Rational Approach to Brain Imaging and Electrophysiology
Robin A. Hurley
Nash N. Boutros
Katherine H. Taber
Background
The first step in the evaluation of a patient with a neuropsychiatric disorder is a thorough history and physical examination. After gathering this information, the clinician can make an informed decision if brain imaging or electrophysiology is warranted in the assessment process. These diagnostics may improve the care of patients with neuropsychiatric disorders. The information obtained from such studies may help clarify diagnosis, estimate prognosis, or improve the treatment plan. In this chapter, we discuss principles of test ordering in the clinical setting, review the main brain imaging and electrophysiologic modalities, and provide case examples of their application in neuropsychiatry.
Principles of Ordering and Interpretation
Brain Imaging
Brain imaging can contribute information for diagnosis, prognosis, and treatment planning, as noted in the preceding text. The research into each of these contributions is limited not only by the complexities of functional anatomy, but also by our ability to design studies that would separate out these contributions. As a diagnostic aid, imaging can help identify pathologies that produce clinical symptoms. This, in turn, informs both prognosis and treatment planning. These are interdependent factors in formulating patient management.
In general, it is employed to confirm the presence of focal brain pathology in a patient with findings from the history or examination which suggest such pathology. Patients with focal sensory or motor changes referable to the brain, or with obvious brain disease (stroke, epilepsy) or injury, are the traditional domains of
neurologists. In these patients the indications for brain imaging are reasonably clear and straightforward. The importance of imaging in patients with dementia or significant cognitive change is also well established. Imaging of the cognitively impaired patient may assist in the differential diagnosis of Alzheimer disease (AD), multi-infarct dementia, or cognitive decline due to another cause.1
neurologists. In these patients the indications for brain imaging are reasonably clear and straightforward. The importance of imaging in patients with dementia or significant cognitive change is also well established. Imaging of the cognitively impaired patient may assist in the differential diagnosis of Alzheimer disease (AD), multi-infarct dementia, or cognitive decline due to another cause.1
More challenging are those patients presenting with behavioral, emotional, or cognitive changes, the domain of neuropsychiatrists. Findings suggestive of focal brain pathology in these patients include atypical symptoms or symptom presentations, and focal deficits on neuropsychiatric examination (see Table 18.1). Specific examples of such indications include subsyndromal cognitive deficits, unusual age at symptom onset, unusual symptom evolution, personality changes, accompanying neurologic signs/symptoms, unusual symptoms (symptoms outside clinical norms), and sustained confusion/delirium.2 In addition, neuroimaging is recommended if there is a history of poison or toxin exposure including severe alcohol abuse with significant cognitive/social impairments, or brain injury (traumatic or “organic”).
TABLE 18.1 Clinical Indications for Imaging in Psychiatric Patients | |
---|---|
|
Erhart et al. found that treatment was changed in 15% of nondemented patients because of magnetic resonance imaging (MRI) findings.2 As an example, neuroimaging for differential diagnostics may clarify directions for treatment of cognitive impairment. Acetylcholinesterase inhibitors are helpful in the management of early stage cognitive impairment. Finding brain lesions can alert the clinician to avoid psychotropic agents suspected to slow neuronal repair (e.g., typical neuroleptics) or those agents documented to increase confusion in the patient with brain injury (e.g., lithium or strongly anticholinergic agents).3 If periventricular white matter abnormalities are present on imaging, then small
vessel hypertensive disease (poorly controlled blood pressure) may be present. A more aggressive control of blood pressure is then indicated.
vessel hypertensive disease (poorly controlled blood pressure) may be present. A more aggressive control of blood pressure is then indicated.
Electrophysiology
Unusual presentations and atypical age of onset are the main “red flags” indicating the need for more extensive investigations. Inui et al. emphasized the atypicality of the clinical presentation as the most important factor for initiating an electroencephalograph (EEG) evaluation.4 They found the frequency of epileptiform discharges (including controversial waveforms) to be significantly higher among patients with mood-incongruent psychotic mood disorder (33%) and schizophreniform disorder (30%) as compared with nonpsychotic mood disorder (3.2%) and schizophrenia (0%).
