Fig. 8.1
Two methodologies for intervention in spinal cord injury. There are molecular approaches (left) and electrophysiological approaches (right) for analyzing and biological processes after SCI and providing therapeutic intervention
The molecular and electrophysiological approaches both can be used for analysis of nervous system disorders and therapeutic intervention. The molecular approach is based on the knowledge of molecular cellular biology and aiming to modulate cellular functions in patients with SCI. For example, cell death modulation and apoptosis are closely related to the prevention of secondary injury, which is reported to be a main cause of progressive damage in patients with acute phase SCI. In the same manner, basic research on axonal elongation revealed the existence of inhibitory factors such as Nogo in the injured spinal cord, which acts on a group of receptors that induce the growth cone shrinkage leading to the termination of axonal regeneration. Now, this molecular mechanism is regarded as a functional recovery to induce axonal regeneration, and various pharmacological approaches based on this are now in the preclinical stages of investigation. Taken together, the molecular approach is thought to be an effective means of modifying the neural structure (hardware).
On the other hand, the electrophysiological approach is effective in measuring or modulating neural functions. Various noninvasive means such as electromyography, measurement of nerve conduction velocity, and transcranial magnetic stimulation are used to assess the connectivity of supraspinal circuits and distal neural circuits, and the excitabilities of the corticospinal tract in human subjects. Recently, it is also used as a method to modify neural activities in the central nervous system through the use of transcranial magnetic stimulation or transcranial direct current stimulation. Therefore, these methods are effective in detecting or inducing plastic change in neural activities, which is related to “software.”
To establish a multidisciplinary approach to SCI, it would be interesting to combine those approaches, so that the basic knowledge of molecular biology can be applied directly to a clinical setting. However, there have not been many models that have been used successfully for this purpose probably because of the unavailability of a proper animal spinal cord injury model for observing plastic change in neural activity in an electrophysiological manner. Therefore, at this point, it would be better to utilize either of the two methodologies depending on the clinical problem that needs to be solved.
8.2 Approaches for Treating Complete Spinal Cord Injury
Patients with SCI show varied symptoms and levels of severity. With regard to severity, there is (1) complete SCI, i.e., complete loss of sensory and motor functions below the lesion, and there is also (2) incomplete SCI, i.e., preservation of sensory and motor functions. In the case of complete SCI, especially in patients with severe dislocation of the spinal column, the connection between the brain and lower circuits seems to be completely lost. In such cases, it is important to restore some connection beyond the lesion before considering reprogramming the reorganized neural circuits. For this purpose, the molecular approach has an advantage over the electrophysiological approach.
As mentioned above, molecular biology has revealed much about the mechanisms governing axonal elongation. Especially, the intracellular mechanisms that are triggered by nerve growth factor stimuli have been investigated in detail. Nerve growth factors (NGFs), brain-derived neurotrophic factor (BDNF), and neurotrophin-3 (NT-3) bind to their own receptors expressed on the cell surface of neurons and transduce their effects via phosphorylation cascades of signaling molecules within the cell, which finally induce gene expression for axonal elongation. Among several signaling cascades, activation of the Mek-Erk signals is said to be important, and it has been shown that Mek activation is sufficient to induce axonal growth in PC12 cell line—a model cell line for neurite growth—even in the absence of nerve growth factors. Miura et al. examined the application of this paradigm in a spinal cord injury model [2]. In their report, he transected rat spinal cord at the thoracic 10 level and injected an adenovirus gene transfer vector into the parenchyma of the proximal stump. This type of gene transfer delivered the gene to not only the segmental neural cells in the spinal cord but also the primary motor neurons in the brain, such as the red nucleus, by retrograde transport along the axons. After 6 weeks of spinal transection and simultaneous gene transfer of the control gene (LacZ) or the constitutively active Mek gene, the effect of gene transfer was examined by both behavioral and histological evaluation. Behavioral evaluation using the Basso, Beattie, and Bresnahan (BBB) scale [3], which has been a well-accepted hind limb motor scoring scale in a rat spinal cord injury model, showed better functional recovery in the active Mek-transferred group than in the control group (Fig. 8.2). Histological evaluation performed by injecting an anterograde neuronal tracer into the red nucleus showed marked regeneration of the rubrospinal tract beyond the complete transection site. Taken together, activation of intracellular signals within the primary motor neuron in the brain can be one of the approaches to retain functional recovery in cases in which the axonal connection is completely lost at the lesion site.
