© Springer International Publishing Switzerland 2016
José L. Pons, Rafael Raya and José González (eds.)Emerging Therapies in Neurorehabilitation IIBiosystems & Biorobotics1010.1007/978-3-319-24901-8_12Virtual Rehabilitation
Manuel Bayón-Calatayud1 , Elisabetta Peri2 , Francisco Fernández Nistal3 , Margaret Duff4 , Francisco Nieto-Escámez5 , Belinda Lange6 and Sebastian Koenig6
(1)
Complejo Hospitalario de Toledo, Toledo, Spain
(2)
Politecnico di Milano, NeuroEngineering and Medical Robotics Laboratory, Milan, Italy
(3)
Adacen—Asociacion de Daño cerebral de Navarra Mutilva (Navarra), Mutilva Baja (Navarra), Spain
(4)
Rehabilitation Institute of Chicago, Chicago, IL, USA
(5)
Department of Psychology, Universidad de Almeria, Almeria, Spain
(6)
Katana Simulations UG (haftungsbeschraenkt), Regensburg, Germany
Abstract
This chapter addresses the current state of the art of virtual rehabilitation by summarizing recent research results that focus on the assessment and remediation of motor impairments using virtual rehabilitation technology. Moreover, strengths and weaknesses of the virtual rehabilitation approach and its technical and clinical implications will be discussed. This overview is an update and extension of a previous virtual rehabilitation chapter with a similar focus. Despite tremendous advancements in virtual reality hardware in the past few years, clinical evidence for the efficacy of virtual rehabilitation methods is still sparse. All recent meta-analyses agree that the potential of virtual reality systems for motor rehabilitation in stroke and traumatic brain injury populations is evident, but that larger clinical trials are needed that address the contribution of individual aspects of virtual rehabilitation systems on different patient populations in acute and chronic stages of neurorehabilitation.
Keywords
Virtual realityGame-based rehabilitationNeurorehabilitation Virtual rehabilitation1 Introduction
The world population is expected to grow to 9.68 billion people by 2050 [25]. More importantly, the proportion of people aged 60 or older is expected to increase in the so-called “more developed countries” from currently 23 to 32 % of the total population by 2050 [32]. With neurological disorders such as stroke, Parkinson’s disease, or multiple sclerosis being more prevalent in older adults [1, 8, 30], our healthcare systems desperately need cost-efficient, widely available interventions that can address the cognitive and motor impairments of the aforementioned disorders and help reintegrate affected individuals in society.
Virtual rehabilitation technologies and interactive off-the-shelf games have seen increasing popularity in clinical settings over the past two decades [10, 14]. Virtual rehabilitation includes a wide array of applications that use virtual scenarios and environments for the purpose of clinical assessment and remediation. Available systems range from complex motion platforms, projection systems, tracking systems, and head-mounted displays to low-cost gaming consoles and motion-tracking sensors. Distinguishing factors of such systems are the use of fully virtual environments (i.e., virtual reality), real environments with an overlay of virtual information (i.e., augmented reality), or a mixture of both (i.e., mixed reality). Further, virtual rehabilitation setups can be immersive or non-immersive where immersive denotes systems in which three-dimensional scenarios are displayed and the user can change visual perspective through head movements (e.g., head-mounted displays or cave projection setups). On the contrary, non-immersive systems present virtual scenarios on a two-dimensional display (e.g., on a TV, laptop, or computer screen) with or without interface devices such as keyboard, computer mouse, or a joystick. Regardless of the actual hardware and software configuration, these approaches are often considered viable alternative or adjunct treatments to existing therapies, because of their motivational nature and precise control over feedback and task parameters.
This chapter focuses on the use of virtual rehabilitation systems for the treatment of motor deficits after stroke and traumatic brain injury. Recent advances of virtual reality hardware are being discussed and their potential for new rehabilitation systems along with strengths and weakness are being outlined. Moreover, relevant clinical trials and meta-analyses since the writing of the previous version of this chapter are summarized and their results discussed.