Brain Imaging and Electrophysiologic Modalities
Definitions
Tomography is the creation of a two-dimensional (2-D) image or section (from the Greek tomos [a cutting or slice] and grapho [to write]).
Computed tomography (CT), computer-assisted tomography uses computer processing to assemble information from multiple x-ray images into a 2-D cross-sectional image.
Spatial resolution determines the level of detail that can be seen in a two- or three-dimensional (2- or 3-D) image. It is defined by the number of pixels (picture elements) the image contains in each dimension and their individual size. A related concept is voxel (volume element).
Slice thickness is the width of tissue included in each image.
Signal-to-noise is the ratio of the desired information (signal) to the accompanying background activity (noise).
Plane-of-section describes the orientation of a cross-sectional image. The coronal plane divides the body into front (anterior) and back (posterior) portions. The sagittal plane divides the body into right and left portions. The axial or horizontal plane divides the body into upper and lower portions.
A contrast agent is any substance that is introduced into the body to make an anatomic structure more visible on images.
Background activity is the dominant ongoing EEG rhythm. The term usually refers to the background activity during resting wakefulness. Under normal conditions, this activity is dominated by alpha rhythms. This activity is characterized by waxing and waning and readily attenuates when the person open his/her eyes or begins to concentrate.
Beta, alpha, theta, and delta are the traditional EEG frequency bands. Recent advances in EEG analysis have blurred the boundaries between these traditional ranges—beta (>13.5 Hz), alpha (8.5 to 13.5 Hz) (Fig. 18.5B), theta (4 to 8 Hz) (Fig. 18.5C), delta (0.5 to 3.5 Hz).
Spike-and-sharp waves are paroxysmal (i.e., occur suddenly and are distinct from the ongoing background activity) events and usually are indicative
of an epileptic process. The difference between a spike and a sharp wave is a matter of the duration of the discharge. Spikes are <70 ms in duration and sharp waves are between 70 and 200 ms in duration. This difference could be a reflection of the depth of the focus from the surface. The term spike-and-wave complex refers only to the presence of a prominent wave after each spike, likely reflecting an inhibitory process after the excitatory spike (Fig. 18.5D).
Structural Brain Imaging
Computed Tomography
CT uses photons and a collecting detector to record tissue density. On standard CT, air (the least dense) is black and bone (the most dense) is white. All other tissues are a shade of gray (see Table 18.2). Conventional CT uses several rings of detectors to acquire several images simultaneously. A computer translates the detections into a 2-D picture. This is either displayed on a computer monitor or printed onto x-ray film. Radiation exposure is clinically insignificant (approximately 5 rads). For extra protection, scans are angled to avoid the lens of the eye. If urgently needed in pregnancy, lead aprons are worn. The recent introduction of spiral CT further reduces the radiation exposure, and provides true 3-D imaging. An advantage of 3-D imaging is that no areas are missed between slices.
Modern CT scanners can generate brain images that range from 0.5 to 10 mm in thickness, with 3 to 5 mm used most commonly. The slice thickness of a CT image is an important variable in clinical scanning. Thinner slices allow visualization of smaller lesions. However, the thinnest sections have less contrast (i.e., the signal intensity difference between gray and white matter is less) because the signal/noise is lower. It also takes longer to complete the examination because more slices must be acquired. Therefore, there is more chance of patient movement degrading the images. The longer scan time also decreases the number of patients that can be examined in a day. Thicker sections (or slices) have greater contrast but smaller lesions may be missed. There is also greater artifact due to increased volume averaging. This is particularly true in the base of the skull and may obscure brain stem and mesial temporal structures.
