Traditionally, status epilepticus (SE) was defined as continuous or repetitive seizure activity persisting for at least 30 minutes without recovery of consciousness between attacks. More recently, authors have suggested that seizures exceeding 5 to 10 minutes should be considered SE.1,2 For all practical purposes, a patient should be considered to be in SE if a seizure persists for >5 minutes, as very few single seizures last this long.3
This chapter will focus on patients with status epilepticus that does not respond to initial therapy, known as refractory status epilepticus (RSE). RSE can be defined loosely as failure of any of the standard regimens for SE.4 The exact definition of RSE is controversial. Major points of controversy are the number of antiepileptic drugs (AEDs) required to have failed (usually two or three) with or without a requirement for a certain amount of time to have passed. We use the following definition, as do many others: RSE is convulsive or nonconvulsive SE that continues clinically or electrographically despite first- and second-line therapy,4 with no minimum amount of time required.
Patients with RSE primarily have clinically subtle electrographic or nonconvulsive seizures. Another term for nonconvulsive status epilepticus (NCSE) is subtle status epilepticus, defined as consisting of “nystagmus, eye blinking, and/or mild motor movements with marked impairment of consciousness and continuous or nearly continuous, usually bilateral, ictal patterns on the EEG [electroencephalogram].”5 The same authors later described a continuum of SE from convulsive to subtle to only electrographic,6 a common scenario in RSE. Although subtle status epilepticus has been applied broadly to any SE with subtle clinical manifestations, the 2006 International League Against Epilepsy proposed classification uses the term for the more specific scenario described by Treiman,5,6 in which convulsive SE is followed by periodic epileptiform discharges with subtle or no clinical manifestations.7,8 We have suggested the following term (after Latin consultation) and definition for this phenomenon.8
Status epilepticus terminans: A condition after an overt convulsive seizure in which the patient is comatose for at least 30 minutes and has periodic epileptiform discharges on EEG and subtle or no clinical correlate other than coma. The preceding convulsive seizure can be of any duration, and the periodic discharges can be lateralized or generalized.
Prior to the widespread use of EEG monitoring, the incidence of RSE was estimated at 2000 to 6000 cases per year in the United States.9 Exact incidence and prevalence numbers are difficult to obtain in the absence of a population-based study conducting continuous EEG monitoring for all patients with status epilepticus. The results of the Veterans Affairs (VA) Cooperative study,10 a randomized trial that compared four different first-line interventions for generalized convulsive status epilepticus (GCSE), indicated that RSE may be a problem of greater magnitude than was generally appreciated. In this trial, 38% of patients with “overt” SE and 82% of patients with “subtle” SE had continued seizures after receiving full doses of two AEDs. In a study using EEG monitoring after clinically controlled GCSE, 48% were found to have electrographic seizures, and 14% were found to have NCSE.11 Others confirmed these data and reported that, depending on sampling bias and local practices of implementing EEG monitoring after GCSE, 9 to 48% of patients had continued seizures after initial therapy.12,13
RSE can occur at any age. One study found that patients with RSE were often younger than those with SE responsive to initial therapy (38.7 ± 13 vs 55.4 ± 18.2 years; p = .03),14 but others did not find this association.13
Patients with underlying epilepsy who develop SE often have seizures that are more easily controlled than those of patients without underlying epilepsy. However, prevalence rates are not available. Also, in the existing small case series, epilepsy causing status epilepticus did not remain an independent predictor of refractoriness (RSE in 23% of those with and 19% of those without underlying epilepsy, p = .185).13
Outcome in RSE is poor with most studies. Typical mortality rates are ~50%,15–18 and only a third of patients return to their premorbid functional baseline.13,16 In a systematic review of the literature comparing different treatment strategies for RSE, mortality was ~50% irrespective of the chosen AED.19 Poor outcome is associated with old age, underlying etiology with acute symptomatic causes having the worst prognosis, long SE duration, high Acute Physiology and Chronic Health Evaluation 2 (APACHE 2) scale, and nonconvulsive seizures with or without periodic discharges at the onset.13,14 EEG documentation of NCSE,11 ictal discharges,20 and periodic lateralized epileptiform discharges,20 have been associated with poor outcome and mortality after GCSE. Survival is substantially better in patients with epilepsy and in children, although most children with RSE subsequently have severe chronic epilepsy.21,22
