A 26-year-old woman was admitted following a horse-riding accident. She had fractured her femur and suffered an intracranial hemorrhage. Postoperatively she required mechanical ventilation and then developed a respiratory tract infection. On the 3rd hospital day, her urine output had fallen to 0.5 mL/kg/h, and her serum creatinine had risen from 0.5 mg/dL on admission to 1.24 mg/dL. At this stage her intracranial pressure (ICP), measured with an intraventricular catheter, remained elevated at 35 mm Hg, with a mean arterial blood pressure of 90 mm Hg. In view of the increase in creatinine and decrease in urine output, a fluid challenge was given to try and prevent progression of acute kidney injury (AKI), to exclude a volume-responsive reversible cause of AKI (Table 48-1). However, by the following day her urine output had fallen to 0.3 mL/kg/h, and the creatinine level had increased to 2.0 mg/dL.
Prerenal (volume responsive) | Intrinsic | Postrenal |
---|---|---|
Hypovolaemia | Glomerular | Obstruction |
Vomiting and diarrhea Hemorrhage Decrease in effective circulating volume Cardiac failure Septic shock Cirrhosis Burns Drugs ACE inhibitors NSAIDs | Glomerulonephritis Glomerular endothelium Vasculitis Malignant hypertension Scleroderma Tubular Acute tubular necrosis Rhabdomyolysis Myeloma Drug toxicity Radiocontrast Interstitial Interstitial nephritis Drug reactions | Renal calculi Retroperitoneal fibrosis Prostatic hypertrophy Carcinoma Cervical carcinoma Urethral stricture Bladder neoplasm Pelvic neoplasm Retroperitoneal neoplasm |
As kidney function declines, the products of cellular metabolism accumulate, leading to retention of both the nitrogenous products of metabolism, typically assessed by the measurement of urea and creatinine, and also potassium accumulation and the development of a metabolic acidosis. Other potential toxins also accumulate,1 leading to the term azotemia rather than uremia. This patient has acute kidney injury (AKI); as such, the time course of the illness is short, and only a limited amount of toxins will have accumulated. However, patients with AKI may have to be started on renal replacement therapy (RRT) because of hyperkalemia refractory to standard medical therapy or increasing pulmonary edema compromising oxygenation, and brain hypoxia (Table 48-2).
Biochemical indications |
Refractory hyperkalemia > 6.5 mEq/L Serum urea > 80 mg/dL Refractory metabolic acidosis pH ≤ 7.1 Refractory electrolyte abnormalities: hyponatremia or hypernatremia and hypercalcemia Tumor lysis syndrome with hyperuricemia and hyperphosphatemia Urea cycle defects, and organic acidurias resulting in hyperammonemia, methylmalonic acidemia |
Clinical indications |
Urine output < 0.3 mL/kg for 24 h or absolute anuria for 12 h AKI with multiple organ failure Refractory volume overload End-organ damage: pericarditis, encephalopathy, neuropathy, myopathy, uremic bleeding Create intravascular space for plasma and other blood product infusions and nutrition Severe poisoning or drug overdose Severe hypothermia or hyperthermia |
Historic data suggests that “early” initiation of RRT in AKI is associated with improved survival,2-6 but the evidence base is not sufficiently robust to allow a specific recommendation, and the decision to initiate RRT should remain a clinical one. Although the decision to initiate RRT is straightforward in those patients with refractory hyperkalemia, severe metabolic acidosis and volume overload, and/or overt azotemic symptoms, in the absence of these overt manifestations, there is debate as to the optimal time to initiate renal support (Table 48-2). Early introduction of RRT as soon as a patient enters AKI stage 3 may be of benefit, so that the patient is not exposed to the potentially deleterious effects of metabolic abnormalities and/or volume overload. However, early initiation of RRT in some patients will result in the adverse consequences of treatment, such as venous thrombosis and bacteremia secondary to vascular access catheters, hemorrhage from anticoagulants, and other treatment-related complications, in particular hypotension, which may exacerbate cerebral ischemic injury.
Initial reports, some dating back 50 years, suggested a clinical benefit of early initiation of RRT.2-7 These and other studies formed the basis for standard clinical practice that dialytic support should be instituted when the serum urea reached 80 mg/dL.2-7 However, not all studies have shown a survival advantage with early initiation.8
Although the current consensus from retrospective and observational studies suggests that “early” initiation of RRT in AKI may be associated with improved patient survival, this has not been proven.8 In patients with acute traumatic brain injury (TBI), RRT not only adds complexity to patient management, but may also cause additional complications, in terms of changes in ICP and mean arterial blood pressure.9 Thus, in cases of AKI developing in patients with TBI, early initiation of RRT should only be undertaken in cases of refractory hyperkalemia or pulmonary edema, because in the majority of cases AKI is secondary to a prerenal insult, which may be volume responsive, and recover, therefore avoiding the necessity of RRT. However, additional toxic insults, such as aminoglycoside antibiotics and nonsteroidal anti-inflammatory drugs (NSAIDs), should either be avoided, or minimized, and every effort should be made to reduce the risk of radiocontrast-induced AKI,10 including minimizing the number of radiocontrast studies.11
If kidney function continues to deteriorate, then azotemic toxins accumulate over days, leading to increased intracellular osomolality. If plasma osmolality is rapidly decreased during dialysis, then the osmolality gradient between plasma and the brain may lead to movement of water into the brain, and so potentially increase brain edema.
