17 Raman Spectroscopy and Brain Tumors Abstract Keywords: Raman spectroscopy, hyperspectral Raman microscopy, coherent Raman imaging, stimulated Raman scattering microscopy, stimulated Raman histology, coherent anti-Stokes Raman scattering microscopy, label-free histology, intraoperative pathology Image-guided neurosurgery for the treatment of central nervous system (CNS) tumors has resulted in greater extent of resection and improved patient outcomes.1,2,3 Intraoperative imaging techniques allow neurosurgeons to evaluate residual tumor burden that would have otherwise gone undetected.4,5 Fluorescence-guided neurosurgery represents a major advancement for the surgical treatment of CNS tumors in the 21st century. Wide-field fluorescence imaging using 5-aminolevulinic acid (5-ALA) in high-grade gliomas enables more complete tumor resections, thereby improving progression-free survival. However, any imaging method that relies on labeling agents will face the challenge of nonspecific or nonsensitive tumor detection in the operative setting.6 Label-free methods rely on the intrinsic biochemical properties of normal and lesional tissue to provide image contrast. Raman-based imaging modalities have emerged as promising label-free methods for the detection and diagnosis of primary and metastatic CNS tumors.7,8,9 Spontaneous Raman spectroscopy and coherent Raman scattering (CRS) microscopy have both found applications in image-guided neurosurgery with promising results. Here, we provide a brief description of spontaneous and CRS. We then detail the applications of Raman-based modalities to image-guided neurosurgery. Elastic photon scattering (i.e., Rayleigh’s scattering) occurs when an incident photon has the same wavelength as the scattered photon after interacting with a media or tissue. The majority of photons in the visible spectrum (380–750 nm) are scattered elastically. A small proportion of photons transfer energy to (red-shift, Stokes scattering) or absorb energy from (blue-shift, anti-Stokes scattering) the object being imaged. This transfer of energy results in inelastic scattering and is called the Raman effect, discovered in 1930.10 The energy difference between the incident and scattered photon is called the Raman shift and is measured in wavenumbers (waves per centimeter). The Raman effect is weak relative to elastic scattering. However, using narrowband laser excitation and a sensitive spectrometer, Raman scattering can be detected and measured to generate spectra from the incident tissue. Raman spectroscopy has wide applications in chemistry and biology for its ability to characterize individual molecules and biological tissues. The Raman spectrum of a molecule can be determined by measuring the Raman shifts caused by interactions with each of its constituent chemical bonds. Raman spectral peaks correspond to specific vibrational modes (e.g., stretching, bending, or scissoring) produced by the chemical bond. For example, the –CH2 symmetric stretching mode plays an essential role in characterizing biological tissues using Raman spectroscopy due to its high concentration in fatty acids. Raman spectra can therefore be used as a means to quantify the chemical composition of biological tissues. The vibrational fingerprint of biological tissues is a product of its composition of macromolecules (i.e., nucleic acids, protein, and lipids) and the sum of the vibrational spectra of the tissue constituents. Investigators hypothesized that the chemical differences between normal tissue and tumor may produce sufficiently distinct Raman spectra to allow accurate detection of brain tumor infiltration. Early investigations using spontaneous Raman spectroscopy to characterize brain tissue were conducted in the 1990s and involved distinguishing normal versus edematous brain.11 A strong 3,390/cm Raman peak corresponding the O–H stretching mode identified greater water content in edematous brain. Krafft and colleagues were instrumental in performing influential early studies on the applications of Raman spectroscopy in neuro-oncology.12 After performing a detailed spectral analysis of 12 major brain lipids,12 they were able to differentiate glioblastoma tissue from healthy brain tissue using lipid and water content and they confirmed these differences using mass spectroscopy.13 The same group performed a landmark proof-of-concept study employing Raman spectroscopy to detect intracerebral tumors in vivo by brain surface mapping.14 In a murine metastatic brain tumor model, hyperspectral Raman spectroscopy covering 3.6 × 3.2 mm of cortical surface was able to detect melanotic tumors in the murine brain. The Raman spectra of the metastatic tumors display additional Raman shifts near 597, 976, 1,404, and 1,595/cm due to melanin pigment contribution. Several studies have investigated using Raman spectroscopy for intraoperative brain tumor biopsy guidance. Identifying regions of vital tumor in glioblastoma