Fig. 49.1
Muscle tone during REM sleep in a normal subject, and in patients with REM sleep behavior disorder. The figure shows representative examples of 30 s polysomnogram epochs recorded during REM sleep. Muscle tone in the chin (submentalis), legs (linked tibialis), and arms (linked extensor digitorum communis) are shown in the sixth, seventh, and eighth channels of each example epoch, respectively. a demonstrates a normal level of REM sleep muscle atonia, while b–d demonstrate clear excessive phasic and tonic muscle tone, b shows predominantly excessive phasic muscle activity, especially in the leg, where there is also subtly increased tonic muscle activity. c demonstrates predominate excess phasic burst activity in the chin, arm, and leg muscle channels, accompanied by probable dream enactment manifested by vocalization; d shows excessive phasic and tonic muscle activity in the chin and leg muscle channels. Each of the examples seen in b–d is consistent with REM sleep without atonia, the neurophysiologic substrate of REM sleep behavior disorder
The Association of RBD with Neurodegenerative Synucleinopathy Disorders
RBD can result from several synucleinopathy phenotypes, as shown in Table 49.1. Both idiopathic and symptomatic RBD have been shown to be especially strongly associated with the synucleinopathies, a group of neurodegenerative disorders of unknown etiology related to intracellular accumulation of the protein alpha-synuclein, including Parkinson disease (PD), Lewy body dementia (DLB), multiple system atrophy (MSA), and pure autonomic failure (PAF) [5, 8, 12, 33, 37, 38, 67, 78]. Mild cognitive impairment (MCI), especially the non-amnestic subtype, has also been associated with RBD [5]. RBD is strongly associated with parkinsonism and/or cognitive impairment, which affects more than 82 % of RBD patients [36, 79]. Idiopathic RBD patients have an approximate 12–45 % risk of developing parkinsonism or dementia within five years of RBD symptom onset, which rises to 60 % within fifteen years, and to approximately 84 % risk 20 years after RBD symptom onset [5, 8, 79, 80].
Table 49.1
Symptomatic causes of RBD
Synucleinopathy disorders ∙ Parkinson disease ∙ Lewy body dementia ∙ Multiple systems atrophy ∙ Pure autonomic failure |
Tauopathies (rare) ∙ Progressive supranuclear palsy ∙ Alzheimer disease |
Narcolepsy |
RBD associated with antidepressant medication |
Paraneoplastic disorders |
Brain stem lesional pathology |
There is growing evidence in iRBD patients of frequent presence of “soft,” subtle neurological and neuropsychologic abnormalities (also known as non motor signs) consistent with the early stages of a developing synucleinopathy [5, 81–83]. Patients with iRBD show abnormalities in smell and color vision, similar to that seen in patients with PD [81–84]. Some useful batteries for assessment of vision and olfactory function include the Farnsworth-Munsell-100-Hue test (FM 100) for color vision, and the University of Pennsylvania Smell Identification test (UPSIT) and Brief University of Pennsylvania Smell Identification test (B-SIT) to test olfaction [81, 83]. These clinical assessments may help clinicians clarify which RBD patients are most likely to develop parkinsonism, and poor performance on the FM-100 and UPSIT tests appears to be good predictors for the development of impending neurodegenerative disease in patients with iRBD [81, 82, 84]. RBD patients without impaired olfaction have an 86 % chance of remaining disease free for 5 years, contrasting with a 35.4 % chance in those with abnormal olfaction [12]. Abnormal olfaction in RBD appears similar to olfactory deficits in previously diagnosed PD patients, indicating that progressive neurodegeneration in RBD leads to PD [81, 83]. In addition, RBD patients with impaired color vision have an 18–26 % chance of 5-year disease-free survival [12, 83].
Patients with idiopathic RBD and PD with RBD have difficulties with object identification compared to controls and patients with PD lacking RBD [82]. Neuropsychological performance can also be used as a potential predictor of progressive neurodegeneration in iRBD patients [78, 85]. Impairments in attention, executive function, decision-making abilities, verbal memory, and verbal learning are most common in RBD patients, similar to deficits seen in synucleinopathies [86, 87]. Because iRBD is likely an early manifestation of synucleinopathy, serial neuropsychometric testing may help determine the timeline and progression of neurodegeneration in iRBD patients. Similarly, quantitative electroencephalography (EEG) has demonstrated temporo-occipital slowing in patients with iRBD, with higher theta power similar to that seen in patients with DLB and PDD [86, 88]. Of note, mild cognitive impairment appears to be the driver of EEG slowing in iRBD patients and likely heralds impending MCI [89, 90].
Cognitive impairment in iRBD likely varies due to the time point at which patients are sampled. Patients with iRBD are a heterogeneous group and are considered “idiopathic” because they do not yet express clinical symptoms of neurodegenerative disease, but that does not mean patients in the reported studies are at the same point in the disease progression. Because we do not sample patients at a uniform time point in their supposed disease process, and we do not fully understand why patients progress at different rates, it is difficult to draw conclusions about accompanying cognitive, motor, and autonomic dysfunction that would apply to all iRBD patients [91]. RBD patients that have progressed further toward overt clinical manifestations of neurodegenerative disease would very likely have greater impairments in cognition; therefore, differences seen between controls and iRBD patients may be driven by those patients closer to the clinical expressions of synucleinopathies. To our knowledge, no studies have yet examined cognition in the very early stages of dream enactment behavior with subsequent longitudinal, serial follow-up to determine whether or not there is a discernible common pattern of temporal progression of cognitive decline in iRBD. Future studies examining early RBD are of significant importance in the hopes of better understanding the underlying neurodegenerative process in iRBD.
