Introduction
Radiotherapy has been successfully used for many decades to control tumor growth and hormonal hypersecretion of pituitary adenomas. Although initially used as a primary treatment for these neoplasms, in the 1970s radiotherapy’s role evolved as an adjunctive treatment to transsphenoidal surgery for these tumors. While many patients with pituitary adenomas are cured by surgery, a significant number of patients have recurrent, invasive, or incompletely removed tumors and are at substantial risk for morbidity from tumor progression. Radiotherapy has been effective in reducing the risk of tumor regrowth and preventing tumor mass effects on adjacent brain structures in these patients. Radiotherapy has also successfully controlled excessive hormone production in patients with hypersecreting tumors who have failed surgical and medical treatment. Additionally, medically inoperable patients and patients who refuse surgery can be effectively treated with radiotherapy.
History
A wide variety of types of radiotherapy have been used to treat pituitary adenomas in past decades. Initial treatments included both brachytherapy and external beam radiation therapy given via low energy (orthovoltage) devices, and subsequently Cobalt-60 machines. The poor tissue penetration characteristics of these radiation beams sometimes led to radiation injury to the temporal lobes adjacent to the pituitary region, prompting the development of radiotherapy technologies which achieved better localization of the radiation dose to the tumor and spared adjacent brain tissues. Proton and heavy particle beams generated by cyclotrons, which take advantage of the Bragg peak effect of charged particle radiation to localize the radiation dose to the pituitary region, have been used with success in treatment of pituitary adenomas.
In the 1970s the development of the medical linear accelerator made effective pituitary radiotherapy widely available. The high energy x-ray beams generated by these machines coupled with new imaging technologies allowed for increased precision in radiation dose delivery, very substantially reducing late complications of pituitary radiotherapy. Additionally, devices were developed for stereotactic radiosurgery, where radiation treatment is delivered in a single session rather than protracted over a series of weeks. These devices include the Gamma Knife, which was developed in the late 1960s, followed by specially modified linear accelerators in the 1980s. The improved radiation dose localization achievable with these devices represented a further advance in the potential for tumor control and reduction in risk of brain injury.
Basic Principles
Ionizing radiation deposits its energy in living tissues, ultimately leading to damage to both healthy and neoplastic cells; selectively destroying tumors while sparing normal tissues is the objective of radiation treatment for both benign and malignant tumors. Biological and physical processes can be exploited to achieve this selective effect.
The key cellular target for radiation injury is nuclear DNA. Ionizations caused by radiation may damage DNA by direct or indirect mechanisms, which may be repaired in certain circumstances. However, double-stranded breaks in DNA caused by ionizing radiation may be lethal for the affected cell, leading to reproductive death when the cell undergoes mitosis. Normal and neoplastic tissues vary in their ability to repair radiation injury, and the sensitivity of cells to radiation damage varies with phase of the cell cycle and tissue oxygenation. Conventional radiotherapy employs fractionation of radiation dose into a series of treatments over a period of weeks to exploit these radiobiological principles to increase tumor control rates and minimize complications of treatment.
Confining high radiation doses to the tumor volume while excluding adjacent tissues from significant radiation exposure represents an additional method of selectively destroying tumors while reducing risks of normal tissue injury. This is the essence of stereotactic radiosurgery, but is a key principle of fractionated radiotherapy for pituitary tumors as well.
Normal Tissue Tolerance
The main factors that determine radiation tolerance of the central nervous system are radiation dose, fractionation, treatment time, and treatment volume. The risk of injury from conventional radiotherapy is expressed as a function of these parameters. The risk of brain necrosis is approximately 5% when 60 Gy is given in 30 fractions over 6 weeks to partial brain radiotherapy fields. Additionally, critical anatomic structures within the brain including the optic nerves and brainstem have lower radiation tolerances, which is an important factor in radiotherapy of pituitary tumors.