Although there are no randomized clinical trials, it is probably advisable to initiate RRT before the serum urea nitrogen exceeds 40 to 50 mg/dL in cases of AKI, in order to limit the amount of cerebral edema caused by the fall in serum urea during RRT.12
When should renal replacement therapy be instituted in patients previously established on dialysis?
Patients with chronic kidney disease, established on regular dialysis, are susceptible to brain injury. These patients are at increased risk of subdural hemorrhage due to the prescription of coumarin anticoagulants, intracerebral hemorrhage secondary to hypertension, and cerebral abscesses secondary to central venous dialysis access catheter–associated infections. These patients have chronically high levels of urea and other azotemic toxins, and, therefore, delaying dialysis treatments will lead to even higher concentration of these solutes, thereby increasing the risk of RRT-induced cerebral edema. So for this group of patients, RRT should be considered once the patient has been stabilized.13
The key strategy of any RRT is to remove small, water-soluble solutes. Urea clearance from the plasma water is faster during hemodialysis (HD) treatments than urea movement from cells into the plasma water, because urea moves out of cells through urea transporters. In contrast, water moves rapidly across cell membranes through aquaporin water channels some 20 times faster than the corresponding transcellular passage of urea through urea transporters.14 This differential removal rate sets up a urea concentration gradient across the cell membrane, with water then moving rapidly back into the brain cells down the osmotic gradient (Figure 48-1). Renal replacement therapy, particularly intermittent HD, can therefore lead to cerebral edema, not only in acute experimental animal models of HD,15 but also in the outpatient setting of dialysing healthy patients with end-stage kidney failure.16 This swelling of the brain associated with HD is termed the dialysis disequilibrium syndrome.
Although RRT is primarily designed to remove small, water-soluble toxins that accumulate in kidney failure, it is also used to correct the metabolic acidosis associated with kidney failure, and therefore dialysis solutions contain either bicarbonate or lactate as buffers, in supraphysiological concentrations. The rapid increase in plasma bicarbonate during dialysis leads to increased generation of carbon dioxide (H+ + HCO3– ↔ H2CO3 ↔ H2O + CO2). The bicarbonate, being charged, does not readily cross lipid-rich cell membranes, but the acidifying carbon dioxide readily diffuses into cells (Figure 48-2). Thus, rather than correcting intracellular acidosis, the increased bicarbonate may paradoxically worsen intracellular acidosis.17 Intracellular acidosis increases cell swelling and will exacerbate any underlying cerebral edema. These changes in plasma bicarbonate compared with CSF bicarbonate typically lead to changes in the respiratory center in the medulla, with alteration in breathing patterns in healthy outpatient dialysis subjects.18 Thus too rapid a correction of plasma bicarbonate, in nonventilated brain-injured patients, may adversely affect cerebral oxygenation.
In addition the connection of a patient to an extracorporeal circuit can lead to hypotension, which can potentially compromise cerebral perfusion. The passage of blood across the dialyzer, particularly those with a very negative surface charge (ζ-potential), can lead to the generation of potent vasodilators: bradykinin, nitric oxide, and the anaphylotoxins, C3a and C5a.19 It is now recognized that both cardiac and cerebral perfusion can decrease within the first few minutes of initiating standard outpatient hemodialysis.20,21 This effect on cardiac perfusion may be exacerbated in cases of subarachnoid hemorrhage or severe cerebral edema, due to the associated neurogenic cardiac stunning. Bradykinin generation and complement activation by the extracorporeal circuit can be reduced by rinsing the circuit with isotonic bicarbonate rather than saline, and some centers advocate priming the dialyzer with albumin to coat the membrane to reduce membrane reactions. However, priming the circuit with blood, particularly aged blood, may exacerbate any reactions due to the acidic nature of stored blood.22 Angiotensin-converting enzyme (ACE) inhibitors prevent bradykinin degradation and thus should be avoided.
Several different modes of RRT are potentially available.
Peritoneal dialysis (PD) is a form of continuous RRT and, as such, causes a more gradual fall in serum urea and osmolality during treatment than intermittent HD.23 Even so, the dialysis disequilibrium syndrome has still been reported during PD.24 Peritoneal dialysate fluids are hyponatric, with a sodium concentration of 132 mEq/L, but are hypertonic because of high glucose content, varying from 13.6 to 38.6 g/dL, and so may cause patients to become hyponatremic. Large intraperitoneal volume exchanges of hypertonic glucose solutions can adversely impact cerebral perfusion and cerebral perfusion pressure by reducing right atrial filling and cardiac output.25 To minimize these potential changes, PD prescriptions should use the lowest glucose concentrations possible to avoid major swings in intraperitoneal volumes. This can best be achieved by using PD cycler machines with a tidal exchange program.