Morbidity after SE may result from neurologic deficits directly caused by the seizures (discussed below under Pathologic Substrate), acute brain disease (e.g., the stroke or tumor causing SE), or secondary medical complications and prolonged hospitalization. Acute systemic effects of GCSE include hyperglycemia, lactic acidosis, massive catecholamine release, cardiac arrhythmia, hyperpyrexia, increased central venous pressure, and increased cardiac output.23,24 Later consequences of prolonged status may include cardiorespiratory failure, hypoglycemia, hyponatremia, pulmonary edema, aspiration, cardiac arrhythmia, tachycardia, hypotension, hyperpyrexia, lactic and respiratory acidosis, rhabdomyolysis, shoulder dislocation, and rib fractures.23,24 Myocardial infarction may occur, as can contraction band necrosis from a hypersympathetic state.25 RSE is associated with a number of serious medical complications, even after controlling for hospitalization duration, including fever, pneumonia, hypotension, bacteremia, and anemia treated with blood transfusions.13,16
Few studies have investigated predictors of refractoriness.13,14,19,26 The likelihood of developing RSE increases if SE is not diagnosed and treated early. There are animal and human data to suggest that therapeutic interventions are most effective when initiated early and that efficacy decreases significantly with increasing seizure duration.26,27 Studies have demonstrated that seizures are effectively controlled in 80% of patients if treatment is started within 30 minutes of onset of SE but in only 40% if treatment is started after 2 hours of seizure activity.26
Nonstructural causes for SE, such as hypoxia-ischemia, metabolic encephalopathy, and central nervous system (CNS) infection, are more refractory to therapy.26 Localization is highly variable, but a focal process is probably involved in most cases of subtle SE, even when the EEG appears to be generalized. Preliminary perfusion computed tomography (CT) evidence suggests that regions of hyperperfused cortex can be identified during subtle SE but are not seen in other postictal settings.28
NCSE and focal motor seizures at onset have also been identified as independent risk factors for refractoriness in SE;13 secondary generalized seizures tend to be particularly resistant to treatment.29 Among patients with CNS encephalitis, 9% had RSE.30 As mentioned previously, some authors found SE more likely to be refractory to initial therapy in younger patients.14 Among children, a family history of seizures, a higher seizure frequency prior to onset of SE, a higher number of maintenance AEDs, nonconvulsive SE, and focal or electrographic seizures on initial EEG were found to be associated with RSE by univariate analysis.22
NCSE and periodic EEG patterns are independently associated with poor outcome for patients with acute ischemic stroke, aneurysmal subarachnoid hemorrhage, CNS infections, traumatic brain injury, and nontraumatic intracerebral hemorrhage (ICH) after controlling for other predictors of poor outcome.31–33 It is not completely clear if seizures themselves cause harm or if they are surrogate markers for the severity of brain injury. There are some clinical data that support the notion that these EEG patterns may cause additional harm. Nonconvulsive seizures are associated with increasing mass effect and midline shift in patients with nontraumatic ICH.34 CSE triples mortality in patients with ischemia stroke independent of stroke size and location.31 Duration of convulsive and nonconvulsive seizures independently predicts outcome.10,35 NCSE without acute brain injury is rare but may cause CNS injury by itself.36 Likewise, elevations of biomarkers of neuronal injury, such as neuron-specific enolase (NSE) from serum and cerebrospinal fluid (CSF), and via cerebral microdialysis (elevated glutamate glycerol and lactate–pyruvate ratio) suggest that seizures may cause neuronal damage.37,38 NSE levels are highest with acute brain injury together with seizures, and seizures alone may cause an elevation.39,40 One theory is that seizures in an acutely injured brain, which is marginally maintaining homeostasis, lead to excessive metabolic demand, increased blood flow, elevated glutamate, and other adverse processes that compromise at-risk brain tissue, leading to additional brain injury with cell death. The impact of these biochemical processes on neuronal health may be exacerbated by systemic effects that are often seen in patients with SE, including hypoxia, fever, hyper- and hypoglycemia, and hypotension. Importantly, there has not been a study to date that has attempted to determine if treating nonconvulsive seizures, periodic epileptiform discharges (PEDs), or NCSE affects outcome.