TABLE 18.2 Relative Image Intensity on Computed Tomography (Noncontrast) | ||||||||||||||
---|---|---|---|---|---|---|---|---|---|---|---|---|---|---|
|
Magnetic Resonance Imaging
MRI is based on manipulating the small magnetic field around the nucleus of the hydrogen atom (proton), a major component of water in soft tissue. To take an MRI of a patient’s soft tissues, the patient must be placed inside a large magnet. The strength of the magnet is measured in tesla (T). Most clinical systems have a field strength of 1.5 T, although 3.0 T systems are becoming more available for clinical work. (More powerful systems are often used in research settings.) A
midfield system is generally 0.5 T, and low-field units range from 0.1 to 0.5 T. The greater signal available with 1.5 and 3.0 T systems allows higher resolution images to be collected. However, this increased detail is costly because high-field systems are more expensive than mid- or lowfield systems. Also, many patients feel uncomfortable while lying inside these huge enclosing magnets (see Fig. 18.1A). Open design magnets are now available that provide less feeling of confinement to the patient (Fig. 18.1B).
midfield system is generally 0.5 T, and low-field units range from 0.1 to 0.5 T. The greater signal available with 1.5 and 3.0 T systems allows higher resolution images to be collected. However, this increased detail is costly because high-field systems are more expensive than mid- or lowfield systems. Also, many patients feel uncomfortable while lying inside these huge enclosing magnets (see Fig. 18.1A). Open design magnets are now available that provide less feeling of confinement to the patient (Fig. 18.1B).
To create an MRI, the patient’s hydrogen atoms are exposed to a carefully calculated series of radio frequency (RF) pulses while the patient is within the scanner’s magnetic field. These RF pulses change the magnetization of the hydrogen atoms, generating tiny electric signals that are picked up by a receiver placed close to the area being scanned (the imaging coil). A head coil that encloses the head, allowing maximum signal pickup, is used to obtain brain images. The magnetic field gradients needed to acquire the image are created by huge coils of wire embedded in the magnet, driven with large current audio amplifiers similar to those used for musical concerts. These can create a great deal of noise during the scan, and may distress the unprepared patient.
In MRI there are a wide variety of image types possible, each sensitive to a different physical aspect of tissue. This is quite different from CT, where the images reflect a single parameter, the density of tissue. The pulse sequence used to acquire the MRI (the combination of RF and magnetic field pulses used by the computer to create the image) determines the unique information the image will contain. Clinical MRI most commonly uses the spin echo (SE) or the fast spin echo (FSE) sequences. The expected appearances of tissues using the most common SE imaging methods are summarized in Table 18.3. T1-weighted images are traditionally considered best for displaying anatomy, whereas T2-weighted images are best for displaying pathology. However, both pathology and cerebrospinal fluid (CSF) will appear bright, making it difficult to visualize pathology near the ventricles. A variation on the T2-weighted scan has been developed (called fluid attenuated inversion recovery [FLAIR]) where CSF is dark, making pathology near CSF-filled spaces much easier to see. FLAIR MRI is extremely useful in neuropsychiatry (see Fig. 18.2).
Two other methods that are useful in clinical imaging are gradient echo (GE) or gradient refocused echo (GRE) and diffusion-weighted (DW) MRI. GE imaging (also called susceptibility weighted imaging) is very sensitive to anything in the tissue causing magnetic field inhomogeneity, such as hemorrhage or calcium. These images have artifacts at the interfaces between tissues with very different magnetic susceptibility, such as bone and brain. The artifacts at the skull base are sometimes severe. DW MRI is sensitive to the speed of water diffusion, and may be able to visualize areas of ischemic stroke in the critical first few hours after onset. It is also showing potential in the imaging of other conditions, including neurodegenerative conditions and traumatic brain injury (TBI).