Autonomic dysfunction may be the earliest predictor of impending neurodegeneration in iRBD. Orthostatic blood pressure drop may present as early as 20 years prior to development of overt neurological signs of synucleinopathy [92]. Urinary incontinence (13 years), constipation (15 years), and erectile dysfunction (11.5 years) also begin long before other neurological symptoms [92]. In addition, there is increased cardiac denervation in patients with iRBD and PD, further indicating that RBD may be an early expression of PD in many cases. However, the amount of cardiac denervation does not appear predictive of neurodegenerative disease development [84, 93, 94]. Reduced 123I-MIBG uptake may begin as early as Braak Stage 1, indicating that cardiac scintigraphy may be useful in early identification of PD development. Since cardiac denervation is present in the earliest stages of PD and RBD, but the amount of denervation does not predict neurodegeneration, RBD may represent early PD in a subset of patients [94]. Notably, while 123I-MIBG scintigraphy may be significantly decreased in RBD and PD, iRBD patients have been shown to have even greater reduction of MIBG uptake when compared to PD patients without RBD and neurologically normal controls, possibly indicating that PD-RBD may be a specific PD phenotype [95, 96]. Recently, plasma urate levels have also been shown to be a possible predictive factor of synucleinopathy neurodegeneration, with higher uric acid levels reportedly associated with a decreased risk for PD development [97] and a longer duration of RBD without conversion to PD, suggesting that plasma urate may modulate the progression of neurodegeneration in RBD. However, significantly more research is required to draw definitive conclusions [97].
Imaging in RBD
Similarly, advances in objective neuroimaging tests have begun to allow the non-invasive identification of patients at risk of development of a neurodegenerative disorder [5, 7, 98]. These tests include dopamine transporter uptake 123I-FP-CIT SPECT (DaTscan) and transcranial sonography (TCS), which have shown to be effective in detecting subclinical changes in the substantia nigra that may reflect evolving synucleinopathy; and 99mTc–ethylene cysteinate dimer SPECT (ECD-SPECT), a measure of regional cerberal blood flow (rCBF) which has been shown to predict future development of PD and DLB [98]. These tests may be useful in the identification of patients who could participate in future clinical trials of neuroprotective therapies designed to slow progression or arrest neurodegeneration in patients with iRBD [7]. Abnormalities on TCS (i.e., substantia nigra hyperechogenicity) and DaTscan (i.e., 123I-FP-CIT binding deficiencies in the striatum) in iRBD patients appear predictive of those who subsequently develop more classic features of a synucleinopathy, whereas patients with no abnormalities on neuroimaging remained disease free during follow-up [7]. The sensitivity of combined TCS and DaTscan scans was 100 % for predicting development of neurodegenerative disease within 2.5 years, so DaTscan and TCS may be useful in diagnosing neurodegenerative disease before clinical symptoms of parkinsonism, cognitive decline, or dysautonomia appear [7].
ECD-SPECT scan showed increased hippocampal rCBF in all iRBD patients who developed PD or DLB when compared to iRBD patients who remained disease free [98]. Notably, patients who developed PD displayed increased perfusion in the hippocampus, pons, and anterior cingulate cortex, while patients who developed DLB had increased rCBF confined to the hippocampus. Increased rCBF was also associated with several clinical markers of neurodegeneration. UPDRS-III was positively correlated with rCBF in the right hippocampus, and olfaction was correlated with increased rCBF in the medial frontal gyrus, while impairments in color vision were correlated with increased perfusion in the pons and left hippocampus [98].
Cortical afferent inhibition during transcranial magnetic stimulation (TMS) study was shown to be decreased in iRBD patients when compared to controls and correlates strongly with poor performance in episodic verbal memory and executive function tasks, suggesting that cholinergic dysfunction may underlie cognitive impairment in iRBD [99]. In addition, voxel-based MRI morphometry (VBM) has recently shown gray matter loss in the left and right anterior cerebellum, pontine tegmentum, and left parahippocampal gyrus in iRBD patients, a pattern similar to neurodegeneration in DLB and MSA [100]. These findings indicate the utility of SPECT, TCS, TMS, and MRI scans to predict future neurodegeneration, and possibly synucleinopathy subtype in patients with iRBD, which may enable more accurate prognostication and individualized diagnostic and therapeutic approaches.
Finally, the degree of RSWA appears predictive for future PD development. Patients with a more significant degree of elevation in baseline mentalis tonic density were more likely to develop PD in one study [33]. RSWA increases over time in patients with iRBD, suggesting a progressive neurodegenerative process leading to the destruction of brainstem regions responsible for the control of REM sleep toward clinical manifestations of parkinsonism and dementia [101, 102]. However, RBD symptom duration may not be the correct measure to determine group comparability, and patients with higher RSWA densities may have been studied further along in their disease course toward the eventual expression of PD, so additional prospective studies are necessary to determine whether the degree of RSWA is a marker for progression to PD.
RBD and RSWA Association with Specific Synucleinopathy Disorders: Parkinson Disease, Lewy Body Dementia, and Multiple System Atrophy
RBD and Parkinson Disease
RBD is common in Parkinson disease (PD) with as many as 55 % of PD patients reporting RBD symptoms [12, 32, 103–105]. RBD symptoms can develop prior to the development of clinical PD symptoms or during any of the six stages of PD [67]. Depression has been shown to be a risk factor for future development of PD [106]. In addition, depression and antidepressant use have also been associated with RBD [28, 30, 51, 107]. PD patients with RBD are more likely to have a comorbid psychiatric disorder compared to PD patients without RBD, and iRBD patients with comorbid depression have an increased risk for development of PD compared to RBD patients without comorbid depression [96, 108].