2 Advances in Virtual Reality Technology
Virtual reality systems are often a heterogeneous set of input/output devices that can consist of a wide range of displays, tracking devices, controllers, and computer systems. Historically, such systems were almost exclusively associated with high costs, cumbersome, wired equipment, and a lack of compatibility between different devices and device drivers [27]. However, within the past two years virtual reality technology has seen a transformation toward low-cost components that are being developed and marketed for the rapidly growing video and computer game industry. Much of that growth is also driven by the rise of mobile computing and the availability of high-resolution mobile displays. Modern head-mounted displays have evolved from cumbersome, tethered devices to goggles that simply allow the user to attach an off-the-shelf smartphone and leverage the inbuilt motion sensors and cameras of the phone. Consequently, prices for head-mounted displays have dropped from tens of thousands to merely hundreds of dollars while image quality and tracking latency have improved tremendously. Examples of such new display solutions are the Oculus Rift,1 Samsung Gear VR,2 Avegant Glyph,3 Durovis Dive,4 Google Cardboard,5, or the Zeiss VR One.6 Most of these are still in alpha or beta prototype stage, but new innovative products are announced on a regular basis and should invigorate the competition further. A similar trend is evident for video game peripherals and 3D interaction devices which are mostly developed for console and computer game markets. New tracking devices such as the Microsoft Kinect 1 and 2,7 Leap Motion controller,8 Razer Hydra,9 Nimble Sense10, and treadmills such as the Virtuix Omni11 and Cyberith Virtualizer12 provide an affordable entry to naturalistic 3D interaction in virtual scenarios. All of these new competitors are of high importance to developers and researchers in the virtual rehabilitation field, as for the first time in the history of virtual reality technology, therapeutic systems have become affordable and accessible for use in the wider clinical setting, outside of the research laboratory. In addition, the development of virtual rehabilitation software has become much more accessible as game engines such as Unity,13 Unreal Engine 414, and CryEngine 315 are widely available for low monthly subscriptions or even for free. Each development tool comes with large communities of enthusiastic game developers who provide free assets, tutorials, and help on online forums and discussion groups. Virtual reality hardware companies have realized the potential of these large communities and provide free integrations of their devices and drivers for the most common game engines. All of these factors taken together provide an excellent ecosystem for the development of low-cost virtual rehabilitation systems that would have cost hundreds of thousands of dollars three to five years ago.
3 Advantages of Virtual Rehabilitation
Innovative technologies such as virtual rehabilitation tools are being applied by using motor learning principles and taking advantage of neuroplasticity in order to compensate, restore, and recover loss of sensorimotor function occurring in stroke and traumatic brain injury patients. This section will outline the different factors that make virtual rehabilitation systems a suitable option for the treatment of sensorimotor deficits in neurorehabilitation and add value beyond traditional forms of treatment.
(a)
Comprehensive data collection
Data is at the core of most other aspects of virtual rehabilitation systems mentioned in this section. Comprehensive collection of performance data enables the patient and therapist to track rehabilitation progress and adjust training parameters for optimal recovery. Performance data is also necessary to track the efficacy of each rehabilitation system and can help the clinician decide which intervention is best used for different patient populations or individual patients. Data collection can encompass usage patterns, task completions, task difficulty adaptations, and task outcomes on a macro level and reaction times, responses to task stimuli, movement quality, and logging of feedback or distractions on a micro (behavioral) level. Furthermore, each variable can be tracked and summarized over longer timespans across multiple sessions or even across patients and patient populations. This allows researchers and clinicians to track the efficacy of combinations of tasks and feedback for patients with different deficits, lesion locations, and demographics. Moreover, summary data of task usage gives deeper insights into the success and habits of clinicians and how their experience or background influence therapy outcomes with virtual rehabilitation tools.
Large datasets can be collected when using sensors and motion tracking systems. Oftentimes, datasets can be too complex for unprocessed use by clinicians and must be condensed before they can aid clinicians in their decision-making process. Compared to clinical observation using rating scales, automated data collection of virtual rehabilitation systems can capture many high-resolution variables simultaneously. Exemplarily, a tracking system can capture movement of all tracked joints at millisecond and millimeter accuracy and combine this information with the system’s presentation of task stimuli, distractions, and the user’s responses and errors. Manually observing the same scenario and assessing all variables in a reliable and valid manner is simply impossible. Additionally, automating data collection frees the therapist’s resources and allows for unhindered interaction between patient and therapist.
(b)
Multisensory feedback
Feedback is an integral part to rehabilitation exercises as it allows patients to monitor their performance, promote errorless learning, and avoid compensatory movements. Virtual rehabilitation systems often have a multitude of opportunities for feedback delivery. Most systems include components for visual and auditory presentation of information which can be utilized for feedback delivery. Even tactile input through the use of pressure sensors, electrotactile stimuli, or puffs of air are potential feedback mechanisms.