The underlying mechanism for refractoriness is currently incompletely understood but includes AED target alteration through ongoing seizures, reduced sensitivity of gamma-aminobutyric acid type A (GABA-A) receptors to AEDs (particularly the GABA-ergic AEDs, including barbiturates and benzodiazepines), reduced surface expression of GABA-A receptors (e.g., trafficking), upregulation of N-methyl-d-aspartate (NMDA) receptors, and possibly overexpression of drug efflux transporters.27,41–43
As mentioned, prolonged convulsive SE typically progresses to nonconvulsive or subtle SE if seizures continue. Clinically, these patients appear comatose and may show eye deviation; subtle myoclonic, intermittent, focal, or multifocal movements; and nystagmoid eye jerks.10,44 Others have reported hippus in patients with nonconvulsive seizure activity on EEG.45
Animal studies of untreated SE have identified five discrete electrographic stages of continuous seizure activity: discrete electrographic seizures, waxing and waning seizures, continuous ictal discharges, ictal discharges with flat periods, and PEDs.46 In humans undergoing EEG monitoring, it is unclear if this sequence occurs, as treatment is usually given, altering the natural progression, but all of these stages have been documented in patients in SE (Figure 28-1). As patients go through these stages, seizures become increasingly refractory to treatment (Figure 28-2). Some patients clearly present with CSE that has stopped, but now they are comatose and have PEDs on EEG; this is the condition of status epilepticus terminans (SET), as mentioned above (also referred to as subtle SE by some) (Figure 28-3). SET typically presents with bilateral clinical and EEG findings despite focal epileptogenic foci and does not usually occur in idiopathic generalized epilepsy.47
Figure 28-1.
Five stages of status epilepticus in humans. Stage 1: Discrete seizures with interictal slowing. Stage 2: Merging of discrete seizures with continuous discharges but waxing and waning of frequency and amplitude. Stage 3: Continuous ictal discharges. Stage 4: Continuous ictal discharges with intermittent flat periods. Stage 5: Periodic epileptiform discharges on a flat background. (From Treiman DM, Walton NY, Kendrick C. A progressive sequence of electroencephalographic changes during generalized convulsive status epilepticus. Epilepsy Res. 1990;5:49–60. Reprinted with permission.)

Figure 28-2.
Response to treatment of status epilepticus stratified by initial electroencephalographic (EEG) findings. (From Treiman DM, MP; VA Status Epilepticus Cooperative Study Group 265. Utility of the EEG pattern as a predictor of success in the treatment of generalized convulsive status epilepticus. Epilepsia.1991;32:18. Reprinted with permission.)

Figure 28-3.
(A) EEG of a young woman with encephalitis and convulsive status epilepticus. After convulsive seizures subsided, the patient was comatose with occasional lip twitching. (B) EEG showed generalized periodic epileptiform discharges on a flat background. (From Hirsch LJ. Subtle status epilepticus. In: MedLink Neurology. San Diego, CA: MedLink; 2008. Reprinted with permission.)