There is some evidence that PD-RBD may be a specific PD subtype. PD patients with RBD are less likely to be of the tremor predominant phenotype, less levodopa responsive, have higher Hoehn–Yahr scores, more frequent hallucinations, a greater degree of autonomic dysfunction, and most important, an increased risk for dementia [95, 96, 105, 109]. PD-RBD patients have a 45 % risk of development of dementia within four years compared to a 0 % risk in PD patients without RBD [95]. Of note, only PD patients with clinical RBD symptoms were at an increased risk for future development of dementia, when compared with PD patients with RSWA on PSG but no history of dream enactment and PD patients with normal REM sleep [109]. This suggests that PD-RBD may be a more widespread disease process compared to PD patients without RBD.
PD-RBD patients may have a gender disparity for the type of dream enactment behaviors similar to that seen in iRBD [110]. Men with PD-RBD report more aggressive and violent dreams with a higher percentage of violent movements during sleep. However, women with PD-RBD report more disturbed sleep with less violent movements during sleep [110]. Clinical RBD symptoms may remit in PD patients and appear less injurious when compared with iRBD patients [41, 111, 112], possibly due to decreased functionality as PD progresses. However, motor activity appears improved during dream enactment behavior compared with awake motor activty, so decreased functioning may not be fully responsible for clinical RBD remission and the lesser injury potential due to RBD behaviors in PD patients [113, 114]. The smoothing of movements during dream enactment represents sleep-related intact corticofugal and corticospinal neural circuitry that bypasses the basal ganglia, where functioning is impaired by synucleinopathy during wakefulness [114].
RBD and Lewy Body Dementia
Cognitive impairment is frequent in RBD and many patients develop dementia, primarily DLB or PDD [67, 84, 115–117]. While DLB and PDD are similar, important differences exist in the time course for the development of cognitive symptoms relative to development of motor symptoms. If motor symptoms are present for >1 year before cognitive decline, patients are considered to have PDD. If motor symptoms are present for <1 year prior to cognitive decline, or if they develop anytime after the onset of cognitive decline, patients have DLB [118]. Dream enactment is common in DLB, and PSG-confirmed RBD is present in as many as 83 % of DLB patients [119]. In fact, DLB and RBD are so closely associated that including RBD as a core feature of DLB increases the likelihood of DLB diagnosis sixfold [117]. Visuospatial impairment is one of the hallmarks of DLB, but can also be present in PDD. In addition, executive functioning is also affected in patients with DLB and PDD [84, 115, 116]. Idiopathic RBD patients show visuospatial and visuoconstructive dysfunction similar to patients with DLB, indicating that RBD may be a predictor for the development of dementia [82, 84]. In addition, many RBD patients eventually express clinical and/or pathologic features of Lewy body dementia/disease [5, 78, 107, 116, 117, 120, 121]. DLB patients with RBD have a shorter duration of dementia, earlier onset of parkinsonism and visual hallucinations, and lower neuritic plaque scores than DLB patients without RBD, indicating that DLB-RBD is a specific subtype of DLB which may have more widespread neurodegeneration than DLB without RBD [121]. Further insights clarifying the association between dementia and RBD, especially determining which patients are at highest risk for deteriorating cognitive functioning, are needed.
RBD and Multiple System Atrophy
Of the synucleinopathies, multiple system atrophy (MSA) appears to be the most strongly associated with RBD, with 68–100 % of patients having RBD or RSWA [85, 122, 113, 123]. Why MSA is more strongly associated with RBD than PD or DLB remains unclear. RBD symptoms in MSA appear to be less severe, even though RSWA is increased, when compared with PD-RBD and iRBD patients, a possible consequence of the more widespread neurodegeneration in MSA and its impact on functionality [124]. A diagnosis of RBD may help differentiate MSA from pure autonomic failure (PAF); in one case series, four patients with MSA had dream enactment behaviors, while all six patients diagnosed with PAF had no sleep disturbances [123]. However, other studies have shown RBD also occurs in PAF, indicating that further research is required on the association of PAF with RBD [122]. RBD has been found to be equally common in both MSA-P and MSA-C subtypes, suggesting that RBD is not a reliable finding to differentiate between subtypes of MSA [125].
RBD and Tauopathies
There have been reported cases of RBD in Alzheimer’s disease, but the pervading theory is that concomitant Lewy body pathology instead may more likely explain the RBD symptoms [126]. REM sleep without atonia and RBD have been variably reported in patients with progressive supranuclear palsy (PSP) and rarely in patients with corticobasal degeneration [127–130]. Evidence is contradictory on whether the amount of RSWA in PSP is similar to the amount seen in Parkinson disease [128, 130, 131]. Regardless, RBD rarely presents years in advance of PSP features, instead tending to occur concurrently with the motor dysfunction [5, 126, 130]. RBD has not been found in other primary tauopathies. Yet rare examples of autopsy-proven Alzheimer’s disease and PSP with RBD have been documented [5]. While it is possible that RBD can occur with a variety of the neurodegenerative proteinopathies, the frequency of RBD associated with synucleinopathies is far greater than in non-synucleinopathy disorders [5].
Narcolepsy and RBD
Since narcolepsy is a boundary state disorder, RSWA and RBD frequently co-occur in up to 60 % of cases [57]. Unlike RBD associated with synucleinopathy neurodegenerative disorders, RBD occurs equally in female as in male narcolepsy patients and has less complex and violent dream enactment behaviors which begin at an earlier age [57, 132]. RSWA without dream enactment occurs more frequently in patients with narcolepsy–cataplexy compared to both idiopathic hypersomnia patients and neurologically normal controls, correlating with the degree of hypocretin cell loss [57]. RSWA may be a useful diagnostic marker when unsure of a diagnosis between narcolepsy and idiopathic hypersomnia [133]. Antidepressants or stimulants may induce clinical RBD symptoms in narcolepsy patients, so sleep neurology clinicians must regularly inquire about dream enactment symptom occurrence and frequency in narcolepsy patients during follow-up care.