increases the diagnostic yield of biopsy specimens. Necrotic specimens from brain tumor biopsies show a prominent Raman peak at 1,739/cm, consistent with significantly higher concentration of cholesterol and cholesteryl esters.15 Using linear discriminant analysis of specimen spectral data, accuracy for discriminating necrotic versus vital tumor approaches 100% ( Improvements in fiber-laser technology have allowed for the development of a Raman spectroscopy probe system for in vivo intraoperative brain tissue classification.18 In a recent clinical study, a handheld fiberoptic probe was placed in direct contact with the brain in the resection cavities of 17 patients with gliomas (low and high grade; Fig. 17.1 Raman spectroscopy for discriminating normal brain, necrosis, and tumor tissue. (a) Mean spectrum of normal, necrosis, and tumor from training data, with visible Raman peaks labeled. (b) Plot of discriminant function analysis scores for training data. (Reproduced with permission from Kalkanis et al.8) Fig. 17.2 Correlating hematoxylin and eosin (H&E) and Raman images for five representative tissues. (a) Standard H&E histology, (b) LFB staining, (c) concentration of phenylalanine (1,004/cm) on red channel, (d) ratio of 1,300:1,344/cm shows approximate lipid:protein ratio on green channel, (e) concentration of 1,660/cm on blue channel, and (f) combined red, green, and blue images. W, white matter; G, gray matter; N, necrosis; GBM, glioblastoma; each Raman image pixel represents an area of 300 µm x 300 µm. Note: The upper left area of tissue HF140B, identified as white matter on histology review, exhibited pallor on both LFB and H&E staining, which appears to be artifactual, potentially due to tissue freezing or edema. (Reproduced with permission from Kast et al.17)
Label-free imaging techniques rely on the intrinsic biochemical properties of tissues to generate image contrast and identify tumor infiltration. Spontaneous and coherent Raman scattering techniques allow for chemical characterization of the underlying tissue. Raman spectroscopy can detect variations in water, lipid, protein, and cholesterol content of normal versus brain tumor tissue. Raman spectra analysis allows neurosurgeons to reliably differentiate normal tissue, necrotic, and viable tumor in fresh and frozen surgical specimens. By focusing on specific regions of the Raman spectra, coherent Raman scattering microscopy can be used to rapidly generate histologic images. Stimulated Raman scattering (SRS) microscopy can detect brain tumor infiltration with high (> 90%) accuracy. Advances in fiber-laser technology have allowed for the development of a clinical SRS microscope for intraoperative brain tumor diagnosis. In stimulated Raman histology, a hematoxylin and eosin color scheme is applied to SRS data, yielding a label-free intraoperative pathology method, enabling rapid and accurate brain tumor diagnosis. In this chapter, we review how Raman-based techniques can improve the surgical management of brain tumor patients by detecting tumor infiltration, guiding tumor biopsy/resection, and providing label-free images for accurate histopathologic diagnosis.
17.1 Introduction
17.2 Overview of Spontaneous Raman Scattering
17.2.1 Raman Spectroscopy for Brain Tumor Detection
Fig. 17.1). Leveraging these spectral differences, Kalkanis and colleagues were able to differentiate normal brain, necrosis, and glioblastoma tissue in frozen brain tumor sections with an accuracy of 97.8%.8 In a subsequent investigation, five frozen sections (normal, necrosis, dense glioblastoma, and two infiltrating glioblastoma) were mapped at five Raman shift wavenumbers across the entire specimen in 300 μm2 step sizes.16 Tissue maps were able to identify boundaries between gray and white matter, necrosis, glioblastoma, and infiltrating tumor. Further narrowing to three key Raman spectral regions allowed for the development of a virtual red, green, and blue (RGB) color scheme.17 Red (1,004/cm channel), green (1,300:1,344/cm channel), and blue (1,600/cm channel) color scales were assigned to Raman peak intensities and fused for multichannel imaging (
Fig. 17.2). The multichannel color maps were used to discriminate white matter, gray matter, and tumor tissue with diagnostic accuracy of approximately 90% on a cross-validation.
Fig. 17.3).19 Time to image acquisition was 0.2 seconds for each area interrogated and measured 0.5 mm in diameter; sampling depth of the probe was 1 mm. Using a boosted tree method to analyze intraoperative Raman spectra, the investigators were able to distinguish normal brain from tumor-invaded brain (> 15% tumor cell invasion) with an accuracy of 92% (sensitivity = 93%; specificity = 91%). Both low- and high-grade gliomas were able to be detected with similar accuracy. These results represent a promising step forward toward translating Raman spectroscopy to real-time intraoperative use and brain tumor resection guidance.