EEG monitoring has demonstrated subclinical electrographic seizure activity in up to 48% of patients after control of convulsive SE (see Figures 28-4 and 28-5; Video 28-1).11 Breakthrough seizures and withdrawal seizures are frequent during treatment of refractory SE (56% and 68%, respectively, in one series of midazolam use for RSE).16 These seizures are typically subclinical. Additionally, EEG findings in the aftermath of SE include a range of PEDs that do not meet formal seizure criteria.44,48 These PEDs are characterized by a spike, sharp waves, or sharply contoured slow waves that occur every 0.5 to 2.0 seconds, and may be lateralized (PLEDs; Figure 28-6), generalized (GPEDs; Figure 28-3), or bilateral but independent (BIPLEDs; Figure 28-7). These patterns are not limited to patients following SE but may be seen in a number of acute brain injuries. There is controversy regarding the interpretation and therapeutic implications of these EEG findings, with some experts arguing that these represent postictal patterns, and others reporting some evidence suggesting that these may be ictal patterns. PLEDs may be associated with a reversible confusional state in the elderly,49 a local increase in glucose metabolism demonstrated on positron emission tomography (PET) similar to patterns seen during seizures,50 increased regional blood flow in the area of the PLEDs that disappeared with the resolution of the PLEDs,51,52 and individual discharges during PEDs that may be time-locked to focal movements in patients with focal motor SE.53 These clinical and imaging data supporting the notion that PLEDs at times may be ictal stand in contrast to reports of benign clinical courses with long-standing chronic PLEDs.54,55 Many authors (including us) believe these patterns are usually on an ictal-interictal continuum56,57 in which there is no dichotomy between “ictal” and “interictal” (see Other Treatment Decisions).
Figure 28-5.
A 2-year-old with epilepsy believed to be postictal after a cluster of seizures treated with lorazepam, seemingly successfully. Three pages of consecutive EEG showing high-amplitude, well-developed electrographic seizure from the right hemisphere with no clinical correlate (see Video 28-1). These recurred every few minutes, all without detectable clinical correlate.

Quantitative EEG can be quite useful for following monitoring trends and rapidly interpreting large quantities of EEG (Figure 28-8).3,58 However, it is crucial to look at the underlying raw EEG.
Figure 28-8.
A 63-year-old woman presented with convulsive seizures in the setting of fever with nonconvulsive SE after lorazepam and fosphenytoin. (A) EEG monitoring showed frequent nonconvulsive seizures that were easily quantified using compressed spectral array analysis of the raw EEG. (B) Corresponding raw EEG reveals nonconvulsive seizure at the time marked with a vertical blue line in A.

Modern neuroimaging tools have furthered the understanding of SE, but their role in the clinical management beyond determining the cause (e.g., diagnosing a brain tumor or stroke) is currently limited. Most imaging changes due to SE are transient, but prolonged uncontrolled SE may lead to structural changes, including hippocampal sclerosis57 and laminar necrosis59 (Figure 28-9). Other imaging abnormalities in prolonged seizures are focal signal hyperintensities on fluid-attenuated inversion recovery (FLAIR) magnetic resonance imaging (MRI) sequences.60 Diffusion-weighted imaging (DWI) using apparent diffusion coefficient (ADC) maps demonstrates restricted diffusion in the hippocampus, thalamus, and affected cortex possibly related to associated cytotoxic edema (Figure 28-10).61,62 The radiologic characteristics of SE may resemble those of ischemic stroke (on MRI, cortical hyperintensity on DWI and T2; on CT, decreased attenuation, effacement of sulci, and loss of gray-white differentiation), but they can be differentiated based on the nonvascular distribution, increased flow on magnetic resonance angiography, and leptomeningeal enhancement on postcontrast MRI.63 Interestingly, in animal models, the degree of maximum decrease of ADC is directly related to the degree of neuronal cell loss due to prolonged seizure activity.64 Proton magnetic resonance spectroscopy (MRS) reveals elevated lactate, decreased N-acetylaspartate, and elevated choline60,65–68 and may provide a method to determine the cellular injury secondary to SE. Ictal single-photon emission computed tomography (SPECT), magnetic resonance perfusion, BOLD (blood oxygen level dependent) functional MRI, and arterial spin labeling may reveal perfusion changes during different stages of SE, but research in this area is preliminary and ongoing.69–71
Figure 28-9.