RBD Associated with Brainstem Lesions and Autoimmunity
Lesions of the brain stem caused by tumors, paraneoplastic, and autoimmune neurological disorders such as anti-Ma2-associated encephalitis or Morvan syndrome have been associated with clinical RBD symptoms and RSWA [13, 15, 16, 18, 19, 22–25, 128, 134, 135]. In addition, autoimmune disorders have been reported to occur in up to 20 % of women with RBD [29]. Brain stem lesions in patients with subsequent development of RBD symptoms have been helpful in localizing the structures responsible for control of REM sleep muscle tone in humans. One recent case identified RBD due to a discrete lesion in the right dorsomedial pons, the presumed location of the human sublateral dorsal nucleus/subcoeruleus that is believed to play a major role in generation of REM sleep muscle atonia [136]. Other lesional cases of RBD have also impugned the dorsal pontine tegmentum [26, 135]. Further case reports of brain stem lesions may allow insights into RBD pathophysiology. RBD or RSWA has also been found in up to 13 % of patients following traumatic brain injuries, indicating damage to brainstem structures responsible for REM sleep muscle control [137].
RBD Associated with Psychiatric Disorders and Antidepressants
There is growing evidence that RBD is common in psychiatric populations [28, 30, 51, 138]. Depression and antidepressant use are common, with as many as 60 % of RBD patients using antidepressant medications [30, 66]. In addition, RBD patients report significantly greater feelings of anxiety and depression when compared with matched control patients [107]. However, it remains unclear whether psychiatric RBD is a specific RBD subtype with a different pathophysiologic mechanism or simply an early manifestation of synucleinopathy neurodegeneration unveiled by antidepressant medications. A recent longitudinal study comparing iRBD patients taking antidepressant medications compared with antidepressant naïve iRBD patients showed a decreased rate of conversion to symptomatic RBD in the antidepressant users [139]. These findings, along with similar RSWA profiles between iRBD patients taking antidepressants and those not taking antidepressants, support the contention that psychiatric RBD is not a distinct RBD subtype, but simply iRBD that has been unveiled by antidepressant medication use [107, 139]. Notably, patients with depression have shown to be at an increased risk for developing PD, indicating that depression may be an early maker of PD [140, 141]. In addition, iRBD patients with depression appear to be at a significantly greater risk of future development of PD when compared to iRBD patients without depression [108]. PD patients with depression have been shown to have decreased norepinephrine binding in the locus coeruleus compared to PD patients without depression, providing evidence for a possible link between RBD and depression [142]. However, while there appears to be some relationship between psychiatric disease, RBD, and PD, idiopathic and symptomatic RBD patients have significantly greater tonic RSWA elevation when compared to patients with psychiatric RBD on antidepressant medications, indicating that there may be mechanistic differences between these RBD types [107]. Further research in this area is needed to understand these relationships.
Selective serotonin reuptake inhibitors (SSRI) and tricyclic antidepressants have been shown to exacerbate RBD symptoms and increase REM sleep muscle tone in patients without clinical dream enactment [29, 30, 138]. However, it remains unclear whether neurochemical effects mediated by antidepressants cause a reversible state of RSWA and RBD, or these drugs simply unveil RSWA and RBD in predisposed individuals [6, 29, 30]. Patients without a history of dream enactment on antidepressant medications have been reported to have increased RSWA when compared to normal volunteers not on antidepressants [107, 143]. In addition, limited evidence suggests that patients who previously received antidepressant medication use but who were not using antidepressants at the time of polysomnography had normal RSWA indices, suggesting that antidepressant medications do not cause a permanent alteration in REM sleep muscle tone [107]. Clinically, it is important to consider the impact of antidepressant effects in patients with RBD. Gradual cessation of SSRI medications or changing to an alternate antidepressant medication such as bupropion, which may have lesser propensity to aggravate RBD, may help decrease the frequency of dream enactment behaviors [144].
Pathophysiology of RBD
The mechanisms for RSWA and RBD remain poorly understood. Animal studies involving the rat and cat have informed much of our understanding of REM sleep atonia control. RBD-type behaviors were first demonstrated by selective lesioning of the cat locus coeruleus in 1965 [145]. Further study of sleep in the cat and rat has shown that the regulation of REM sleep is complex, and primarily located within the pons and medulla, especially the noradrenergic locus coeruleus (LC) and the cholinergic pedunculopontine nucleus (PPN) and laterodorsal tegmental nucleus (LDTN). Glutamatergic projections from the medullary magnocellular reticular formation (MCRF) also play a role in motor inhibition. In addition, the glycinergic/GABAeric nucleus raphe magnus, and the ventral and alpha gigantocellular nuclei have been implicated in the suppression of REM sleep muscle tone [146]. The hypothalamus, thalamus, substantia nigra, basal forebrain, and frontal cortex also participate in REM sleep regulation [145, 147].
Brainstem regions thought to be involved in the pathophysiology of RSWA and RBD are the MCRF, locus coeruleus/subcoeruleus (LC/SC) complex, PPN, LDTN, and the substantia nigra [145, 148]. Lesions in the LC/SC cause RSWA, and the site and severity of the lesion determines whether simple or complex behaviors result [3]. However, the rat sublateral dorsal nucleus (SLD), analogous to the cat SC, appears to have a vital role in the regulation of normal REM atonia, and when lesioned, mediating the pathophysiology of RSWA and RBD [149–153]. The SLD is located in the dorsal pontine tegmentum [152]. Lesions in the SLD result in RSWA in rats. The SLD contains primarily excitatory glutamatergic neurons with both caudal and rostral projections [153]. Rostral projections excite thalamocortical neurons, the basal forebrain, and lateral hypothalamus to generate REM sleep (EEG) [153]. Caudal projections to the ventromedial medulla (VMM), the premotor glycinergic/GABAergic neurons of the nucleus raphe magnus, and the ventral and lateral gigantocellular nuclei are responsible for activating hyperpolarization within spinal motorneurons and subsequent generation of REM sleep muscle atonia [153]. Rats also possess a “REM-on” and “REM-off” region [154]. The REM-on region is inhibited by GABAergic and galaninergic projections from the forebrain ventrolateral preoptic nucleus (VLPO), in addition to cholinergic projections from the PPN/LDTN which are active during wakefulness and non-REM sleep [153, 155]. The REM-off nuclei are activated by projections from the noradrenergic LC, serotonergic raphe nucleus (RN) and by hypocretinergic pathways from the lateral hypothalamus [3]. The REM-on nuclei in the SLD and the precoeruleus (PC) also interact with REM-off nuclei and are mutually inhibitory [154].