Feedback can target individual performance parameters such as movement speed, trajectory, precision, and smoothness as well as more holistic parameters such as task completion or completion time. Feedback delivery can occur in real-time or as a summary after the movement or even the training session have been completed. With the proper use of feedback, the patient’s attention can either be focused on individual task parameters or the movement as a whole, depending on the goal of the training session. Real-time feedback requires additional attentional resources and has to be used carefully in order to not distract from the actual task. The choice of feedback modality and presentation can facilitate the processing of feedback without too much task interference. For example, visual feedback can lend itself to outline an optimal movement trajectory while auditory feedback can indicate information about movement speed [16]. Moreover, feedback modalities should be adapted to accommodate the strengths and weaknesses of different patient populations. Exemplarily, patients with deficits in visual attention might benefit more from auditory feedback and aphasic patients might benefit most from non-written visual and non-spoken auditory feedback.
Lastly and most importantly, all aforementioned feedback mechanisms can be applied dynamically and adaptively when a system’s collected data is being utilized. Choice of feedback modality, frequency, and task parameters to give feedback on should be adjustable to the unique situation of each patient. Either the therapist, patient, or the virtual rehabilitation system itself should be able to change feedback parameters throughout the course of a patient’s rehabilitation. As the patient’s performance increases, different feedback mechanisms, frequencies, or increasingly implicit feedback might become more relevant for an optimal recovery.
(c)
Precise control over scenarios
Developers of virtual rehabilitation scenarios usually have full control over all aspects of the simulation. That is, events, distractions, animations, task stimuli, and feedback can be precisely controlled to guarantee a consistent experience for each patient. Ideally, most of these parameters are then exposed in the application’s interface to give the therapist control over the content of each training session. Alternatively, the aforementioned data collection capabilities allow the simulation to tweak task parameters automatically based on the user’s performance and therapeutic goals. Exemplarily, error reduction or error augmentation can be adjusted dynamically to balance motivational aspects and therapeutic success over the course of therapy sessions. However, control over virtual scenarios extends much beyond the configuration of task parameters. Displaying environments and avatar movement are two key components that can have a large impact on the training scenario. Environments can range from game-like or abstract environments to more realistic simulations to suit the patient’s preferences and enhance motivation. In fact, simulating environments that are otherwise inaccessible or too dangerous for patients is one of the key advantages of virtual rehabilitation tools. For example, patients can safely practice reaching for targets in a virtual supermarket