The brain is highly sensitive to radiation fraction size. Many reports of radiotherapy-related brain injury in the literature, particularly in earlier years, included patients treated with daily radiation fractions in excess of 200 cGy, which have demonstrated to significantly increase the risk of brain necrosis and other complications.
Dose and Fractionation
The optimal dose and fractionation regimen for treatment of pituitary adenomas is somewhat controversial. Although some authors recommend doses in the 50 to 54 Gy range, other reports suggest no increase in tumor control at doses exceeding 45 Gy. The probability of brain injury increases as dose and fraction size increase, and it is unclear that any benefit is achieved using regimens exceeding 45 Gy given at 1.8 Gy per fraction over 5 weeks, which has been demonstrated to provide excellent long-term control of pituitary adenomas with a very low risk of complications. Limiting daily fraction size to 1.8 Gy is recommended to reduce the risk of optic injury. There is no clear evidence that the various types of pituitary adenomas require different radiation doses for tumor control.
Radiotherapy Techniques
Radiotherapy attempts to deliver a homogeneous dose to the tumor while excluding surrounding brain tissues from the volume that receives a high radiation dose. As radiotherapy techniques have evolved, treatment volumes have become progressively more conformal. Initially, external beam radiotherapy for pituitary tumors was delivered through lateral opposed treatment fields, resulting in a significant dose to the temporal lobes and an increased risk of brain injury, particularly when lower energy x-ray beams were employed. Conventional radiotherapy techniques were modified to use at least three beams intersecting on the pituitary region or rotational arc fields, reducing the dose to the temporal lobes. High energy (>15 Mv) x-ray beams introduced in the 1970s also allowed increased sparing of superficial brain tissues, reducing the risk of complications.
With the advent of CT and MRI, precise tumor localization became possible, and conformal radiotherapy based on these imaging modalities was developed. This technology employs a beams-eye view and multileaf collimator beam-blocking techniques, resulting in improved radiation dose distributions with reduced dose to normal brain tissues. Further improvement in radiation dose distributions was achieved with the development of intensity modulated radiotherapy (IMRT), where the radiation treatment planning process is automated based on specified parameters, such as tumor margin dose and dose to the optic apparatus, enabling selective sparing of critical structures, such as the optic nerves, chiasm, and hypothalamus. Significant improvements in linear accelerator accuracy and patient immobilization have also considerably increased the precision of radiotherapy.
Linear Accelerator–Based Radiosurgery
In contrast to radiotherapy, where treatment is delivered in multiple fractions over a period of weeks, radiosurgery delivers a precisely localized radiation dose to the tumor volume in a single session. Although the large majority of patients with pituitary adenomas who have undergone radiosurgery have been treated with the Gamma Knife, linear accelerator–based techniques have also been employed. A number of these devices are presently in use, including the BrainLAB, Peacock, X-knife, and CyberKnife systems. In many radiosurgery systems, stereotactic head frames are fixed to the skull, achieving precise patient immobilization. Alternatively, frameless radiosurgery has also been used. The CyberKnife uses image-guided robotic radiosurgery to compensate for movement of the patient or tumor during radiosurgery, resulting in very precise, highly conformal dose distributions to the target volume.
Radiosurgery is highly effective for pituitary adenomas when treatment volumes are not extensive. Patients with smaller volume tumors (e.g., maximum tumor diameter 3 cm or less), who have sufficient distance (usually greater than 3 mm) between the tumor and the optic apparatus, are excellent candidates for radiosurgery. Tumors typically remain unchanged in size or partially regress after radiosurgery; complete response is uncommon. Tumor growth stabilization is achieved in greater than 90% of pituitary adenoma patients treated with radiosurgery.
The sensitivity of the optic apparatus to single doses of radiation is a key consideration in radiosurgery, with a range of tolerance doses reported. Ideally, the dose to the optic nerve is limited to 8 Gy; at this dose, visual complications of radiosurgery are extremely unusual, although up to 9 Gy may be delivered with a very small risk of optic injury. The sensory and motor nerves of the cavernous sinus have a significantly higher tolerance (>40 Gy) to single radiation doses. Prior radiotherapy may significantly increase the risk of visual complications from radiosurgery.