Recent research from a RBD transgenic mouse model has illustrated the importance of ionotropic glycine/GABAA, and metabotropic GABAB receptors for generation and maintenance of REM sleep muscle atonia [151]. Only simultaneous inhibition of both ionotropic and metabotropic receptors results in heightened REM sleep muscle tone similar to that of wakefulness [151]. Inhibition of only single neurotransmitter receptors results in incomplete muscle activation, and it is very likely that the large degree of variation of RSWA seen between different patients, and within the same patients on repeated studies over different nights, is a direct result of how many receptors are inhibited [151, 156]. While large lesions to the SLD result in RSWA, they also cause a large decrease in total REM sleep time [153, 154]. Therefore, because RBD patients often have a normal amount of REM sleep, it is unlikely that RBD and RSWA in iRBD result from lesions directly to the SLD [150, 153]. Two populations of SLD/SC neurons have been found in the cat brain; ascending glutamatergic projections responsible for generation of REM sleep EEG activity, and descending glutamatergic projections inducing REM sleep muscle atonia [153]. Possibly only the descending projections are disrupted in iRBD, resulting in RSWA and dream enactment, while the ascending projections are relatively spared [153]. Another possible hypothesis is that the selective degeneration of premotor REM-on GABA/glycinergic neurons of the nucleus raphe magnus and the ventral and alpha gigantocellular reticular nuclei occur in RBD as a result of alpha-synuclein aggregation, which is also in accordance with the Braak hypothesis [157]. Both noradrenergic and serotonergic influences also play a role in REM sleep muscle tone. Decreased serotonin and noradrenaline concentrations have been found in the nuclei of hypoglossal motor neurons, with a subsequent increase in REM muscle tone with direct injection of serotonin into these nuclei [153, 158]. On the other hand, noradrenaline does not appear to directly increase muscle tone, but instead amplifies glutamatergic driven muscle excitation [159]. Dream enactment behavior and phasic muscle twitches during REM sleep are hypothesized to result from direct or indirect glutamatergic inputs from the motor cortex projecting to spinal motor neurons [153]. The fact that movements during DEBs in patients with PD appear faster and less stereotyped than movements during wakefulness argues for direct activation of spinal motor neurons resulting from corticospinal and corticofugal projections bypassing the smoothing contributions of the basal ganglia during REM sleep [153, 160]. Ultimately, REM sleep muscle atonia likely results from a powerful combined glycinergic/GABAergic drive stimulated by glutamatergic projections from the SLD, with additional mediation by seratonergic and noradrengergic influences [153].
Since most of our understanding of REM sleep comes from animal models, there are very likely interspecies differences between humans and animals. However, lesional cases of RBD in humans also propose that human dorsomedial pontine structures analogous to the SLD and MCRF play similar roles to the animal models. Discrete dorsomedial pontine lesions in SLD-analogous structure lead to decreased motor inhibition, as well as decreased excitation of the MCRF, thereby potentially causing RBD symptoms [136]. It remains unclear whether lesions causing RBD in humans may involve the MCRF alone, or whether lesions must have additional involvement of the REM-off regions [154].
Because patients with narcolepsy frequently have RBD, the relationship between orexin-A and orexin-B (hypocretin-1 and hypocretin-2) and REM sleep muscle tone has also been examined. Decreased levels of CSF hypocretin-1 from damage to the lateral hypothalamus have been associated with a greater amount of muscle activation during REM and non-REM sleep in patients with narcolepsy with and without cataplexy [161]. Hypocretin has also been shown to play a role in REM sleep muscle activation by directly acting on LC neurons, in addition to REM sleep muscle tone suppression by action on the pontine inhibitory area [162, 163], suggesting that hypocretin likely plays a large role in the stabilization of REM sleep muscle control, and that disruptions in hypocretin expression, whether deficient or excessive, could result in RSWA [161, 162, 163]. Decreased hypocretin levels have been associated with RSWA in narcolepsy, while increased hypocretin levels have been associated with RSWA in PD [161, 164]. In addition, in a small case study of patients with idiopathic RBD, hypocretin levels were normal, so further research on the role of hypocretin in mediating RSWA and RBD is needed [165]. Dopamine may also play a stabilizing role in the control of REM sleep muscle tone, with both dopamine deficiency and excessive levels of dopamine resulting in increased REM muscle tone [166, 167]. Because RSWA is occasionally seen in PD patients without RBD, it is possible that dopaminergic dysfunction in these patients contributes to the RSWA without DEB [55]. Further exploration of this relationship is needed.