which would otherwise be an inaccessible location for patients undergoing inpatient rehabilitation. The representation of the patient’s movement on screen can heavily influence task performance. Moreover, it allows patients to assess their own movement and actively learn how their body movement is connected to the visual feedback they receive on the screen. The patient and his/her movements can be represented realistically or in a more metaphorical way in order to increase or take away focus from affected limbs and relevant movements. Characters, if displayed at all, can be realistic avatars, neutral mannequins, cartoon characters, or even real-time camera images of the patient (e.g., Microsoft Kinect or Playstation 2 Eyetoy16).
In sum, each and every aspect of a virtual scenario must be carefully considered by the developers and therapists and in best case should be highly flexible to adapt to each patient’s unique circumstances.
(d)
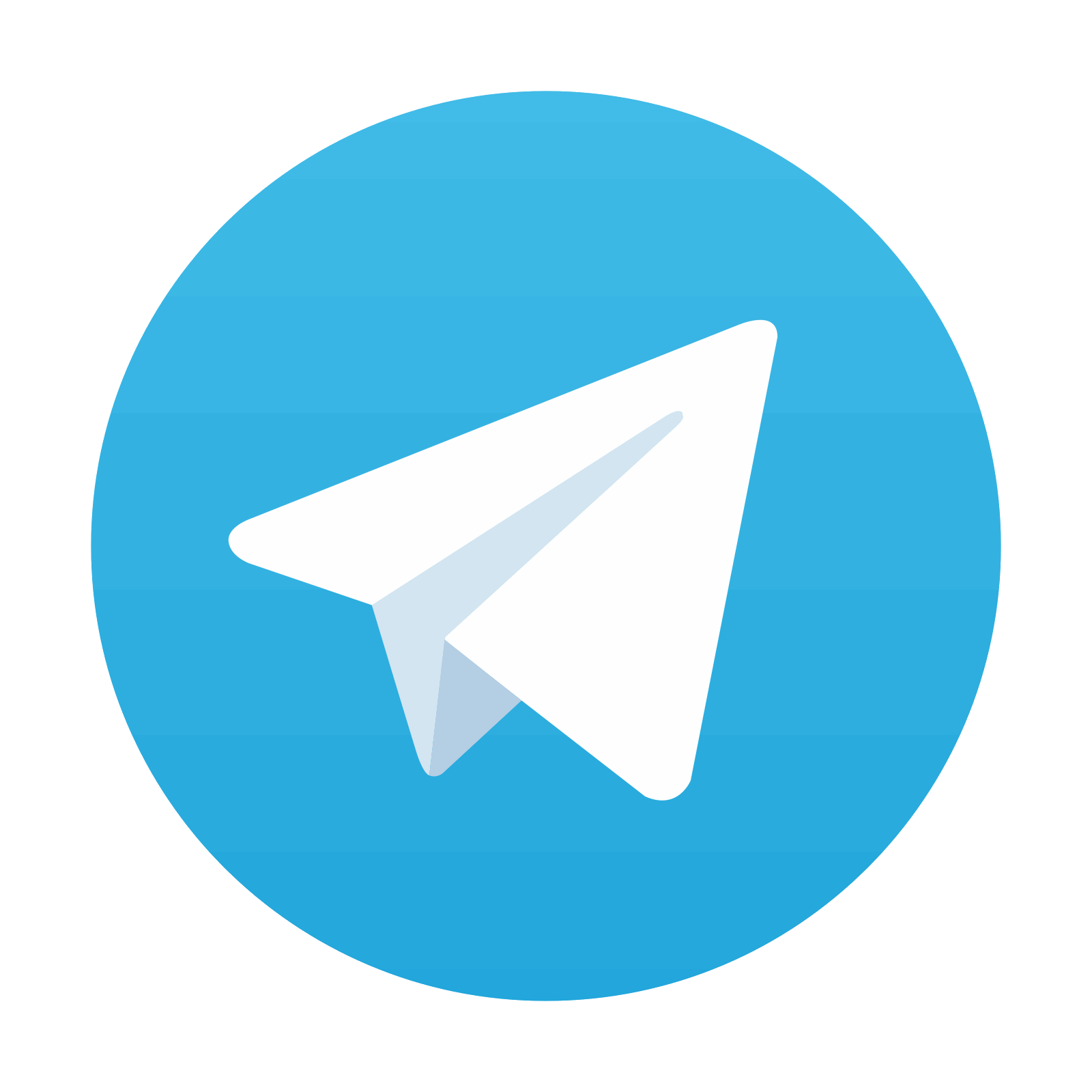
Enhanced motivation
Adherence to therapy programs is one of the most critical aspects of neurorehabilitation as high-frequency repetition of movements has been shown to be key for recovery [3, 18, 19]. Unfortunately, current therapy practices are not able to encourage patients to perform the number of repetitions required for neuroplastic changes to occur [12]. Furthermore, within the home setting, patients often lose their motivation over time as tasks become repetitive and feedback about progress is lacking or non-obvious. Virtual rehabilitation scenarios have become a popular choice for therapy, largely for their ability to motivate patients to continue their exercise regimes over extended periods of time. Game-based mechanics and features such as high scores, achievements, virtual reward items, diverse landscapes, and interesting characters are excellent ways to engage patients during their rehabilitation and clearly communicate progress over time. The implementation of game mechanics also takes away the focus from affected limbs and shifts attention to achievable goals within the game environment, thus potentially reducing anxiety. Repeating similar tasks in different game-like contexts even promotes generalization of learned behaviors which has been shown to be important for transfer to activities of daily life.
However, not each virtual rehabilitation tool needs to look and feel like a computer game. Enjoyment of game-like content or even graphics style often depend on personal preference and graphical fidelity. In some cases, a realistic simulation of a relevant real-life environment can be the most motivating scenario as long as it aligns with the patient’s goals. Yet, even simple task rewards or a scoring system can go a long way to motivate the patient over extended periods of time and provide an easy-to-understand feedback system.
Increased patient motivation continues to be one of the most powerful aspects of virtual rehabilitation systems. Virtual scenarios and tasks need to be designed with a variety of content to engage patients beyond the novelty effect of the first few training sessions. After all, repetitive task practice is still thought to be the most effective means to regain motor function after neurological injuries, despite the frequent lack of patient motivation.