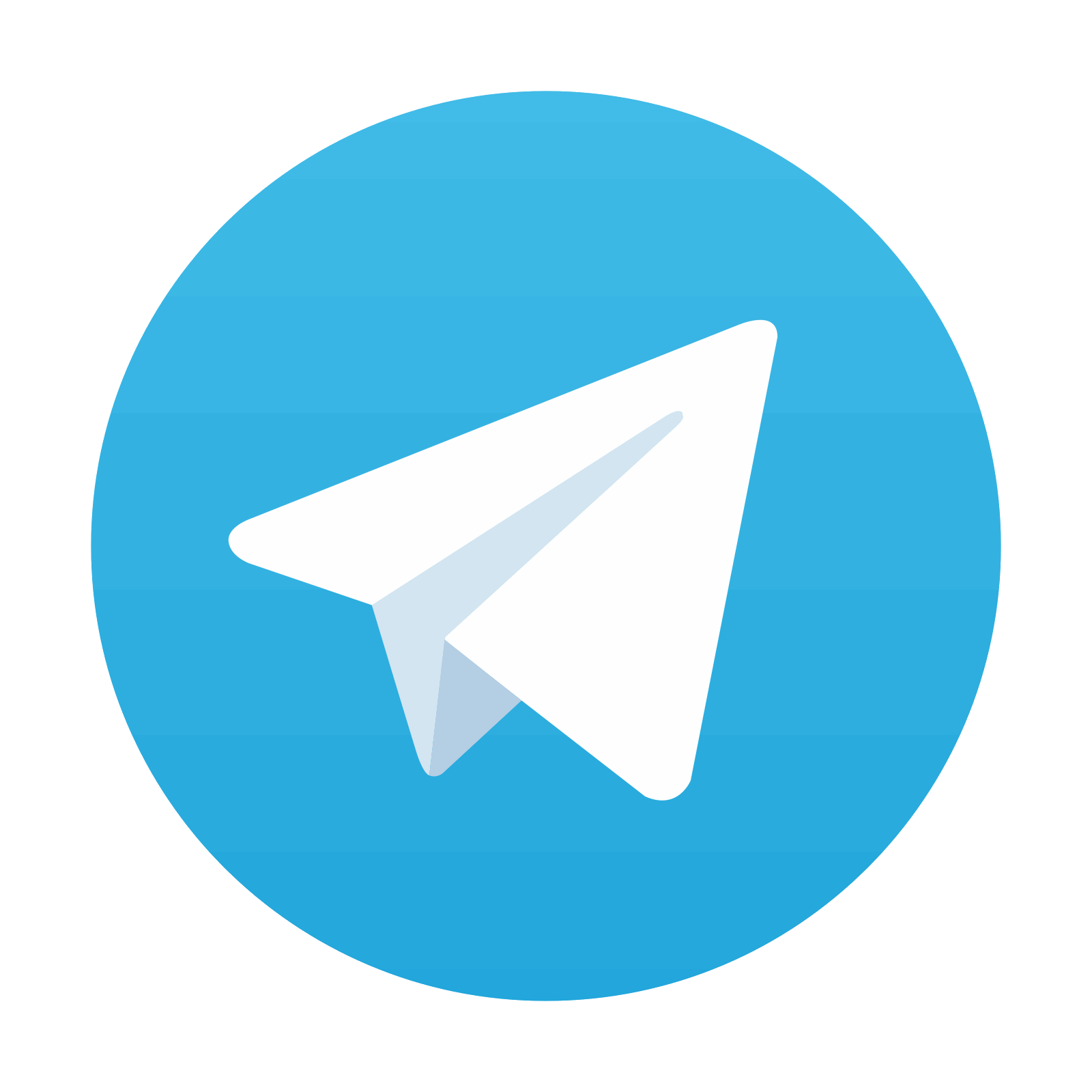
Stay updated, free articles. Join our Telegram channel

Full access? Get Clinical Tree
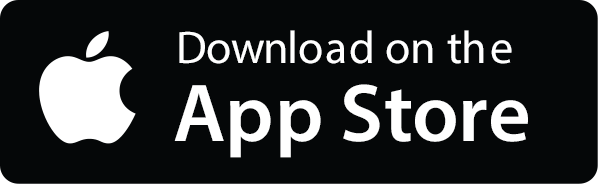
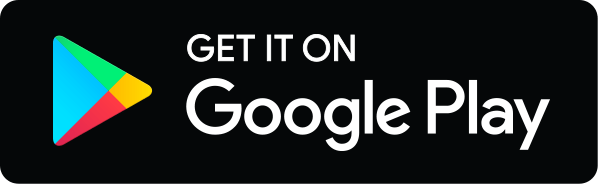