Radiosurgical Management of Lesions of the Craniovertebral Junction
Tumors of the craniovertebral junction (CVJ) pose a challenge for the clinician because their anatomical location makes them difficult to access. Treatment, both surgical and nonsurgical, can be associated with significant morbidity from injury to surrounding neurovascular structures as well as from biomechanical instability at the CVJ.1,2 The main goals of treatment of cranial base tumors of the CVJ are relief of neurological symptoms, eradication of tumor progression and recurrence, and preservation of structural stability.2 Ideally, these objectives should be achieved with the least degree of morbidity allowed by the treatment modality, which can be difficult for pathology in this area given the local anatomy. Stereotactic radiosurgery (SRS) has become a popular treatment method for numerous neurological conditions, both as adjuvant and primary therapy. For many lesions of the CVJ, SRS offers a means of safe treatment for local control of skull base lesions that are not easily surgically curable.
Numerous radiosurgical delivery systems are available, including cobalt-60 (i.e., Gamma Knife) and linear accelerator (LINAC) based systems. Some systems typically require frame placement and are primarily used for single fraction radiosurgery, whereas others can be used in a frameless fashion to deliver up to five fractions of SRS or for additional fractions in intensity modulated radiation therapy (IMRT) mode. For some radioresistant tumors, proton beam therapy is an emerging treatment modality. This chapter reviews the experience, safety, and efficacy of these radiosurgical delivery systems for various lesions of the CVJ.
Historical Aspects and General Principles of Radiosurgery
The history of radiosurgery dates back to the 1950s in Stockholm, Sweden, where Lars Leksell developed a means of precise delivery of a single high dose of radiation capable of noninvasive destruction of deep tissue in the cranium.3 As it evolved, SRS became particularly useful for lesions not easily accessible or safely amenable to open surgical intervention. Today the indications for radiosurgery have evolved to more complex patient treatment algorithms.
Radiosurgery was first performed with the first Gamma Knife unit (Elekta, Stockholm, Sweden) in the 1960s in Sweden.3 The Gamma Knife used a cobalt-60 source to produce gamma rays that converge on a central point that represents the pathology of interest. In this unit, the head is rigidly fixed in a collimator helmet, with the target lesion more or less in the center of the helmet. Unlike radiotherapy, which is typically practiced by radiation oncologists, radio-surgery is surgical in intent and relies on spatial accuracy and precision with the goal of ablation of a precise target by utilizing a sharp dose fall-off to spare adjacent normal tissue.4 Conversely, radiotherapy relies on the “four Rs” of reoxygenation, reassortment, repopulation, and repair to slow neoplastic growth in a generalized region of the brain while minimizing damage to surrounding brain based on the principles of dosimetry.5 Essentially, radiosurgery is surgery for which the surgeon utilizes ionizing radiation rather than mechanical energy (via the edge of a scalpel blade) to remove or destroy tumor with surgical precision. As Leksell noted:
The term stereotactic radiosurgery has been used deliberately to stress the fact that this combination of mechanically directed instruments and modern radiation physics is still surgery, albeit using another physical agent in place of the knife or radiofrequency heat lesion… The Gamma Unit merely represents a change in the type of energy used… the same condition may be treated best in one patient with microsurgery and in another by stereota[ctic surgery]… The simplicity of using the Gamma Unit makes this integration possible… Someone competent in both techniques is best fitted to decide where the boundaries between the two methods should lie.3
Because radiosurgery is used as a surgical tool, SRS is typically performed by a multidisciplinary team comprised of a neurosurgeon, a radiation oncologist, a radiation physicist, and a radiology technician.
The Gamma Knife uses a 192–201 photon cobalt-60 source delivery system that emits gamma ray photons as high-energy beams. These beams converge on a central target point, which is the only location where enough energy is produced to promote tissue destruction through absorption of radiation. Tissue destruction generally occurs over weeks to months.6 Typically, the goal of radiosurgery using the Gamma Knife is to construct a treatment plan that exposes the entire lesion to the prescription dose of radiation at the 50% isodose line while keeping the dose to brainstem and other critical structures below tolerated levels. Numerous reports have detailed the use of the Gamma Knife for treatment of the CVJ.1,7,8
In the 1980s, neurosurgeons and radiation oncologists began modifying linear accelerators to perform radiosurgery for cranial lesions, including those of the CVJ.9,10 While there are some differences in technique and custom, including the practice of treating lesions at the 70 to 90% isodose line rather than the 50% isodose line, the general principles of radiosurgery using LINAC systems are the same as those for Gamma Knife. An additional advantage is that various LINAC systems developed bite blocks to allow radiosurgery without a head frame.11 However, concerns about the precision and reproducibility of this approach somewhat limited their widespread utilization, particularly for lesions near critical structures such as the brainstem and the optic nerve.