Results
Control of Tumor Growth
Conventional radiotherapy is highly effective in preventing tumor growth of pituitary adenomas. It is particularly useful in patients with extensive macroadenomas for which complete resection is not possible or radiosurgery is contraindicated due to tumor size or proximity to the optic apparatus. Patients with recurrent tumors after surgery, poor operative candidates, and patients who refuse surgery may also be successfully treated with fractionated radiotherapy.
Long-term control of pituitary adenomas with radiotherapy is well documented. In a large series of patients with nonsecreting adenomas treated with radiotherapy reported from Royal Marsden Hospital, the 10-year and 20-year progression-free survival rates were 97% and 92%, respectively. Tumor volume influenced control rates in this study, with a 100% tumor control rate for intrasellar tumors (31 patients), 92% for macroadenomas less than 2 cm (180 patients), and 91% for tumors greater than 2 cm (34 patients). Similar results were reported from the University of Pittsburgh, with 10-year and 20-year progression-free survival rates of 89% and 76%, respectively. A number of other reports in the literature have documented similar results. Long-term follow-up is necessary, as late recurrences can occur postradiotherapy. Radiosurgery has been used as an effective salvage therapy in this situation, as has repeat radiotherapy.
Acromegaly
Radiotherapy is effective in reducing growth hormone levels in patients with acromegaly, with a number of reports in the literature documenting its efficacy. The time course of hormonal response after treatment is typically slow, often requiring a number of years for the growth hormone level to reach its nadir. An estimate of the rate of growth hormone reduction after radiotherapy is 50% after an interval of 27 months, with the median time to normalization ranging from 2 to 7 years.
The pretreatment growth hormone level may influence the time to maximum hormonal response, with patients with higher levels requiring longer intervals to achieve normalization. Although different measures of treatment success have been used in the literature, the percentage of patients achieving growth hormone levels below 10 ng/mL at 10 years after radiotherapy ranges from 60% to 100%. Patients with persistently elevated or recurrent, rising hormonal levels after surgery should receive radiotherapy or radiosurgery.
Cushing’s Disease
Cushing’s disease patients with persistently elevated hormone levels or recurrent tumors after surgery may be successfully treated with radiotherapy. Hormonal normalization rates ranging from 50% to 100% have been reported in the literature for patients with ACTH-secreting adenomas receiving radiotherapy. Alternatively, these patients may be successfully treated with radiosurgery. Rates of hormonal response after radiotherapy are faster in Cushing’s disease than in acromegaly, with normalization typically occurring within 2 years after treatment.
ACTH-secreting tumors are usually small and are frequently cured by surgery. However, recurrence rates are significantly higher in patients with macroadenomas or invasive tumors, and postoperative radiotherapy is useful in preventing tumor recurrence in these situations; radiotherapy is also effective as primary therapy in patients who are poor surgical candidates.