Computed Tomography Versus Magnetic Resonance Imaging
CT is more widely available and is less expensive than MRI (see Table 18.4). Several types of pathology, particularly calcification, acute hemorrhage, and bone injuries, are better imaged with CT. Otherwise, MRI is preferred because it has a much higher resolution, can provide images in any plane of section, does not have artifacts near bone, and is sensitive to more types of pathology. CT has limited contraindications surrounding contrast agent administration: Allergy to iodine,
contrast dyes, or shellfish, creatinine ≥1.5 mg per dL, or metformin administration on the day of contrast scan. Contraindications to MRI include magnetic metals in the body, history of welding without screening x-rays, or implanted electrical, mechanical, or magnetic devices.
contrast dyes, or shellfish, creatinine ≥1.5 mg per dL, or metformin administration on the day of contrast scan. Contraindications to MRI include magnetic metals in the body, history of welding without screening x-rays, or implanted electrical, mechanical, or magnetic devices.
TABLE 18.3 Relative Image Intensity on Magnetic Resonance Imaging (Noncontrast) | |||||||||||||||||||||||||||||||||||||||||||
---|---|---|---|---|---|---|---|---|---|---|---|---|---|---|---|---|---|---|---|---|---|---|---|---|---|---|---|---|---|---|---|---|---|---|---|---|---|---|---|---|---|---|---|
|
Contrast-Enhanced Structural Imaging
Structural brain images can be acquired either with or without intravenous administration of a contrast agent. These intravascular agents normally do not
penetrate into the brain, as they cannot pass through an intact blood–brain barrier (BBB). If this barrier is disrupted, the contrast agent leaks into surrounding tissue and changes its appearance on imaging. Conditions where the BBB may open include tumors, inflammatory or autoimmune conditions such as lupus or multiple sclerosis, and infectious processes. Other conditions where a contrast agent is useful include evaluation of suspected aneurysms, arteriovenous malformations, or other vascular processes (e.g., temporal arteritis).5,6,7
penetrate into the brain, as they cannot pass through an intact blood–brain barrier (BBB). If this barrier is disrupted, the contrast agent leaks into surrounding tissue and changes its appearance on imaging. Conditions where the BBB may open include tumors, inflammatory or autoimmune conditions such as lupus or multiple sclerosis, and infectious processes. Other conditions where a contrast agent is useful include evaluation of suspected aneurysms, arteriovenous malformations, or other vascular processes (e.g., temporal arteritis).5,6,7
TABLE 18.4 Factors to Consider When Choosing an Imaging Modality | |||||||||||||||||||||||||||||||||||||||||||||
---|---|---|---|---|---|---|---|---|---|---|---|---|---|---|---|---|---|---|---|---|---|---|---|---|---|---|---|---|---|---|---|---|---|---|---|---|---|---|---|---|---|---|---|---|---|
|
Ordering the Examination
The neuroradiologist needs very clear clinical information on the imaging request form (not just “rule out pathology” or “new-onset mental status changes”). If a lesion is suspected in a particular location, the neuroradiologist should be informed of this or give enough clinical data for selection of the best imaging method and parameters to view suspicious areas. The neuroradiologist and technical staff also need information on the patient’s current condition (e.g., delirious, psychotic, easily agitated, or paranoid). This may eliminate difficulties with patient management during the scan.
