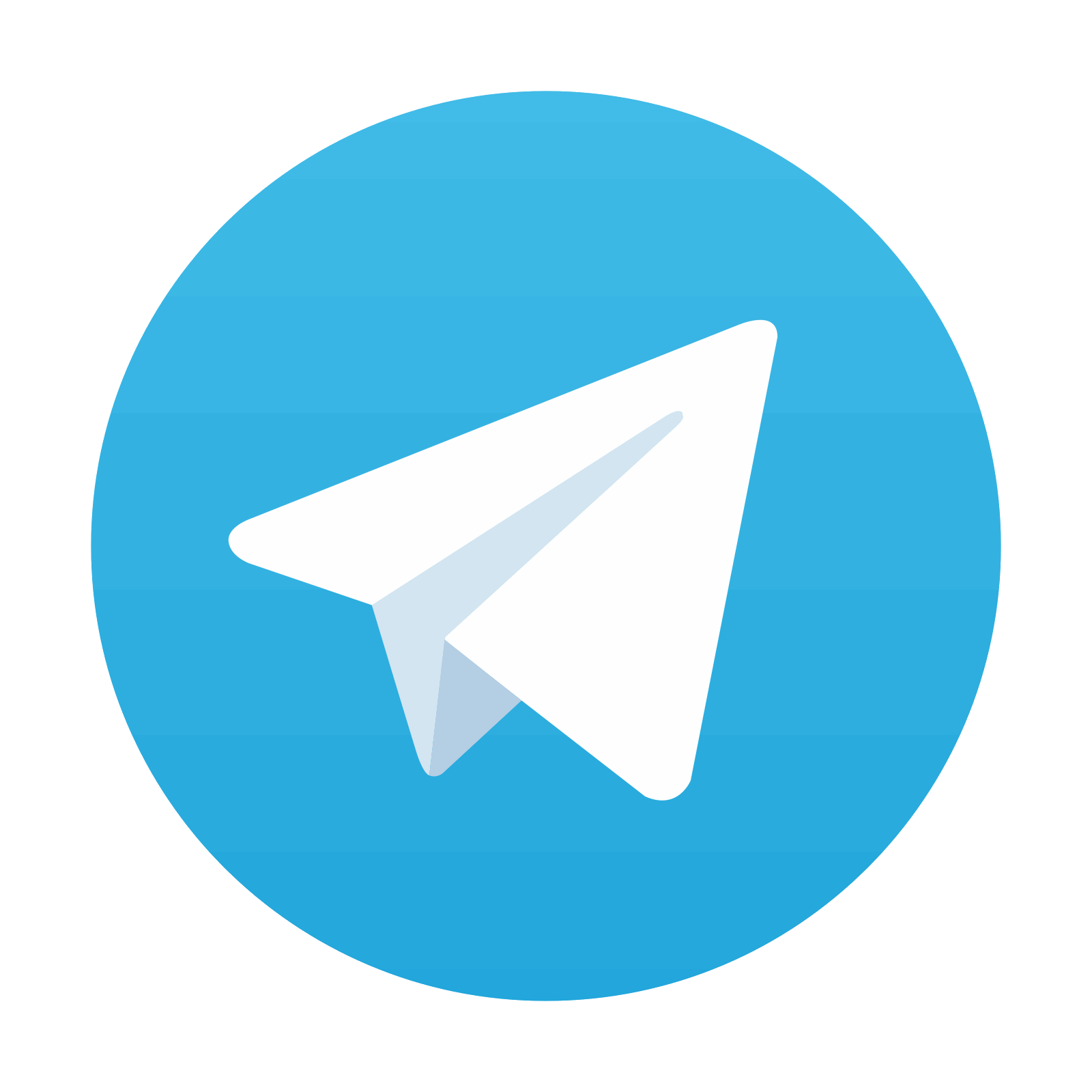
Stay updated, free articles. Join our Telegram channel

Full access? Get Clinical Tree
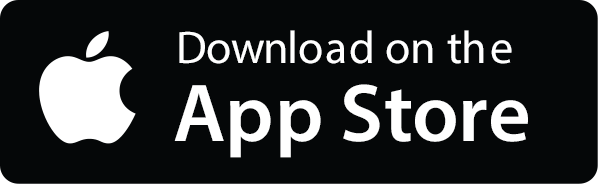
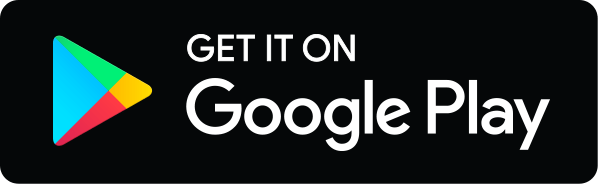