The CyberKnife SRS system (Accuray Inc., Sunnyvale, CA), developed by neurosurgeon John Adler, is a LINAC-based system with an integrated industrial robot that compensates for the lack of precision and reproducibility inherent in the frameless devices and for the potential for patient movement.12–14 This compensation is achieved by incorporating a real-time imaging system to check for changes from planning or “ideal” position, which then allows the robot to adjust the beam in compensation.12–14 An orthogonal pair of X-ray cameras takes images seconds before beams are delivered at each nodal position by a dynamic robot-mounted LINAC with six degrees of freedom. These images are then reconstructed into a digitally reconstructed radiograph (DRR) that compares them to the ideal or planning position at the time of planning and adjusts for variations in x, y, and z coordinates as well as pitch, yaw, and roll. The robotic arm then makes the required adjustments to target the radiation beams to the lesion with a spatial accuracy of 1.1 mm.15 BrainLAB (Westchester, IL), in cooperation with Varian Medical Systems, has developed a gantry-mounted LINAC system called Novalis Tx that utilizes DRR to similarly target and track movement of the cranium and spine.16 Finally, TomoTherapy (Accuray Inc.) incorporates an axial computed tomography scanner into a gantry-based LINAC system to image and compensate for changes in position of the cranium and spine.17 Numerous reports have detailed the efficacy of these devices for the treatment of lesions of the CVJ.18–22
Although frame-based radiosurgical technologies, including Gamma Knife and many LINAC-based systems, are limited to the treatment of lesions of the cranium and high cervical spine, the CyberKnife and Novalis systems are also capable of radiation delivery with high accuracy to lesions throughout the spine.14,23 These developments have enabled routine radiosurgical treatment of CVJ lesions that extend into the lower cervical spine. In addition, this frameless technology allows for fractionation of radiation, which is difficult to do with frame-based systems because of the morbidity of frame placement and because even slight changes in positioning/repositioning of the frame might account for major changes in the treatment plan. More recently, several of the frame-based platforms have developed bite blocks to allow fractionation using conventional platforms.
The radiobiology of radiosurgery also differs somewhat from conventional radiotherapy. Radiotherapy relies on delivery of radiation over large intracranial volumes that include normal brain structures. Fractionation is employed because normal brain repairs damage between fractions to a greater extent than do tumor cells.4 Conversely, radiosurgery delivers the entire dose of radiation in one to five fractions, and these small numbers of high-dose fractions provide approximately three times the biological effectiveness of fractionated conventional treatment.4 However, dose inhomogeneity is a noted part of Gamma Knife treatment in which the center of a target receives more radiation than the periphery.6 In general, SRS is limited for tumors less than 3.5 cm in size because treatment of larger volumes may minimize the precision of radiosurgery and increasing the fall-off can put surrounding normal tissues at risk of receiving high radiation doses.
However, like conventional radiotherapy, radiosurgery, whether a single fraction or up to five fractions, is typically delivered on an outpatient basis. On the day of treatment, a rigid stereotactic head frame, mouthpiece, or mask is placed, helping to stabilize the target and eliminate subcentimeter patient movement. In addition, the frame provides exact coordinates for target and treatment plan localization. Before placement, patients are appropriately medicated. In frame-based radiosurgery, the frame is attached to the skull via pins inserted on the frame: two frontal and two occipital. This procedure is performed by a neurosurgeon, usually with the administration of local anesthetic and mild sedation. Sometimes a bite block is used as a substitute for frame placement, particularly when fractionated stereotactic radiosurgery (FxSRS) is contemplated. After frame placement, image acquisition is performed.