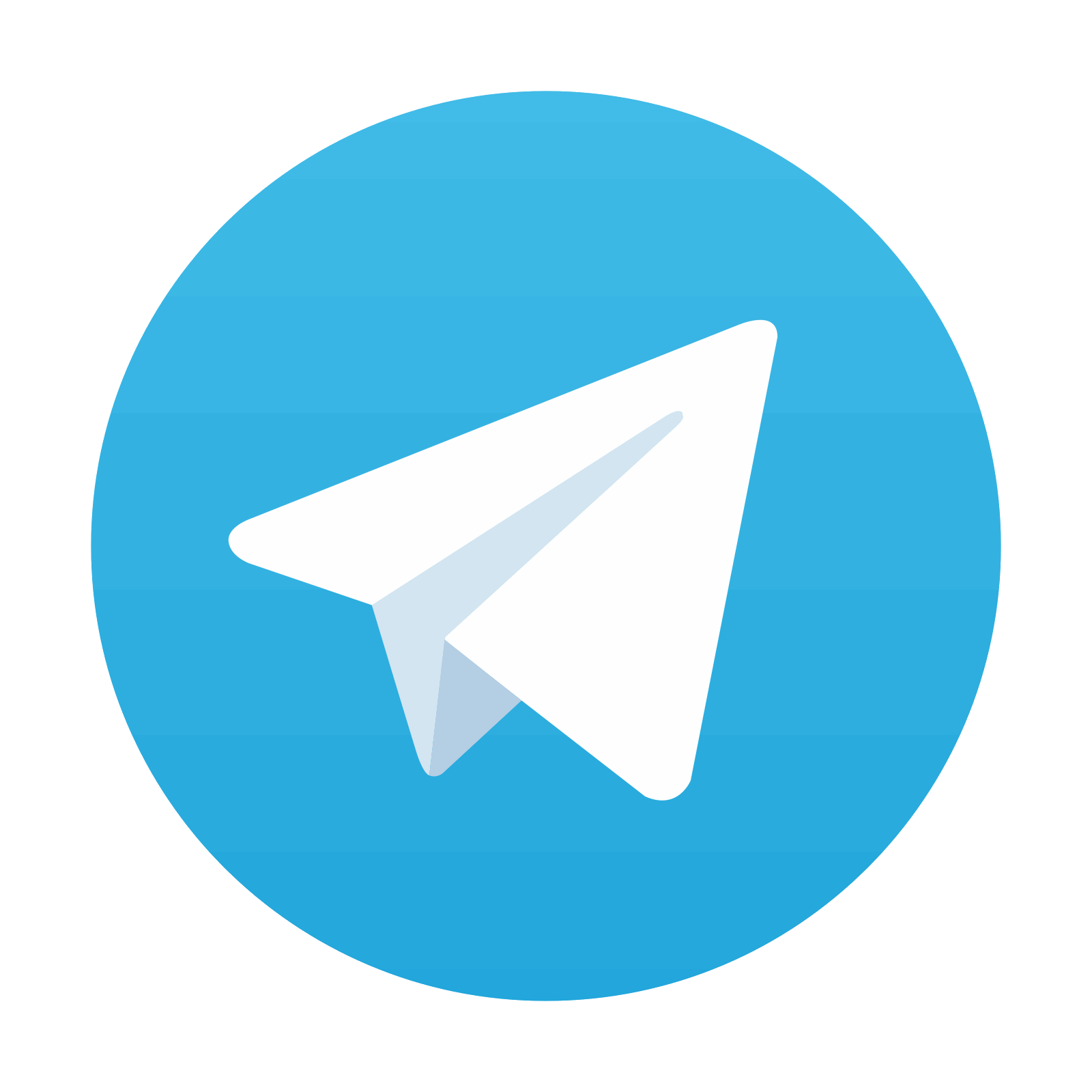
Stay updated, free articles. Join our Telegram channel

Full access? Get Clinical Tree
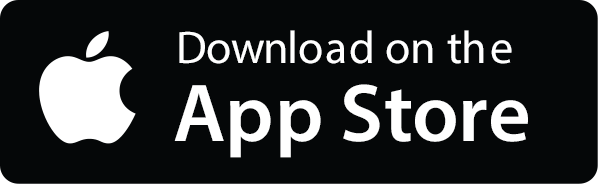
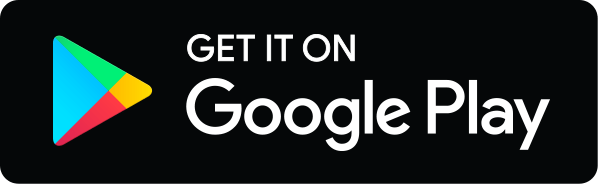