(A) Ictal 99m hexamethylpropyleneamine oxime (HMPAO) single-photon emission computed tomography (SPECT) demonstrating right temporoparietooccipital hyperperfusion. (B1–2) Axial T2-weighted images showing cortical increased signal intensity of the right temporoparietooccipital regions compatible with transient radiologic changes associated with SE. B1 corresponds to the first SE. Notice the extensive cortical and white matter edema associated with the brain abscess compared with the exclusively cortical edema related to radiologic changes associated with SE. The patient recovered after the first SE without significant neurologic deficits. B2 corresponds to the second SE. At this time, the edema was mostly cortical, and there were no signs of acute infection in the temporal region. (B3–4) Follow-up magnetic resonance imaging (MRI), 3 weeks after the second SE. Axial and sagittal T1-weighted images show a high-intensity cortical laminar lesion following a gyral distribution associated with volume loss in the right posterior temporal, parietal, and occipital regions, indicative of cortical laminar necrosis. Notice also a hyperintense lesion on T1-weighted images located at the posterolateral portion of the ipsilateral thalamus (C2). (C1–2) Axial T1/T2-weighted images showing cortical increased signal intensity on T2-weighted images (C1) of the left parietal region and decreased signal intensity in the exact same location in T1-weighted images (C2) during the first SE. Notice the absence of hyperintense cortical lesions in the posterior quadrant during the first SE in C2. (C3–4) Follow-up MRI, 1 week after the second SE. Axial and sagittal T1-weighted images show a high-intensity cortical lesion following a gyral pattern associated with volume loss in the left posterior temporal, parietal, and occipital regions. (From Donaire A, Carreno M, Gomez B, et al. Cortical laminar necrosis related to prolonged focal status epilepticus. J Neurol Neurosurg Psychiatry. 2006;77:104–106. Reprinted with permission.)

Figure 28-10.
Diffusion-weighted MRI demonstrating restricted diffusion patterns in patients with status epilepticus in the thalamic (upper row), hippocampal (lower row), and cortical regions (upper and lower rows). (From Szabo K, Poepel A, Pohlmann-Eden B, et al. Diffusion-weighted and perfusion MRI demonstrates parenchymal changes in complex partial status epilepticus. Brain.2005;128:1369–1376. Reprinted with permission.)

Some attempts have been made to use imaging techniques to help determine if PEDs following SE represent an ictal or postictal pattern. These studies found increased blood flow on SPECT51 and increased metabolism on 18F-fluorodeoxyglucose (FDG)–PET50 to argue that PEDs following SE may be ictal. To us, the terminology used is of minimal importance.
The real questions are the following: (1) Is this EEG pattern indicative of ongoing neuronal injury, and (2) Is this EEG pattern what is keeping the patient from waking up? (Figure 28-11).56 We are hopeful that we can answer these questions, at least in individual patients, using advanced multimodality cerebral monitoring (including microdialysis and brain tissue oxygen monitoring), biomarkers (including NSE), and imaging sequences (including MRS and arterial spin labeling), and tailor treatment to that individual based on those findings. We suspect that the EEG pattern alone will not be adequate for determining whether neuronal injury is occurring, and that individualized, physiology-driven decision making will be required.