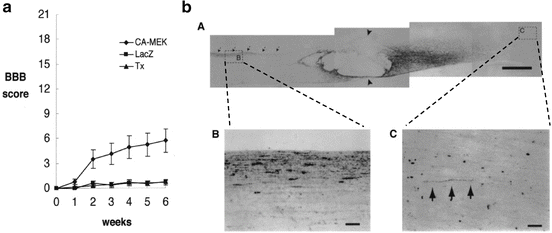
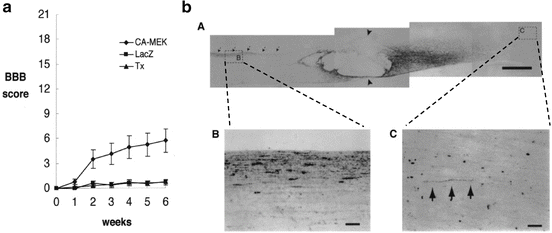
Fig. 8.2
A molecular approach for treating complete SCI. For patients with complete SCI, we have shown the restoration of axonal connectivity between the supraspinal circuit and the area below the lesion. (a) Adenoviral vectors carrying either CA-MEK or mock were applied into transected spinal cord. After 2 weeks functional recovery was observed in the CA-MEK transferred group, in which the intracellular MEK-ERK signaling cascade is constitutively activated in primary motor neurons of the brain. (b) Histological examination reveals axonal regeneration beyond the lesion (anterograde neurotracing)
8.3 Approaches for Treating Incomplete Spinal Cord Injury
The therapeutic strategy for patients with incomplete SCI should be different from that for those with complete SCI. Because the symptoms for this condition are varied and the degree of severity and the segment injured varies among patients with incomplete SCI, the patient’s condition needs to be thoroughly investigated, and the aspect of neural function that requires treatment should be determined. For example, the treatment approach for a patient who cannot stand even with support should differ from that for one who can walk with support but with a spastic gait. Here, we will discuss gait rehabilitation in patients with incomplete SCI, especially those who can stand with assistive tools and walk a few steps with assistance. These patients are classified as Frankel C and are considered to probably have SCI of “mild” severity. However, it is not practical for these patients to perform locomotion activity.
With regard to the modification of neural functions, physiological functions should be strengthened and abnormal functions corrected. Besides facilitation of voluntary movement of the lower limbs, automated movement of limbs is also considered an important physiological neural function in those patients. While walking, individuals do not have to pay attention to how to move their hip, knee, and ankle joints. It has been shown that a certain “gait program” exists within the central nervous systems and that we utilize this program. The existence of the gait program is shown using an experimental model of decerebrate cats [4–6] and also human subjects [7–9] on a treadmill; it revealed that the program is located in the spinal cord, and this program is now called the “central pattern generator (CPG).” We also examined the functions of CPG in patients with SCI under our experimental settings. By using the training device EasyStand Glider (Altimate Medical Inc., USA), we produced passive lower limb motion in an alternating manner and recorded electromyographic (EMG) signals in the lower limbs. Figure 8.3b shows the activities of each muscle that is completely paralyzed in the subject. Because we observed rhythmic burst from the right and left legs as observed when the limbs make stepping movements, we assumed that the CPG in this subject was activated. With the same device, we can also investigate which components of passive leg motion are critical for CPG activation. If the observed EMG activities are induced by stretch reflex in each leg, the same EMG activities are expected when only one leg is passively moved or both legs are passively moved but in a synchronized manner and not in an alternating manner. Even though the kinematic parameters of the examined leg were exactly the same under the experimental conditions, we only observed a gait-like EMG pattern in those patients who showed passive leg motion in an alternating manner (Fig. 8.3c). These results indicate that CPG activation is specific to afferent stimuli resulting from alternate leg motion [10].
