Receptors and Signaling the Nucleus
INTRODUCTION
In this chapter, we continue a discussion about events at the cellular level and explore what happens at receptors after neurotransmitters make contact.
Electrochemical communication continues at the receptor on the postsynaptic membrane. Without a receptor, the neurotransmitter is like a tree falling in the woods with no one to hear it. The binding of the neurotransmitter with the receptor initiates a series of events that change the postsynaptic cell in some way. Receptors are protein units embedded in the lipid layer of the cell membrane. There are two basic types of receptors activated by neurotransmitters (more on this below), and the response generated depends on what type of receptor is engaged.
Agonists and Antagonists
Most neuropsychiatric medications work their magic by enhancing or limiting the effects of the neurotransmitter at the receptor. Figure 5.1 shows a schematic representation of a receptor, a neurotransmitter, and the opposing effects of contrasting medications. Pharmacologists and neuroscientists use the terms ligand, agonist, and antagonists, but in this book we also use the more self-explanatory terms such as transmitter, drug/medication, stimulate, and block.
FAST RECEPTORS: CHEMICAL
There are two basic types of receptors for neurotransmitters. The one we usually think of is shown in Figure 5.1—an ion channel (also called transmitter-gated ion channel). A neurotransmitter or medication stimulates the opening of the pore inside the receptor and ions rapidly flow into the cell. Receptors that allow the entry of positive ions such as Na+ or Ca2+ result in an excitatory postsynaptic potential (EPSP). Acetylcholine (ACh) and glutamate result in such activation and are considered excitatory.
Receptors permeable to negative ions, such as Cl-, will result in inhibitory postsynaptic potentials. γ-Aminobutyric acid (GABA) and glycine, both considered inhibitory, cause this kind of activation. The essential point regarding these transmitter-gated ion channels is that they are fast. These receptors are magnificent little machines that rapidly allow the entry of large currents with great precision. The ions pour into the cell and the signal from the proceeding neuron, whether excitatory or inhibitory, is quickly propagated along the membrane of the target cell. As we reviewed in Chapter 3, an action potential is only generated if enough EPSPs bring the resting potential of the postsynaptic cell above the threshold at the axon hillock.
Amino Acid Receptors
The amino acid receptors mediate most of the fast transmitter-gated channels in the brain. The two prominent ones are glutamate and GABA, which are reviewed in the subsequent text.
POINT OF INTEREST
The receptors, unlike the transmitter, come in a variety of styles—a relationship that is much like feet and shoes. You only have two feet, but many shoes. Serotonin is one example: there are 14 different receptor subtypes for this one neurotransmitter. Some receptors are categorized as different classes, whereas others are just different subtypes within a class. It is not entirely clear why some differences constitute a new class and others just warrant a new subtype. Most likely a committee decided.
Glutamate
There are three prominent glutamate receptors: N-methyl-d-aspartate (NMDA), α-amino-3-hydroxy-5-methyl-4-isoxazole propionate (AMPA), and kainate, each with several subtypes. They are named after the artificial agonist that selectively activates them. For example, NMDA activates the NMDA receptor, but not the AMPA or kainate receptors. NMDA and AMPA constitute the bulk of fast excitatory synaptic transmission in the brain. The role of kainate is not clearly understood.
Both NMDA and AMPA receptors, which often coexist on the same postsynaptic receptor, allow the rapid entry of Na+ into the cell (and the simultaneous exit of K+) that generates the depolarization of the postsynaptic cell. NMDA receptors are unique in that they also allow the entry of Ca2+ that can act as a second messenger inside the cell. This can have a profound impact on the cell resulting in lasting changes, as will be shown at the end of this chapter when we discuss long-term memory.
The NMDA receptor is further unique in that it requires both the glutamate transmitter and a change in the voltage to open before it will allow the entry of Na+ and Ca2+. This property is due to the presence of Mg2+ ions, which clog the NMDA receptor at resting voltage. Figure 5.2 shows how the AMPA receptor works in conjunction with
the NMDA receptor to depolarize the cell and bring Ca2+ into the cell. This property has a significant impact on the capacity of the neurons to change.
the NMDA receptor to depolarize the cell and bring Ca2+ into the cell. This property has a significant impact on the capacity of the neurons to change.