The Braak Staging Hypothesis and RBD
Because of the strong association of RBD with PD, the Braak staging hypothesis of PD may provide a possible explanation for the occurrence of RSWA and RBD symptoms in PD and perhaps also in DLB [3, 5]. Braak has postulated six stages of progressive and selective Lewy body deposition and Lewy neurite accumulation based on the clinical phenotype of PD, corroborated by pathologic examination [157]. Each successive stage has increased Lewy bodies and Lewy neurites compared to the previous stage. In addition, some structures are almost never affected in the six stages, while others are affected in most, indicating a selective vulnerability in the synucleinopathy neurodegeneration [168]. In stage 1, Lewy bodies and neurites begin to accumulate in the dorsal motor nucleus of the vagus in the medulla, while in stage 2, Lewy bodies and neurite progress rostrally through the magnocellularis reticular nucleus, subceruleus–ceruleus complex, olfactory bulb, and anterior olfactory nucleus. RBD symptoms are also considered to be part of stage 2, given common impairments in olfaction and cardiac denervation reported in idiopathic RBD patients [83]. Stages 1 and 2 are considered the “preclinical” phase of parkinsonism, preceding the evolution of motor or cognitive symptoms, in which RBD is the most distinguishing feature [168]. The parkinsonian stage is encompassed by stages 3 and 4 with the progression of Lewy deposits to the substantia nigra, the pedunculopontine nucleus, and the amygdala occurring in stage 3, while stage 4 results from progression of Lewy deposits into the temporal mesocortex. Widespread neurodegeneration occurs in stages 5 and 6, with Lewy deposits affecting the neocortex, causing cognitive impairment [157]. Further evidence for the relationship between RBD and Braak staging stems from reportedly impaired olfaction and dysautonomia in patients with idiopathic RBD, indicating that RBD may be a precursor of PD [81–83, 94, 103]. As PD progresses from stage 1 to stage 6, there is an increasing loss of hypocretin cells, possibly supporting the hypothesis that hypocretin deficiency either plays a role in RBD pathology, or occurs concurrently and causes other PD-associated features [169]. However, a recent small case series reported increased levels of RSWA associated with increased hypocretin, so this relationship remains incompletely understood [164]. In addition, Braak staging does not well explain why some Lewy body disease patients never display RBD symptomatology, nor why some RBD patients never develop PD.
Treatment of RBD
Suppression of nightmares and prevention of patient and bed partner from injuries are the main goals of RBD treatment. Prediction of injury occurrence in RBD is very difficult. Patients with idiopathic RBD and those who remember their dreams have been recently found to be at significantly greater risk of injury [41]. However, frequency of dream enactment behaviors has not been shown to be predictive of possible injury, highlighting the importance of RBD treatment, even in patients with infrequent episodes of dream enactment [41]. All dangerous objects should be removed from the bedroom. Moving furniture away from the bed, padding the corners of bedside tables, and placing a mattress next to the bed may help prevent injury if the patient falls or leaves the bed during dream enactment [44]. If injury to the bed partner occurs, sleeping in separate beds or separate rooms minimizes risk of injury. Bed rails or barriers between the patient and bed partner may also be utilized, and bed alarms may be useful if the bedpartner has had to move to another room. A novel bed alarm designed to provide RBD patients with a calming instruction to return to sleep has been effective in reducing DEB in medication refractory patients [170]. The alarm system includes a pressure sensitive pad underneath the shoulders of the patient, as well as a clothing tether that is attached magnetically to the alarm system that detaches from the alarm system, activating the alarm and waking the patient from sleep, thereby averting the RBD episode [170]. The device is most effective in patients with a history of leaving the bed during dream enactment behavior. Confusional arousals resulting from obstructive sleep apnea, a common comorbidity in RBD patients, may mimic RBD episodes [171]. Treatment of apnea with continuous positive airway pressure may result in improvement in the frequency and severity of these episodes; however, treatment of OSA has not been shown to decrease RSWA, indicating there may be no direct effect of treatment of comorbid OSA on the pathophysiology of RBD [54].
Clonazepam and Other GABA Agonists
Melatonin and clonazepam are considered to be the two mainstays of pharmacologic treatment for RBD [32, 66, 68, 172, 173]. Melatonin and clonazepam appear to be equally effective in reducing frequency and severity of DEB, but only melatonin has been reported to significantly reduce injuries, with fewer side effects than clonazepam [66]. The GABAA receptor modulator clonazepam was the initial treatment reported as effective for decreasing RBD symptoms, with a median effective dose of 0.5 mg and a range of 0.25–2.0 mg given approximately 30 min before bedtime [8, 28, 59, 66–68, 75, 108]. Of note, while patients on clonazepam have decreased motor activity during REM, REM sleep muscle atonia is not restored, indicating that clonazepam may act on the motor cortex to inhibit glutamate induced phasic muscle bursts, but not on regions responsible for control of REM sleep muscle atonia [153, 174–176]. While clonazepam effectively reduces RBD symptoms, it can also increase the severity of obstructive sleep apnea and cognitive impairment. Additional side effects include sleepiness, dizziness, unsteadiness, and sexual dysfunction [32, 66, 176]. Therefore, patients with cognitive impairment, dementia, and sleep apnea must be monitored carefully when treated with clonazepam. While most patients with RBD associated with neurodegenerative disorders tolerate clonazepam well and benefit from its use, melatonin has been shown to be more effective in treating this population with fewer side effects [66]. Other GABA receptor modulators may also be useful in treating RBD; however, very little research on the effectiveness of these drugs on RBD symptoms exists [177]. Zopiclone has been reported to be effective in reducing RBD symptoms in a small number of patients [35, 177]. Zopiclone is a selective GABA α-1 and α-5 agonist with a short half-life and may impact the pathophysiology of RBD and reduce REM muscle tone by selectively acting on GABAA receptors [35]. However, further research is needed to validate this hypothesis.