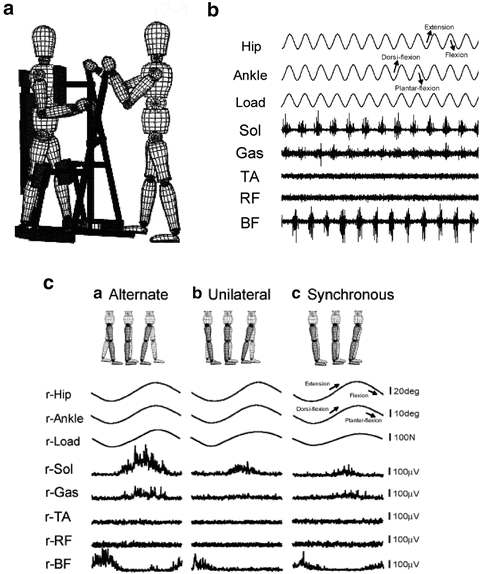
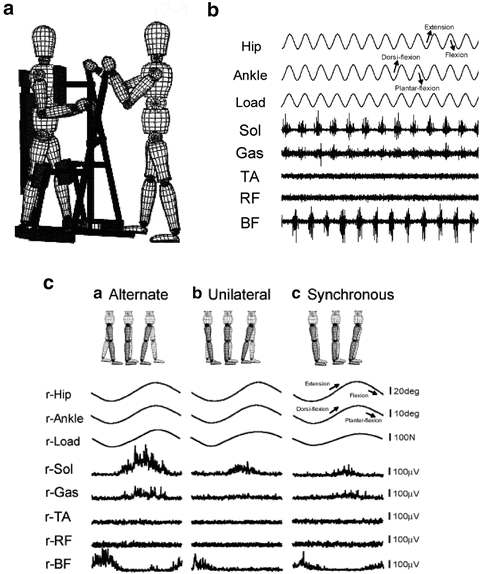
Fig. 8.3
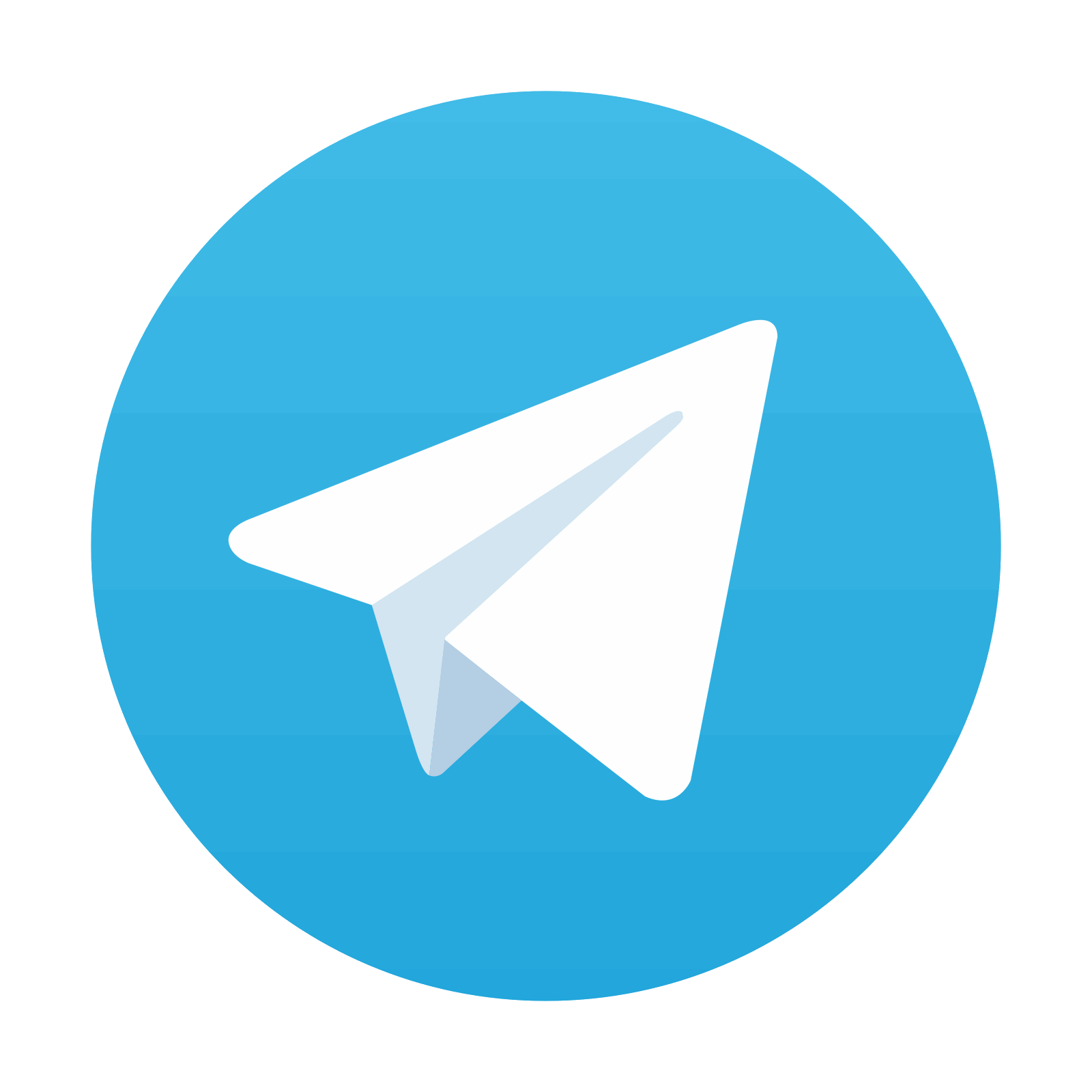
An electrophysiological approach to incomplete SCI. For patients with incomplete SCI, we have shown that the activation of the central pattern generator is one of the key elements for the restoration of locomotive function. (a) Passive leg exercise using the EasyStand Glider. (b) Phasic EMG activities in the soleus (Sol), gastrocnemius (Gas), and biceps femoris (BF) muscles during passive