The glutamate receptor is increasingly being explored as a novel target for psychiatric conditions. Preliminary studies have shown that a single IV injection of ketamine (an NMDA antagonist) can rapidly and temporarily reduce depression. With schizophrenia, glutamate has long been suspected as a possible culprit, and Eli Lilly is conducting clinical trials with a medication that reduces glutamate release. Figure 15.3 shows the benefits of a partial NMDA agonist that, in conjunction with exposure therapy, reduces fear.
γ-Aminobutyric Acid
GABA and glycine are the primary inhibitory neurons in the brain; inhibition is a process that must be tightly regulated. Too much inhibition causes the brain to slow down—even lose consciousness. Not enough inhibition and the electrical activity can get out of control—evoke a seizure. GABA is the most common inhibitory receptor.
The GABA receptor is made up of five protein subunits, which vary for different subclasses of the receptor. The GABAA receptor (Figure 5.3) is the focus of much pharmacological interest. There are several other sites on the GABAA receptor where chemicals can modulate its function. For example, barbiturates and benzodiazepines have their own distinct sites on the GABA receptor. These medications by themselves do not open the GABA channel, but they can enhance the strength or frequency of the opening. Benzodiazepines plus GABA results in more Cl- entering the cell and a greater inhibitory effect.
![]() FIGURE 5.3 • The GABA receptor showing other drugs that can modify and enhance the inhibitory effect of these receptors. GABA, γ-aminobutyric acid. |
Ethanol is another popular drug that enhances the function of the GABA receptor. Long-term use of ethanol decreases the expression of the GABA receptor, which may explain the tolerance that develops with alcoholism. Whether the receptor alterations contribute to the propensity for seizures when the alcohol is withdrawn remains unclear.
The steroid hormones can also modulate GABA receptors (sometimes called neurosteroids when they have effects on neurons) (see Figure 6.1). This may explain the psychiatric symptoms that develop at times when the sex hormones are reduced, for example, premenstrual syndrome, menopause, and chemical castration for men. Additionally researchers have found that some steroid hormone levels drop in patients who panic during an attack. Others have shown that medications such as olanzapine and fluoxetine—known to decrease anxiety—increase steroid hormone levels.
SLOW RECEPTORS: METABOLIC
Starting in the 1950s, researchers teased out the details of a second type of receptor—one that activates a cascade of biochemical events in the cytosol of the receptor cell that ultimately modifies the function of target proteins or the DNA. This type of receptor, called a G-protein-coupled receptor, is perhaps even more relevant to the effect of psychiatric medications than the transmitter-gated ion channel. G-protein is the short form for guanosine triphosphate-binding protein. Although there are many types of G-protein-coupled receptors, the basic style involves three steps:
A neurotransmitter binds to the receptor.
The receptor activates the G-protein, which moves along the intracellular membrane.
The G-protein activates the “effector” protein.
The activated “effector” protein can have a variety of functions including just opening traditional ion channels (not shown). However, the more interesting effector proteins are enzymes that trigger a process called a secondary messenger cascade (Figure 5.4). The neurotransmitter activates the G-protein, which slides along the membrane and stimulates the effector protein. The activated effector protein then converts adenosine triphosphate into cyclic adenosine monophosphate (cAMP): the secondary messenger, which will diffuse away into the cytosol where it can change the neuronal operations. With the exception of serotonin type 3 receptor (5-hydroxytryptamine3 [5-HT3]), all
the monoamine receptors belong to the G-protein-coupled family—which means that most psychiatric medications deliver their punch through secondary messengers.
the monoamine receptors belong to the G-protein-coupled family—which means that most psychiatric medications deliver their punch through secondary messengers.
TREATMENT
ANTIEPILEPTIC DRUGS
The background about inhibitory and excitatory receptors helps one understand the major mechanisms of action of the antiepileptic drugs (AEDs). The goal of treatment with these medications is to modify the aberrant bursting properties, synchronization, and spread of abnormal firing without affecting ordinary electrical activity. These effects, although intended to control seizure disorders, have wide-ranging applications to other neuropsychiatric disorders such as bipolar affective disorder, anxiety, pain, and alcohol dependence, to name a few.