Melatonin
Melatonin has recently been shown to be equally effective for RBD treatment, with fewer side effects than clonazepam [32, 66, 172, 173]. Adverse effects are dose related and primarily include daytime sleepiness; however, headache and hallucinations have been reported [32, 66, 178]. Melatonin may be a more efficacious treatment for patients with symptomatic RBD due to its more tolerable side effect profile [66]. The median effective dose of melatonin has been reported to be 6 mg at bedtime [66]. However, ranges of 3–25 mg have also been reported as tolerable and effective [66]. Melatonin has been shown to increase REM sleep atonia during treatment; however, the mechanism by which this occurs remains unknown [35, 172, 173].
Other Potential Treatments of RBD
Other treatments of RBD have been limited to case reports and small case series. The association between RBD and PD has prompted interest in the role of dopamine in the pathophysiology of RBD. While levodopa decreases the amount of REM sleep time, possibly providing less opportunity for dream enactment behaviors, high doses of levodopa have been shown to increase both REM muscle tone and hallucinations which may be mistaken for RBD [166, 179]. Pramipexole has shown mixed results, varying from little or no effect, to reduction of RBD episodes [180–182]. In a recent large series, 62 % of patients treated with pramipexole monotherapy reported improvement in RBD symptoms, compared to 88 % improvement reported by patients treated with clonazepam, suggesting that pramipexole is likely not a suitable first-line therapy for RBD [183]. In this study, pramipexole was more effective in treating patients with lower amounts of RSWA compared to patients with significant amounts of RSWA, indicating that dopaminergic agents may help stabilize control of REM muscle tone early in the early course of PD [183]. Zonisamide, which is used to treat motor symptoms of PD, has been reported to decrease DEB in one PD patient [184]. Zonisamide has been shown to increase