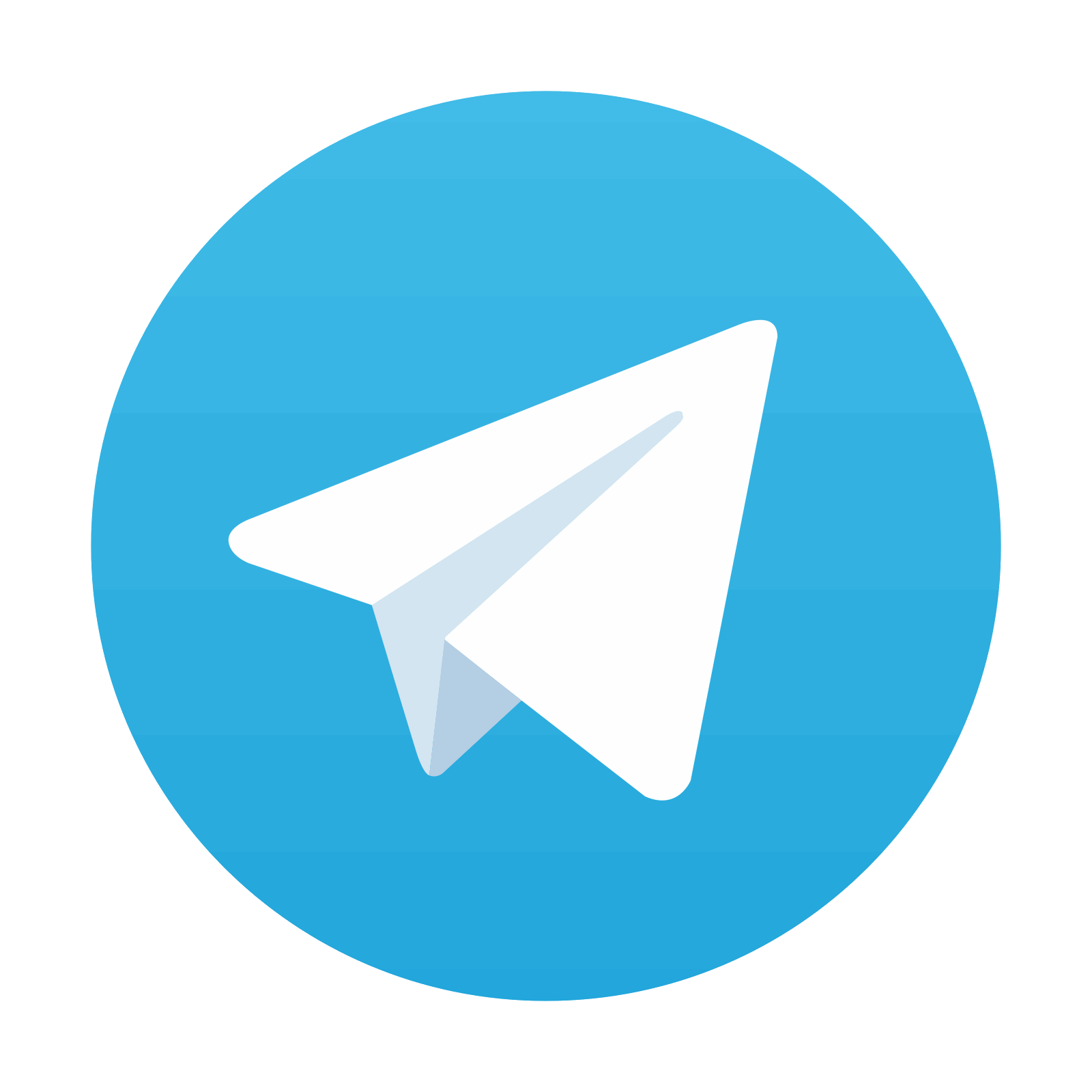
Stay updated, free articles. Join our Telegram channel

Full access? Get Clinical Tree
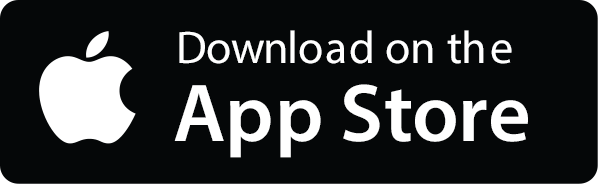
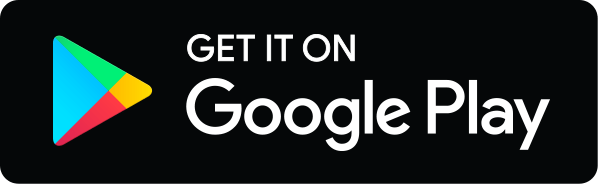