Radiosurgery is comprised of two stages: planning and delivery. Planning for radiosurgery cases involves a combined effort between a neurosurgeon, radiation oncologist, and medical physicist. In general, the images are transferred to treatment planning software and the quality of the images is reviewed, which can be done by the physicist or one of the clinicians. If the quality is accurate, the images are co-registered with the frame or whatever non–frame-based reference system will be used. Co-registration is generally done by the neurosurgeon, often with support of the medical physicist. Finally, the target lesion as well as the critical anatomical or functional regions to avoid are contoured by the neurosurgeon with the treatment planning system software ( Fig. 18.1 ). Various collimator sizes that correspond to the diameter of the beam are available (4, 8, 14, and 18 mm for Gamma Knife). In addition, the beam can be more precisely “shaped” using various other approaches unique to each treatment platform. Examples include hybrid collimators in the new Gamma Knife Perfection, the adjustable IRIS used in the CyberKnife system, and the beam-shaping software, which employs mini microleaf collimators used by BrainLAB and Varian. Typically, to maximize conformality and minimize dose to surrounding normal structures, multiple versions of the plan are made as the plan gets more refined. The net effect is a highly conformal treatment plan that precisely demarcates the lesion to be treated. The radiation oncologist and neurosurgeon then choose a treatment dose based on conformality, treatment effect on surrounding normal structures, and radiation sensitivity of the pathology being treated.

The goal of radiosurgery is control of the tumor rather than the complete elimination that might be achieved with conventional surgery. Thus, treatment selection is critical. Large tumors in accessible locations or those associated with clinical symptoms are preferentially treated surgically; smaller tumors and those in less accessible locations as well as those in older, more debilitated patients are often treated with radiosurgery with the goal of tumor control rather than tumor elimination.
It has been increasingly recognized that tumor biology is also relevant to radiosurgical treatment. Malignant tumors require higher radiation doses, whereas benign tumors require less. As treatment volume increases, the dose delivered is lowered to prevent adverse reactions to the surrounding normal tissues. Different dose regimens are used for specific tumors, and data are often extrapolated from the experiences previously reported in the literature. Dose-volume histograms should be carefully examined to determine whether the dose planning is safe, and the conformality of the treatment plan should also be carefully reviewed.6 Although most patients are safely treated with radiosurgery, it is important to note that radiosurgery is not without risk. Adverse effects include skin reactions, tissue swelling, and severe radiation necrosis. Radiation necrosis is generally due to the surrounding normal brain reacting to the death of tumor cells, and some necrosis of normal tissue surrounding previously irradiated tumors can also be responsible for this phenomenon.6,24 Radiation necrosis is usually noted 6 to 24 months after initial radiation.24 Treatment is generally conservative with corticosteroids, but some patients with significant symptoms may require surgical resection of the areas of radiation necrosis. Recent studies suggest that hyperbaric oxygen, anticoagulation, and/or bevacizumab may be efficacious in the treatment of radiation necrosis, but these methods remain controversial.25 The risk of developing radiation reactions is dose-volume dependent and related to the dose of normal rather than abnormal tissues.
Although planning SRS treatment shares many commonalities across platforms, treatment may vary with the platform selected. Gamma Knife requires a stereotactic frame, which fits precisely in a helmet containing the collimators. The helmet and patient are then rolled into the Gamma Knife unit where the cobalt-60 sources sit, and the frame is moved around in the helmet to align the various “shots” that have been planned for the tumor. In the most recent versions, these movements are performed robotically by moving the frame itself (Version 4C) or moving the entire treatment couch (Perfection). In contrast, LINAC systems utilize photons produced by accelerating electrons along a linear path that collide with a tungsten target plate producing 6-MV X-rays for treatment.10 Unlike the Gamma Knife in which radiation sources are stationary, LINAC systems typically move in a rotational arc around the patient′s head and the bed changes positions, which changes the gantry and delivery angles and results in numerous noncoplanar arcs around the patient′s head.26 Lastly, the CyberKnife utilizes a smaller LINAC mounted on an industrial robot arm that tracks the patient′s movements in a near real-time fashion and “paints” the tumor with ionizing beams in a noncoplanar fashion from one of the 400 nodal points in its firing range with six degrees of freedom.12
In some of the more advanced systems, such as BrainLAB/Varian and CyberKnife, mini-multileaf collimators can be used to shape the radiation beam for optimal conformality. Increasingly, LINAC systems allow for use of bite blocks and masks, resulting in reproducible positioning and immobilization, which also allows for dose fractionation. Thus, many LINAC platforms are capable of FxSRS as well as fractionated image-guided radiotherapy, unlike frame-based systems, which are used primarily for single fraction SRS.4 Currently available treatment platforms are presented in Table 18.1 for comparison.