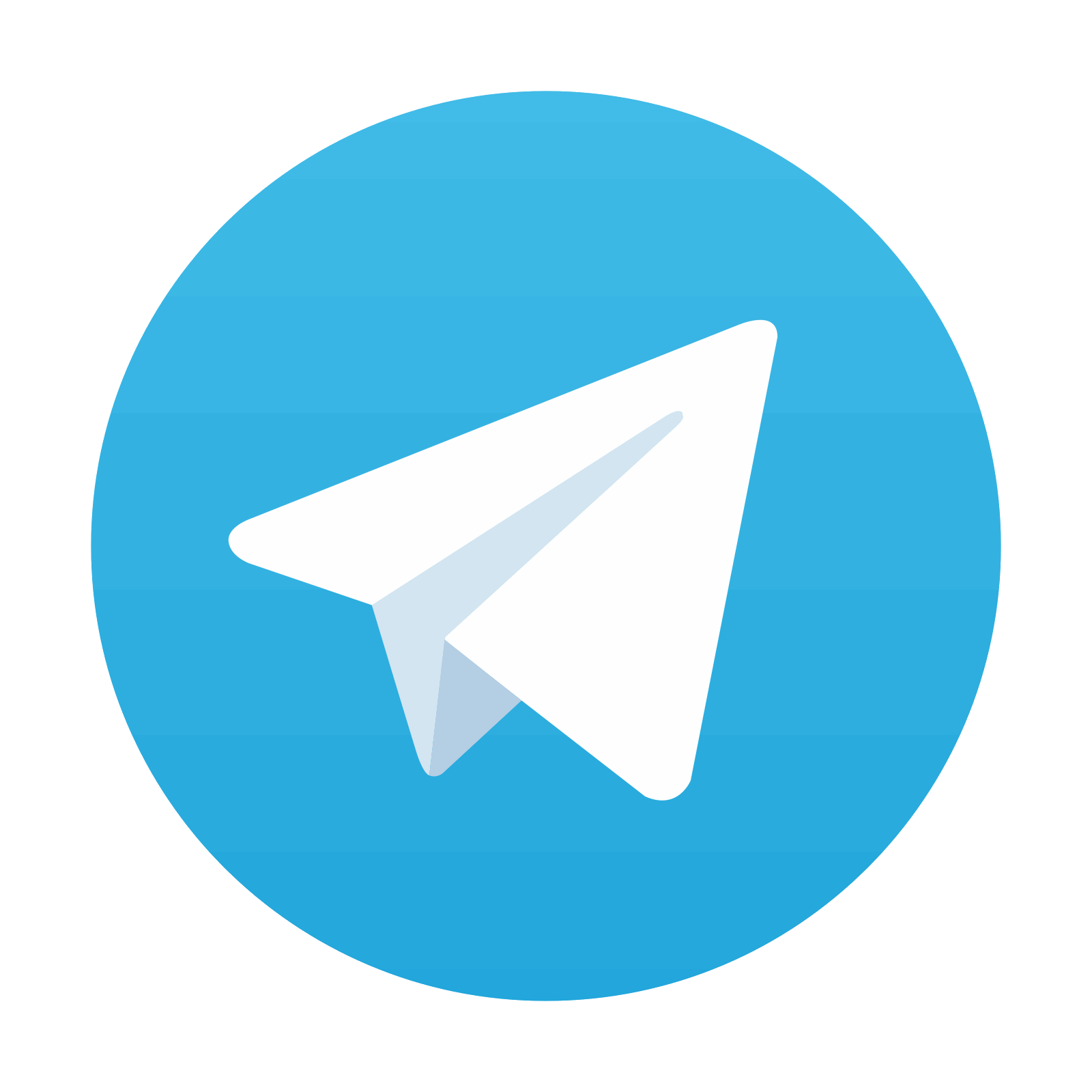
Stay updated, free articles. Join our Telegram channel

Full access? Get Clinical Tree
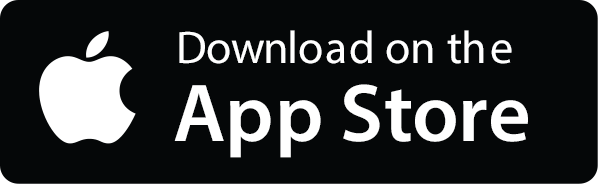
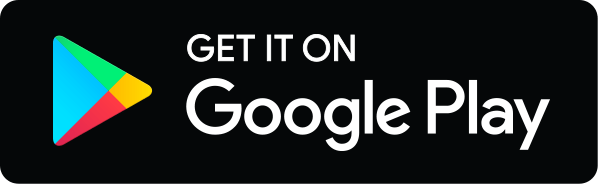