leg exercise in patients with complete SCI. (c) Alternate leg motion is necessary for the induction of gait-like EMG activities. Even though kinematic parameters are equivalent, EMG activities evoked during alternate passive leg motion are much stronger than those evoked during unilateral or synchronous leg motion
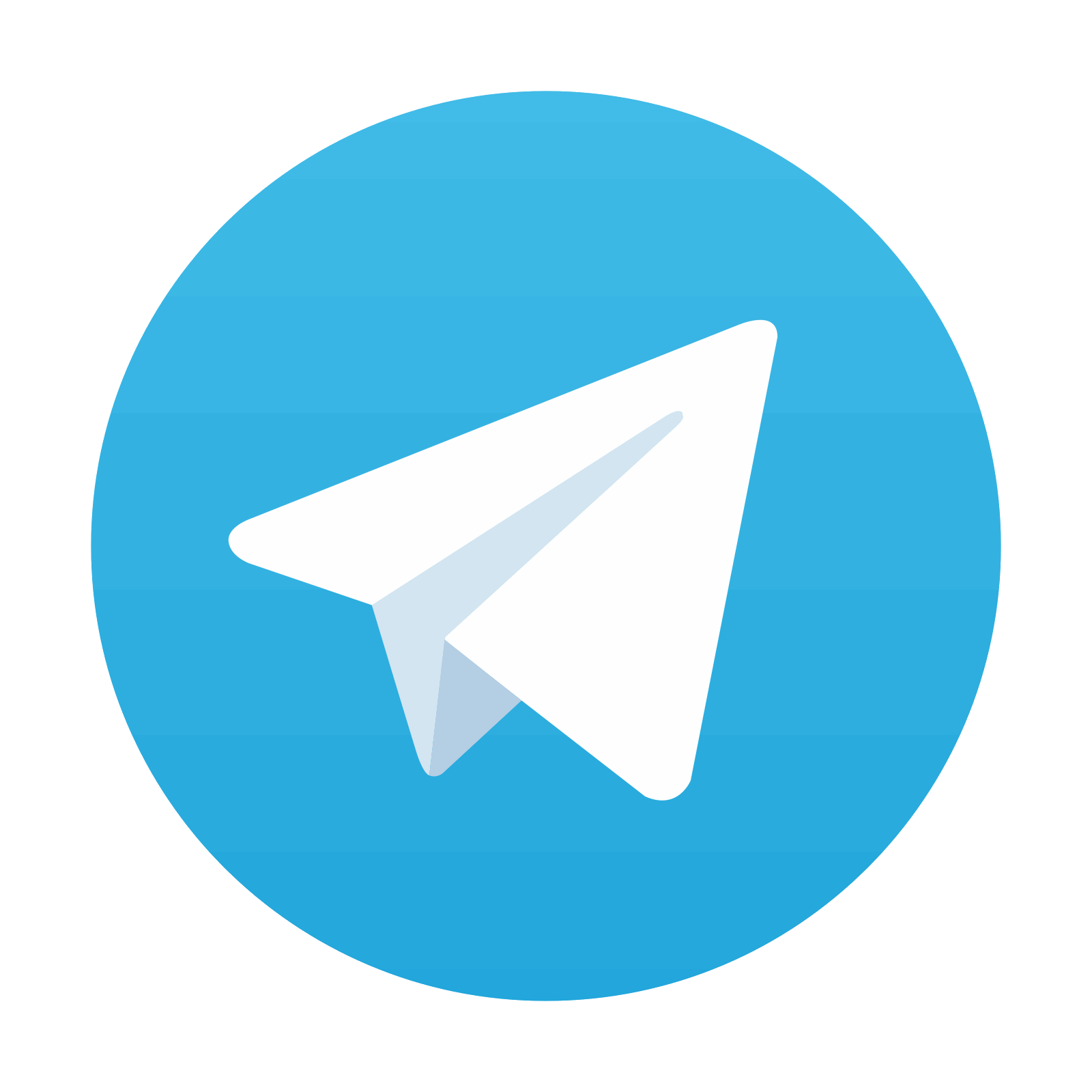
Stay updated, free articles. Join our Telegram channel

Full access? Get Clinical Tree
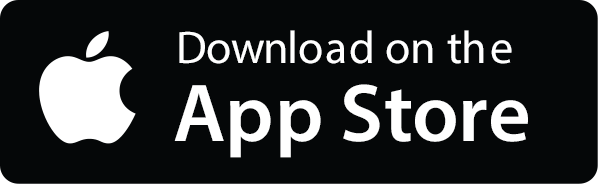
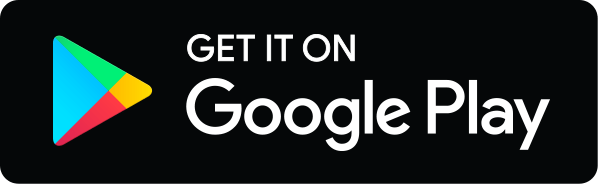