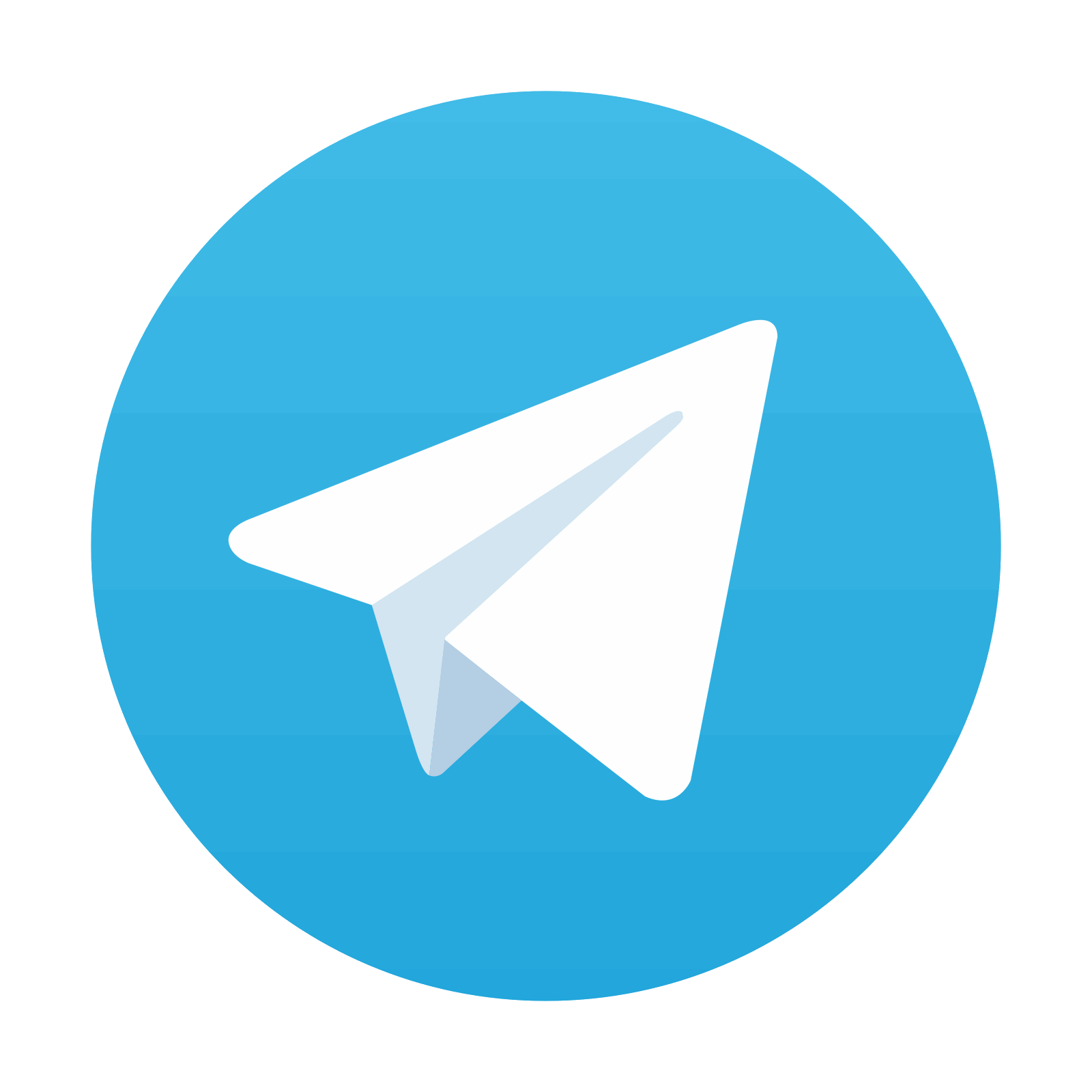
Stay updated, free articles. Join our Telegram channel

Full access? Get Clinical Tree
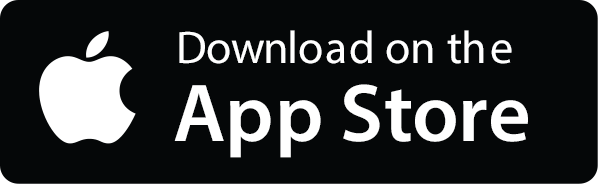
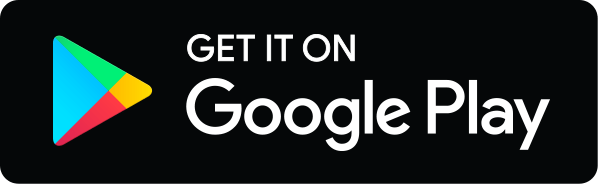