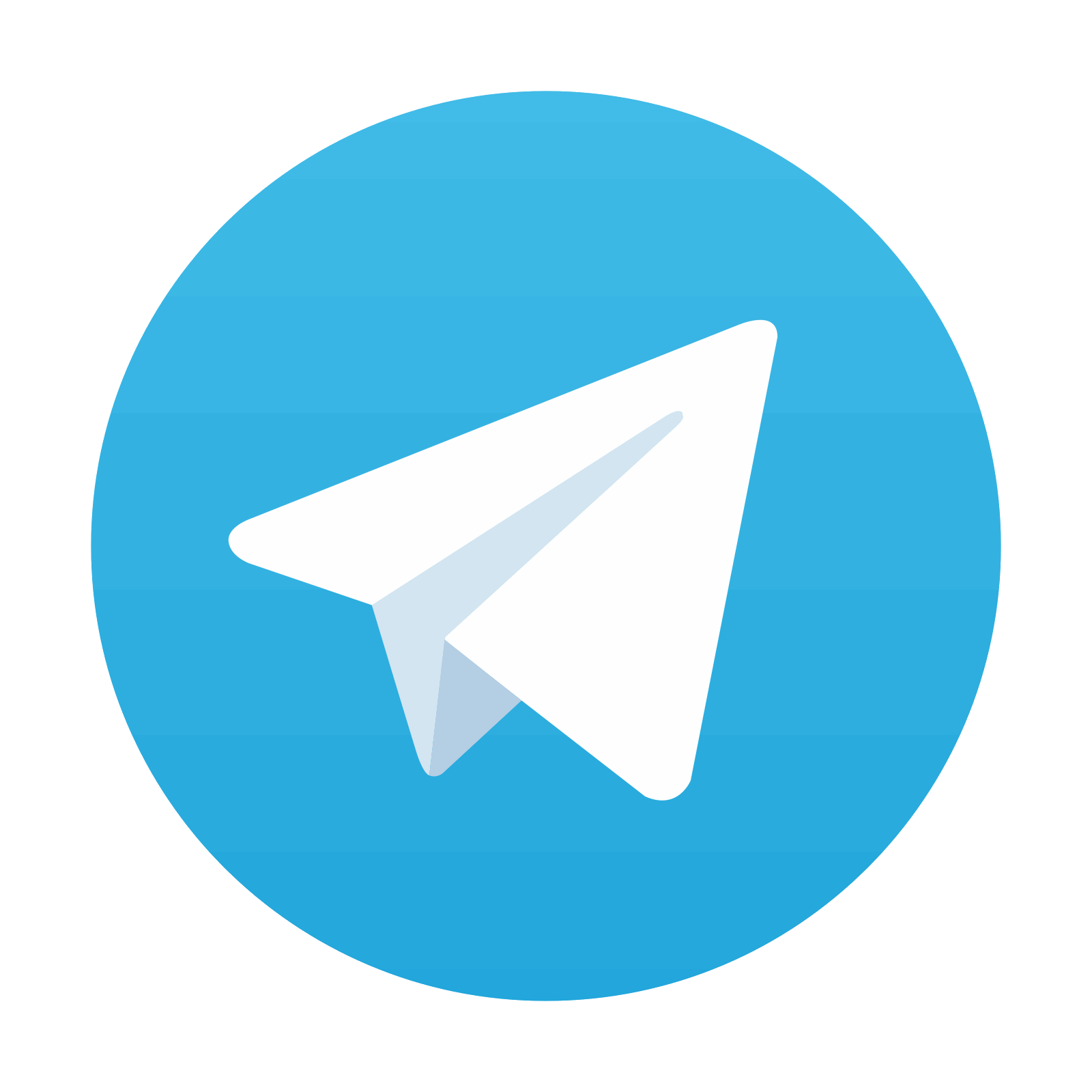
Stay updated, free articles. Join our Telegram channel

Full access? Get Clinical Tree
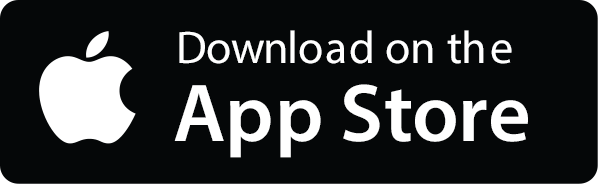
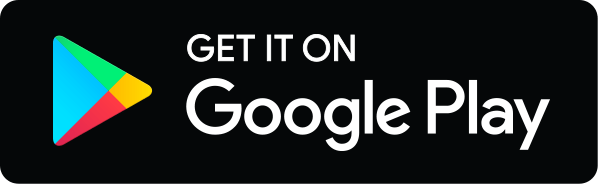