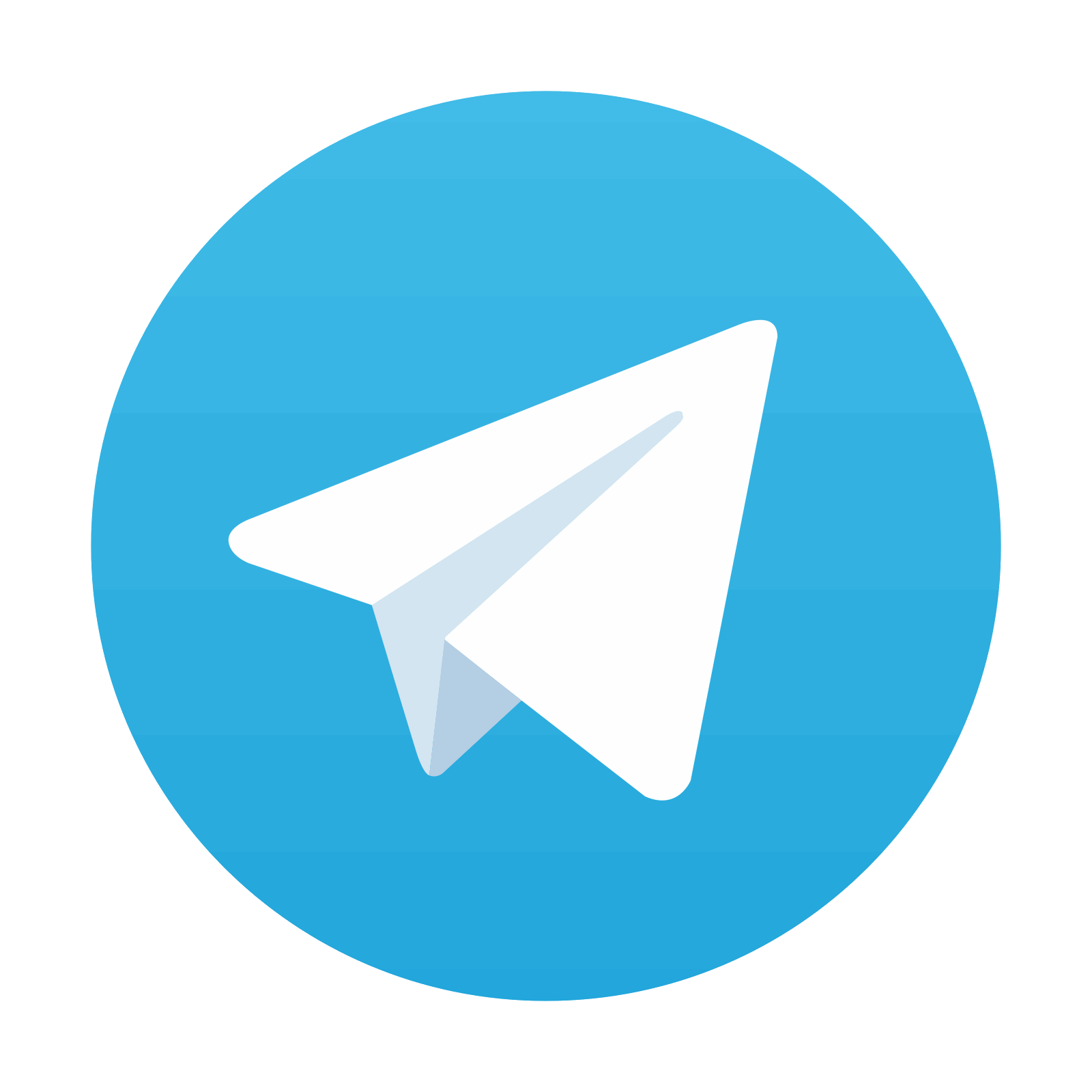
Stay updated, free articles. Join our Telegram channel

Full access? Get Clinical Tree
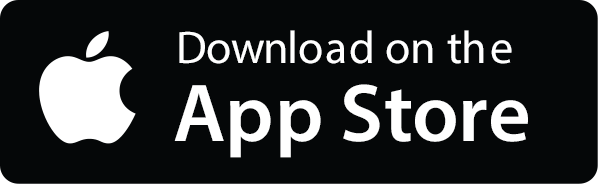
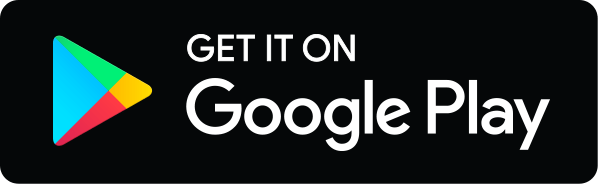