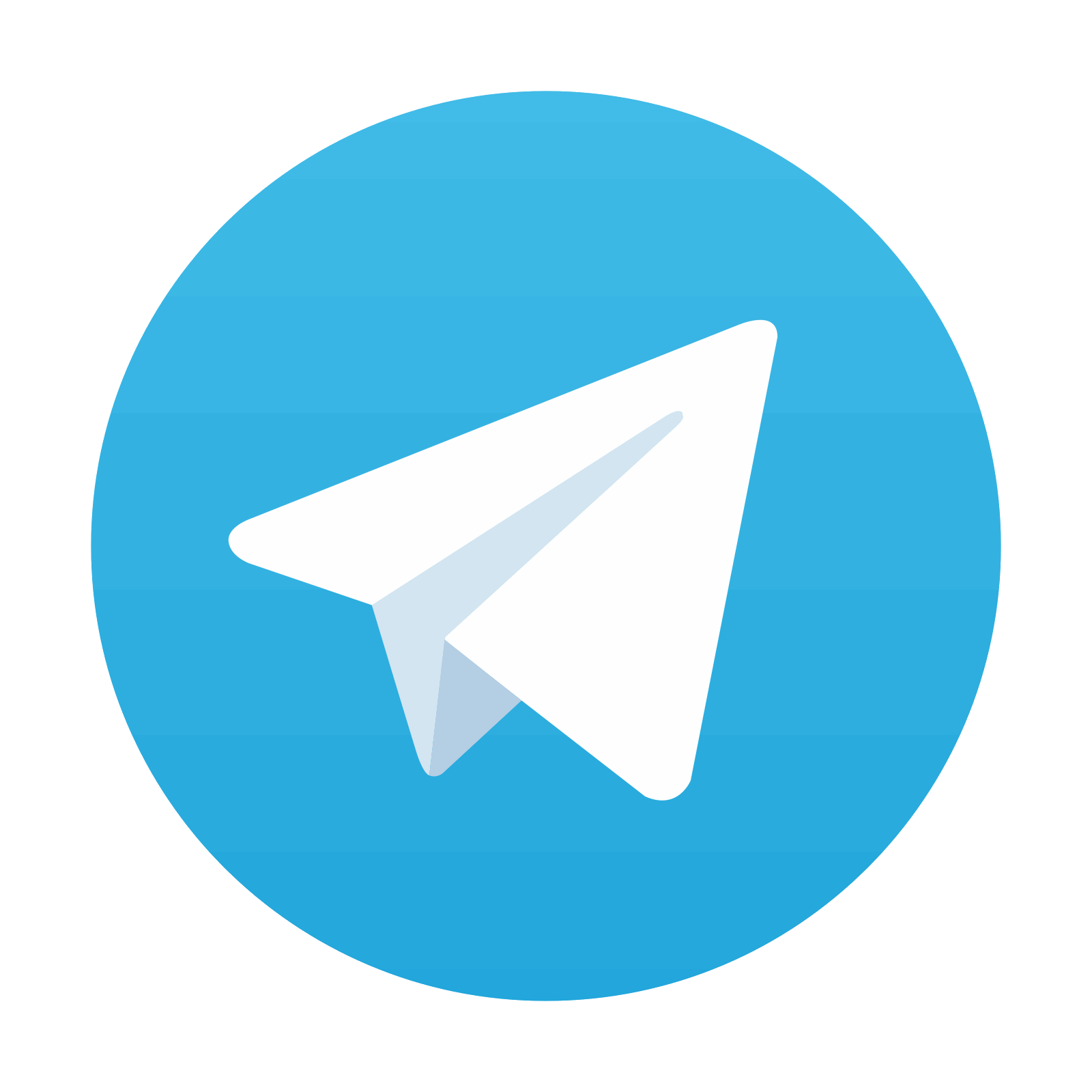
Stay updated, free articles. Join our Telegram channel

Full access? Get Clinical Tree
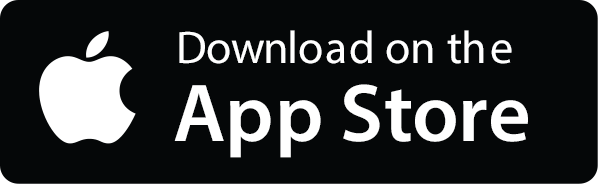
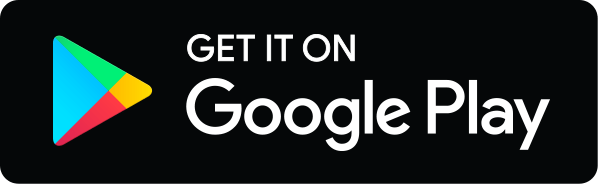