GABA-ergic neurotransmission and decrease glutamate in the rat brain, indicating that zonisamide could influence RBD symptoms both by inhibition of glutamatergic phasic muscle bursts as well as through GABA induced hyperpolarization of motor neurons [185, 186]. Given the high frequency of RBD in PD, further research on the effects of zonisamide on RBD symptoms is warranted. If efficacious in treatment of RBD symptoms, zonisamide may allow for the use of one treatment for both motor dysfunction and RBD symptoms, eliminating the need for polytherapy in a cognitively vulnerable patient population that may be impacted more severely by polypharmacy. Donepezil has also rarely been reported to reduce motor events related to RBD [187, 188]. Agomelatine is a novel antidepressant with a melatoninergic mechanism that has been reported to effectively decrease RBD symptoms with minimal side effects [189]. In addition, RSWA decreased in patients taking agomelatine, suggesting a relatively potent melatoninergic effect [189]. Because agomelatine has also shown similar efficacy to other antidepressant medications with fewer side effects, agomelatine may be considered a very useful medication in RBD patients with depression [190]. In addition, the herbal supplement Yi-Gan San has been shown to suppress RBD symptoms in three patients, possibly by acting on serotonergic and GABAergic systems [191]. While clonazepam and melatonin are effective in reducing RBD symptoms, only rarely do these or other treatments completely eliminate potentially injurious dream enactment behaviors. Therefore, future large, prospective comparative treatment trials are needed to develop better and more tolerable treatments that completely eliminate injury potential (Table 49.2).
Table 49.2
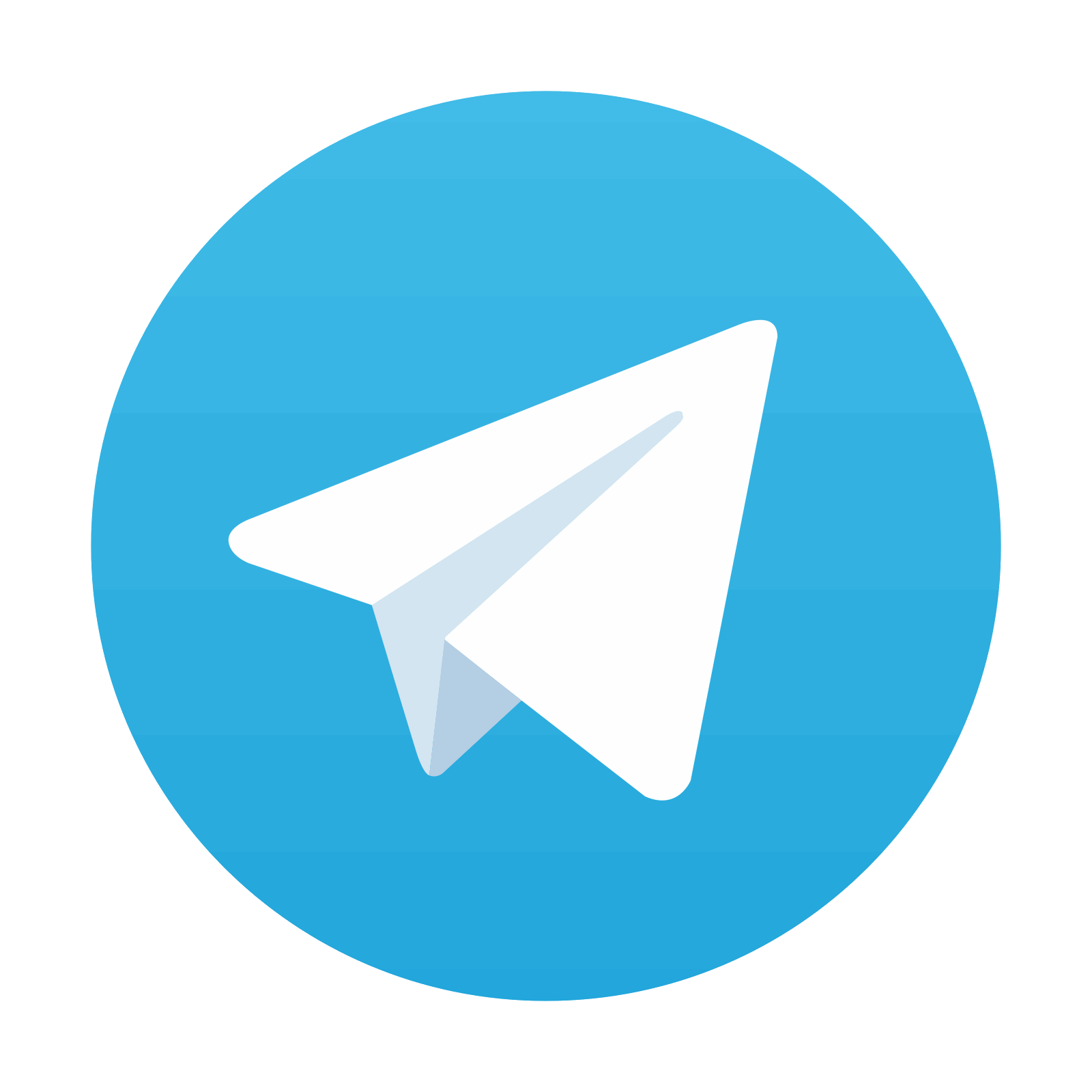
Treatments for RBD
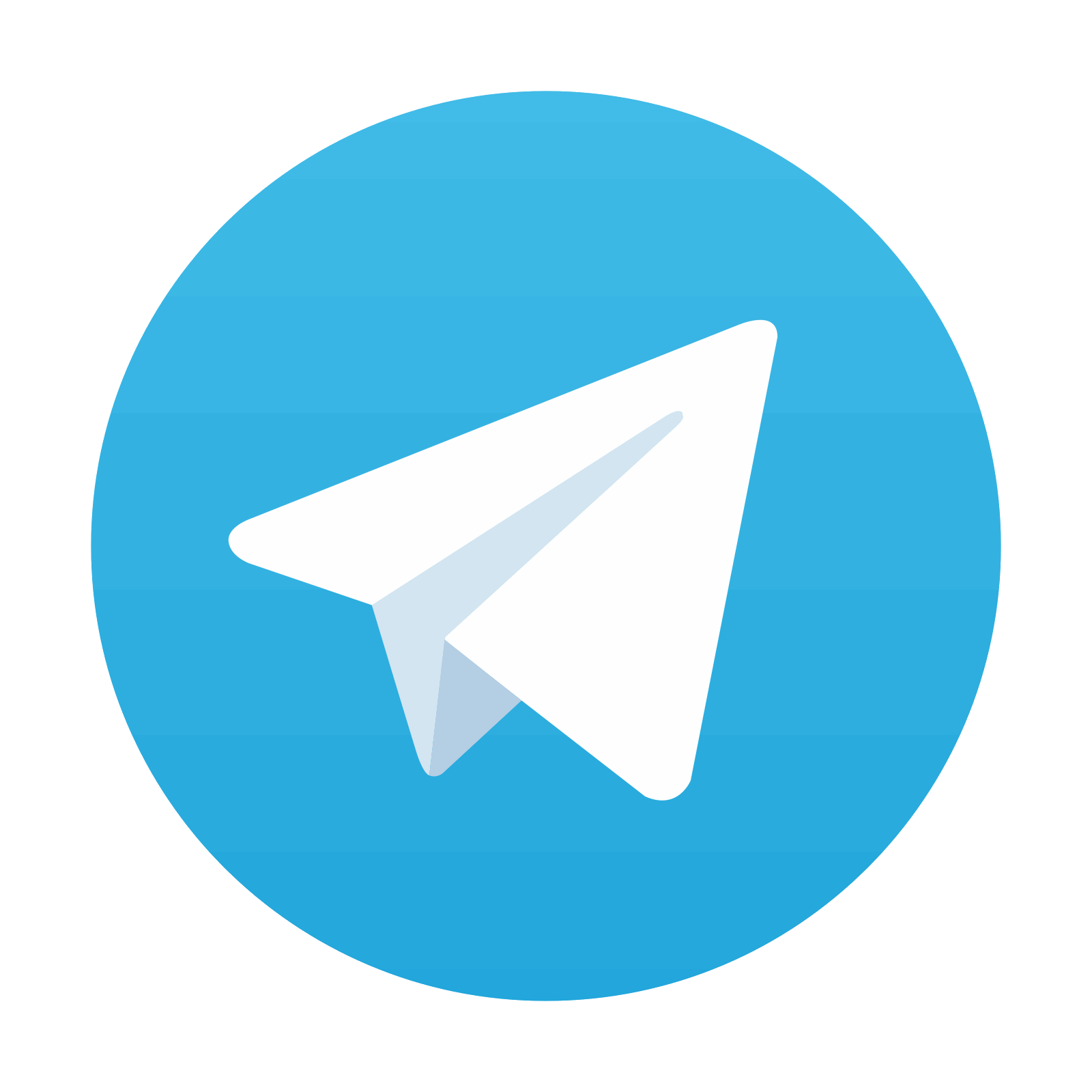
Stay updated, free articles. Join our Telegram channel

Full access? Get Clinical Tree
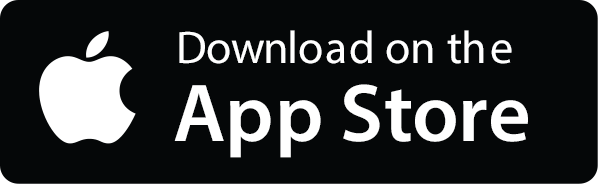
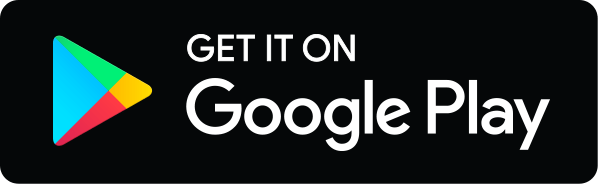
